This chapter emphasizes four specific areas that are often the focus of injury prevention protocols: the hamstrings, spine, anterior cruciate ligament, and ankle.
Hamstring Strains
- Roald Bahr
Muscle injuries occur frequently and manifest as contusion injuries in contact sports and as strains in sports that involve maximal sprints and acceleration. Among sprinters, hamstring strains represent approximately one third of all acute injuries. Because the different football codes (i.e., for soccer, rugby, American football, and Australian Rules football) combine maximal sprints with frequent player-to-player contact, it is not surprising that a sizable proportion of injuries are thigh injuries. In fact, recent studies from the professional level show that hamstring strains alone rank as the first or second most common injury in soccer, Australian Rules football, rugby, and American football, and in most studies they account for one in every five to six injuries. Unlike data from studies conducted in the 1980s, current data appear to show a trend toward a gradual increase in the proportion of hamstring strains compared with other injury types, such as ankle sprains. However, it should be noted that quadriceps strains are also common in soccer and that muscle contusion injuries to the quadriceps muscles account for a significant proportion of all football injuries at the elite level. Hamstring strain injuries are also common in sports where the muscles may be stretched past the usual range of movement, such as in dancing and water skiing.
Causes
Injury Mechanisms
Two main mechanisms are involved in thigh muscle injuries: direct (contusion) and indirect (distention or strain) mechanisms. The contusion mechanism is straightforward: The player is typically injured by a direct blow from an opponent, usually with the knee hitting the lateral thigh in a tackle (also known as a “charley horse” or “cork thigh”). The muscle is crushed between the opponent’s patella and his own femur ( Fig. 41-1 ).

The injury mechanism for hamstring strains is less well understood. The hamstring muscle group is composed of three muscles—semimembranosus, semitendinosus, and biceps femoris. All of them (except the short head of the biceps) have their origin at the ischial tubercle on the pelvis, and they insert at the inside and outside of the lower leg right below the knee, which means that they overlap two joints—they extend the hip joint and flex the knee joint. Muscle strains usually occur at the myotendinous junction, but avulsion injuries from the ischial tubercle are also seen.
Hamstring strains occur most often during maximal sprints. It is difficult to document exactly at what time injuries occur during the running cycle. However, because the net moment developed by the hamstrings is thought to be maximal in the late swing phase, right before heel strike, this position is thought to be vulnerable. In this instance, the hamstring muscles work eccentrically.
Risk Factors
A number of risk factors have been proposed for hamstring strains, with the most prominent being the following four internal factors ( Box 41-1 ): age, previous injury, reduced hip range of motion (ROM), and poor hamstring strength. In theory, limited ROM for hip flexion could mean that muscle tension is at its maximum when the muscle is vulnerable, at close to maximum length. However, this hypothesis has yet to be confirmed, because several studies involving soccer players suggest that hamstring flexibility is not a risk factor for strains. However, other studies pertaining to soccer and Australian Rules football have shown that low quadriceps flexibility represents a risk factor not only for hamstrings, but also for quadriceps strains.
Well Documented
- •
Previous hamstring strain
- •
Increased age
- •
Low hamstring muscle strength
Less Well Documented
- •
Low hamstring muscle flexibility
- •
Low quadriceps muscle flexibility
- •
Race (black players and players of Aboriginal descent are at greater risk)
- •
Sex
- •
High level of play
- •
Insufficient warm-up
- •
Muscle fatigue
- •
Player position
Decreased hamstring strength would mean that the forces necessary to resist knee flexion and initiate hip extension during maximal sprints could surpass the tolerance of the muscle-tendon unit. Hamstring strength is often expressed relative to quadriceps strength as the hamstrings/quadriceps ratio, because it is the relationship between the ability of the quadriceps to generate speed and the capacity of the hamstrings to resist the resulting forces that is believed to be critical. Several studies show that players with decreased hamstrings/quadriceps ratios or side-to-side strength imbalances may be at increased risk of injury.
A history of previous hamstring strains greatly increases injury risk. Injury can cause scar tissue to form in the musculature, resulting in a less compliant area with increased risk of injury. A previous injury can also lead to reduced ROM or reduced strength, thereby indirectly affecting injury risk. Soccer players with a history of previous hamstring injury have a seven times higher risk of injury than healthy players; as many as 13% can expect to sustain a new injury during one season. Older players are at increased risk for hamstring strains, and although older players are more likely to have a previous injury, increased age is also an independent risk factor for injury.
Other risk factors that have been suggested but are less well studied include race, sex, level of play, player position, improper running technique, superior running speed (peak performance), low back pain, increases or changes in the training program (particularly during intense periods of training), insufficient warm-up, and muscle fatigue.
Methods for Preventing Hamstring Strains
Research on injury prevention methods for hamstring strains is limited, and the evidence available has mainly been collected from observational studies. None of the prevention methods described here has been tested in large-scale randomized clinical trials with hamstring strains as the main end point. Studies to date have examined intervention methods targeting the key risk factors for hamstring strains: hamstring strength, hamstring flexibility, and previous injury. In addition, one observational study of South African rugby players suggests that the use of thermal pants might reduce the risk of hamstring strain.
The consistent finding that a history of previous injury leads to a severalfold increase in the risk for new strains has led to the suggestion that this finding is at least partly due to inadequate rehabilitation and early return to sport. A study pertaining to Swedish soccer has documented that a coach-controlled rehabilitation program consisting of information about risk factors for reinjury, implementation of rehabilitation principles, and use of a 10-step progressive rehabilitation program, including return-to-play criteria, reduced the reinjury risk by 75% for lower limb injuries in general. Although the specific effect on hamstring strains could not be assessed in this study, it seems reasonable to recommend the use of functional and specific rehabilitation programs and careful screening of players before return to play.
No intervention studies on the preventive effect of flexibility training on hamstring strains in elite athletes have been performed. However, one study involving military basic trainees indicated a reduced number of lower limb overuse injuries after a period of hamstring stretching, whereas another military-based study found no effect of stretching. It should be noted that these studies were designed to examine the effect of general stretching on lower limb injuries in general, not the effect of a specific hamstring program on hamstring strain risk. Questionnaire-based data on flexibility training methods collected from 30 English professional football clubs, where the stretching practices of the teams were correlated to their hamstrings strain rates, indicate that using a standard stretching protocol reduces injury risk. Also, in one study from Australian Rules football, a reduction in the incidence of hamstring strains was observed with a three-component prevention program, with stretching while fatigued being one of the components. The other factors in the program were sport-specific training drills and high-intensity anaerobic interval training. Because the program included three components, it is not possible to determine which of these factors is/are responsible for the observed effect.
The best evidence for injury prevention is available for programs designed to increase hamstring strength, particularly eccentric hamstring strength. Several studies indicate that decreased hamstring strength is a risk factor for sustaining hamstring strains. Electromyographic studies have shown that activity is highest late in the swing phase and during heel strike, when the hamstrings work eccentrically or transfer from eccentric to concentric muscle action. It is assumed that most hamstring strains occur during eccentric muscle actions, when the muscle activity is highest. It is well documented that strength training is mode specific, and thus it may be argued that, to be specific, strength training for the hamstring muscles should be eccentric.
It has been suggested that an indicator of susceptibility for the damage from eccentric exercise is the optimum angle for torque. When the muscle is at a short muscle length, it is believed to be more prone to eccentric damage. By means of isokinetic dynamometry, it has been shown that mean optimum angle in previously injured muscles is at a shorter length than for uninjured muscles.
Recent studies from Scandinavia have shown that replacing the traditional hamstring strength exercise used by teams—that is, hamstring curls—with exercises to develop eccentric strength reduces the risk of hamstring strains. Traditional hamstring curls have been shown to be ineffective in increasing eccentric hamstring strength among elite athletes. In contrast, a simple partner exercise—the Nordic hamstring lower ( Fig. 41-2 )—has been demonstrated to be effective in improving eccentric strength. A pilot study has also shown that using a special apparatus—the YoYo flywheel ergometer—also increases eccentric hamstring strength. Both of these methods have been shown to prevent hamstring strains in studies with soccer players and rugby players. Because the Nordic hamstring lowers are easily implemented in a team setting, this exercise is recommended as a specific tool to prevent hamstring injuries. However, to avoid delayed-onset muscle soreness, it is important to follow the recommended exercise prescription with a gradual increase in training load when introducing a program of Nordic hamstring lowers (see Table 41-1 ). By the end of a 10-week training period, many players are able to stop the downward motion completely before touching the ground (i.e., at about 30 degrees of knee flexion), even after being pushed by his or her partner(s) at a considerable speed. When a player can reach this stage, the characteristics of the Nordic hamstring lower exercise appear to resemble the typical injury situation: eccentric muscle action, high forces, and nearly full knee extension. The program has been implemented in several different sports and in younger age groups; injuries from the exercise itself have not been recorded.

Week | Sessions per Week | Sets and Repetitions |
---|---|---|
1 | 1 | 2 × 5 |
2 | 2 | 2 × 6 |
3 | 3 | 3 × 6-8 |
4 | 3 | 3 × 8-10 |
5-10 | 3 | 3 sets, 12-10-8 repetitions |
Spinal Injuries
- Adam Shimer
All athletic activities demand a high degree of dynamic spinal stability and control. Spinal stability is the result of a complex interplay of bone, joints, ligaments, and muscles. The virtues of core strengthening and stability are intuitively attractive and routinely touted by both the lay press and the athletic training community. However, to date, strong evidence to support its effectiveness is sparse and often contradictory. Many popular exercise trends, including yoga, tai chi, and Pilates, have a strong focus on core stabilization techniques. Ideally, an exercise program focused on spinal stabilization would improve performance, treat pain, speed recovery from injury, and prevent further spine injuries.
History of Spinal Stability
The human spine is an inherently unstable structure, demonstrating in vitro collapse with axial loads as little as 90 N (about 20 lb). Panjabi first described spinal stability as the interaction of passive (bones/ligaments) and active (muscles) systems under neural control. He stated that clinical instability is the “loss of the spine’s ability to maintain its patterns of displacement under physiologic loads so there are no initial or additional neurologic deficits, no major deformity, and no incapacitating pain.” Perturbation of any one of these systems can place a greater demand on the remaining intact systems to maintain stability. Perhaps then if the associated muscles are weak or poorly coordinated, more stress is placed on the passive system, resulting in a greater chance of injury to the intervertebral disks, facets joints, and ligaments.
Many studies have focused on the role of a weak or dysfunctional core muscle as a cause of low back pain and injury. Hides et al. found a decreased ability to “draw in” the abdominal wall in Australian Football League players with back pain. In another study, Hides et al. demonstrated magnetic resonance imaging evidence of multifidus atrophy in subjects with chronic low back pain (LBP). Other investigators have used surface electrodes to show that persons with LBP exhibit significantly impaired trunk muscle coordination with increased superficial muscle activity and concomitant decreased recruitment of the deeper muscles during movements in the frontal, sagittal, and transverse planes.
An exercise regimen designed to decrease LBP and the risk of injury needs to identify which muscles are most important for conferring stability. Researchers have adopted two important models for spinal stability. The first model is that of a guy-wire system, such as the systems to stabilize large radio antennas or support small trees. In this model, antagonist or out-of-phase muscles must contract at the same time. This degree of this co-contraction is very small at rest because full contraction of the antagonist muscle group would result in high stress on the passive system (bone, intervertebral disks, and ligaments) and would render the spine stiff and inflexible. The other popular model is the contribution to the hydrostatic pressure within the closed abdomen that can be increased with transverse abdominis (TrA) and external oblique contraction. Unfortunately, spinal stability is a dynamic state whereby different positions, angular and linear acceleration, torque, and force demand differential contributions from all of the stabilizers. To date, no consensus has been reached on the optimal coactivation pattern for spinal stability.
Core-Strengthening Exercise Programs
A training regimen to optimize spinal dynamics and thereby sports performance, pain relief, and injury prevention would ideally address the components of stability. Most investigators agree, however, that the passive (osseoligamentous) system generally cannot be altered and therefore a “low back” training system will ideally include exercises for the active system (muscular control) and the neural feedback (truncal proprioception). This statement is made with the understanding that training and strengthening a single muscle or separating the active from the neural system is overly reductionist and that most exercises include aspects of all three systems in a complex interplay. Proper education is a key component to developing healthy spine habits. The education should promote proper posture, weight control, smoking cessation, and aerobic conditioning.
Training the Active System (Core Strengthening)
Although agreement has not been reached on the optimal muscle coactivation pattern for spinal stability, research has focused on the TrA, obliques, and multifidus. Rather than concluding that any one muscle is the key to spinal stability, it appears that all muscles work together in varying contributions depending on the continuously changing demands to provide stability. An exercise routine after a low back injury should balance the strengthening goal with placing the lowest loads on the spine.
Two different methods have been described for isolated core strengthening: abdominal bracing and abdominal hollowing. Abdominal bracing is conscious coactivation of the abdominal musculature and paraspinal musculature without decreasing or increasing abdominal circumference. Abdominal hollowing is described as drawing one’s umbilicus toward the spine. The abdominal hollowing maneuver, which coactivated the TrA and internal oblique, has been shown to correct abnormal electromyographic patterns. Vera-Garcia et al. compared recruitment patterns in truncal perturbation using hollowing and bracing maneuvers. They found that the bracing maneuver fostered torso co-contraction, reduced lumbar displacement, and increased trunk stability. Truncal proprioception may also play a role in core stability. Franco et al. studied a group of sprinters using a 6-week proprioceptive training program that included five exercises combining the BOSU balance trainer and Swiss ball. They found that the experimental group demonstrated improved performance on some eyes-open stability tests.
Outcomes
Proper use of best practice– and evidence-based care inherently requires a quantitative outcome measure with which one intervention, such as a core-strengthening regimen, can be measured against another. To date, no agreement has been reached on how to measure core strength. Interestingly, more evidence may exist for the importance of core-strengthening and core-proprioception training for prevention of lower extremity trauma than for prevention of low back injuries. Multiple studies have drawn a correlation between improved core control and decreased knee injuries, particularly in female athletes. Studies that have supported the superiority of core stabilization rather than a more traditional exercise program have significant design flaws, including lack of true control subjects, poor participant retention, and lack of statistical rigor. In two other randomized controlled trials, no significant difference was found between a core-strengthening program and standard exercise routines. Evidence is also scant that core-strengthening protocols improve athletic performance. Childs et al. showed an increased pass rate for military trainees in the Army Physical Fitness Test when a core-strengthening program is substituted for the traditional exercise program that uses sit-ups.
Conclusions
The importance of spinal stability is undeniable. The concepts of spine stability are discussed and used on a daily basis by spinal surgeons and other allied spine care practitioners. High-grade instability from trauma or degenerative conditions can result in neurologic injuries and often requires surgical stabilization. Most systematic reviews have concluded that core strengthening is beneficial but not statistically more beneficial than a traditional exercise routine of similar intensity and duration. More randomized, prospective studies are required to determine if core-strengthening programs improve the rate of recovery from low back injuries, prevent future injuries, and/or improve performance.
Anterior Cruciate Ligament Tear Prevention in the Female Athlete
- James Onate
Anterior Cruciate Ligament Injury Prevention Program
The number of anterior cruciate ligament (ACL) injury prevention programs has proliferated during the past two decades, with a seemingly endless number of research-investigated and clinically initiated programs currently being conducted. The essential component of most of these programs is a multivariate approach aimed at creating adaptations in movement technique, neuromuscular control, fatigue resistance, injury awareness, and mobility/stability concepts aimed at altering high-risk movement patterns. Most programs contain some type of injury risk awareness, strengthening of the lower extremity and trunk, enhancement of whole-body proprioception, increase in neuromuscular coordination, improvement of balance, and correction of potentially at-risk movement patterns through technique instruction and motor learning feedback systems in isolation or combination. Implementation of an ACL injury prevention program is ultimately the responsibility of the entire sports medicine team, including the player, coach, parent, athletic trainer, physician, strength and conditioning specialist, physical therapist, and other persons as necessary. The success of the program depends on an integration of roles for all persons involved and requires careful planning, execution, and review to evaluate the potential success of the program. Ideally, ACL prevention programs should be considered injury prevention plans that are modifiable as the situation demands because of the clinical considerations of individuals/teams, given that various components of each program need to be personalized (e.g., soccer specific vs. basketball specific). Careful planning and use of Finch’s model of translating research into injury prevention practice should also be considered the cornerstone in evaluating the effectiveness of any ACL injury prevention program.
Effective ACL injury prevention programs implement off-season, preseason, and in-season phases to enhance and maintain individual gains. Most of the promising programs with increased compliance have a similar schedule, including sport-specific warm-up two to four times per week during the off-season, training two or three times per week during the preseason, and training one time per week during in-season practice. The fundamental components of the most successful programs are dynamic warm-up, strengthening exercises, plyometrics, balance, sport-specific agilities, and instruction with feedback concerning proper movement techniques. An example of one program is the Federation International de Football Association Medical Assessment and Research Center (F-MARC) 11+ program ( Figs. 41-3 and 41-4 ). The F-MARC program requires minimal training and equipment (only a soccer ball required), making it an attractive alternative for persons with limited budgets and time. Three studies in which the F-MARC 11+ program was used with male and female adolescents demonstrated a reduction in injuries ranging between 21% and 71%. The specificity of the 11+ soccer-focused training program can be performed in different sports by emphasizing the key components of the program and making the sport movement tasks match the desired activity. Similar training programs have been reported to produce a 49% reduction in lower extremity injury risk and a 94% reduction in ACL injury risk in Norwegian handball players.

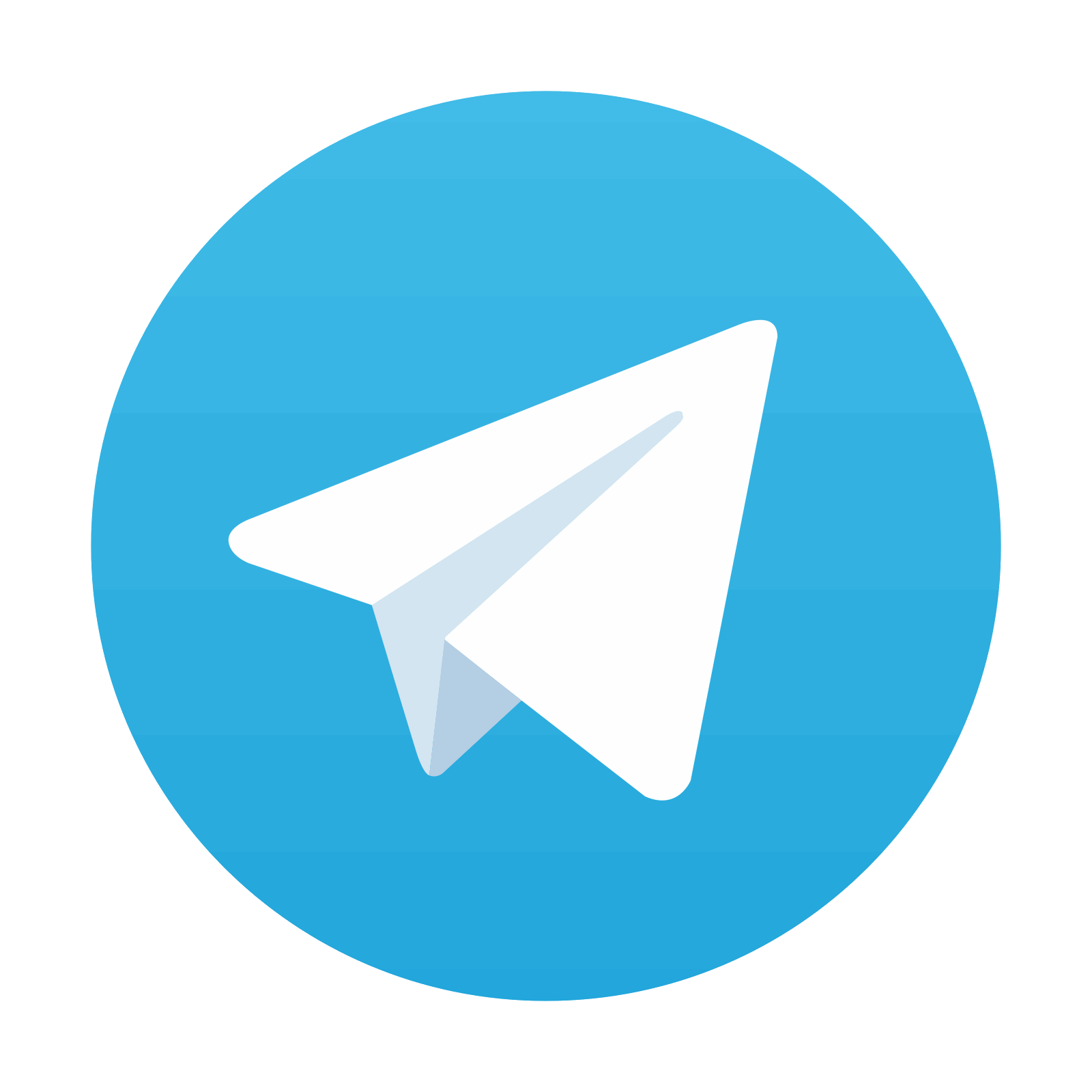
Stay updated, free articles. Join our Telegram channel

Full access? Get Clinical Tree
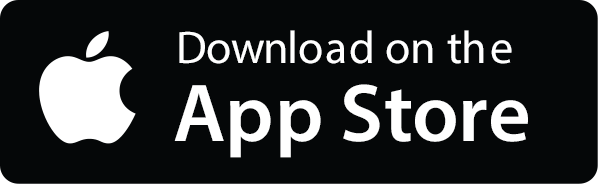
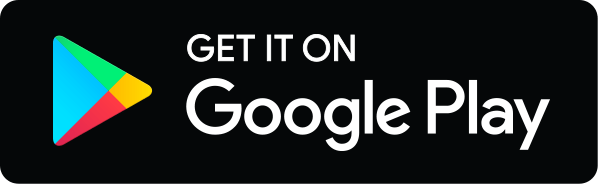
