By studying exercise and performance in hand-cycling in both activities of daily living and in Paralympic sport settings, new insights can be gained for rehabilitation practice, adapted physical activity, and sports. This review looks into the pros and cons of hand-cycling in both rehabilitation and optimal sports performance settings as suggested from the current—but still limited—scientific literature and experimentation. Despite the limited evidence-base and the diversity of study approaches and methodologies, this study suggests an important role for hand-cycling during and after rehabilitation, and in wheeled mobility recreation and sports. An approach that combines biomechanical, physiological, and psychosocial elements may lead to a better understanding of the benefits of hand-cycling and of the fundamentals of exercise in rehabilitation, activities of daily living, and sports.
Given the limited work capacity of wheelchair users in general, the stress of hand-rim wheelchair mobility results in fatigue and local discomfort of the upper extremities with the possible risk of repetitive strain injuries. Different experiments on lever- and crank-propelled wheelchairs have shown that alternative propulsion mechanisms (levers, hub cranks) are less straining and more efficient than hand rims. Hand-cycling (or hand-biking) has been found to be especially efficient, as well as less straining, and has become very popular over the last decades both in industrialized and developing countries in daily life and sports. Physiologic benefits of hand-cycling have been demonstrated in people with a chronic disability and even in early rehabilitation.
Both in daily life and Paralympic sports performance, hand-biking is an interesting mode of training and exercise with many practical benefits. For example, hand-biking is recommended to maintain physical fitness and prevent arteriosclerotic diseases. Also, hand-bikers can easily take part in training sessions together with participants in such able-bodied cyclic sports as cycling and roller-skating. Crank-propelled tricycle wheelchairs (ie, hand cycles) have in particular demonstrated their important role in more extreme environmental conditions in daily life in many non-Western countries. Such wheelchairs enable greater physiologic responses—expressed in higher peak power production, mechanical efficiency, and, thus, endurance capacity—compared with those from hand-rim propulsion. Lower muscular strain in terms of muscle activation was seen compared with hand-rim wheelchair propulsion. In addition, biomechanical studies—still few in number—have generated some understanding of the underlying mechanisms of the improved performance in hand-cycling, again compared with hand-rim wheelchair propulsion. Compared to hand-rim wheelchairs, hand-cycle wheelchairs enable higher velocities over a longer duration at the same or even a lower physical strain due to the following factors: (1) a natural grip of the hands to a well-formed handle bar, with the arms moving within the visual field, mostly making a fully circular motion and without the need for coupling-uncoupling actions in hand-rim propulsion; (2) the use of cranks and levers that allow the use of all flexor and extensor muscles around the arm-shoulder joints to actively contribute to external work over the full motion cycle; the latter in contrast to hand-rim propulsion where the discontinuous motion allows active work only during 30% to 40% of the cycle. The continuous power generation over the full cycle in hand-cycling is suggested to result in an increased gross mechanical efficiency for hand-cycling, which may be almost twice as high as that in hand-rim propulsion. This leads to a larger range of mobility, making hand-cycling a suitable mode of transportation and exercise mode at the recreational level in daily life as well as in fascinating high-level sports. Hand-cycling is interesting for persons with disabilities ranging from single-leg amputation/paralysis to complete spinal cord injuries.
History and hand-cycle technology
Already in 1655, Stephen Farfler ( http://de.wikipedia.org/wiki/Stephan_Farfler ), a watchmaker with paraplegia, built his own, mainly wooden, self-propelled three-wheeled arm-crank wheelchair. In the 1900s and particularly halfway into the twentieth century, solid, yet large and heavy asynchronous hand bikes without gears were used in Europe as transportation modes for persons with disabilities. In the late 1980s, the first modern hand bikes for persons with lower-limb disabilities were developed for recreation and sports. The development of lightweight ergonomic and efficient hand cycles was motivated by the increasing popularity of using the hand bike in a sports setting. Over the last decade, hand-biking in sports gained even more popularity, and was added as an event to the World Championships for the first time in 1998. In 2004, hand-biking appeared for the first time at the Paralympics in Athens ( www.paralympics.org ). Nowadays, Paralympics are closely associated with the “regular” Olympics and receive the accompanying media attention. It is thus expected that hand-biking and other Paralympic sports will increase even more in popularity in the coming years.
A strong ergonomics approach and thus the use of modern technology and lightweight and innovative materials has led to the reintroduction of the synchronous hand cycle in the mid-1980s for outdoor recreational use, where earlier types used asynchronous propulsion. These early hand cycles not only followed rules of bicycle technology, but also mimicked the existing arm-crank systems that became common for upper-body exercise testing in exercise physiology and rehabilitation and showed the first physiologic benefits (higher efficiency and peak power output and lower submaximal physical strain) of full cyclic upper body exercise of asynchronous arm cranking as opposed to hand-rim propulsion.
Disadvantages of the early hand cycles, such as weight, size, and limited maneuverability, have partly been overcome. The use of tracker or attach-unit systems ( Fig. 1 A), an add-on front-wheel crank unit fitted onto a conventional daily hand-rim wheelchair, has made the crank-propulsion mechanism more practical for mobility in daily outdoor life.
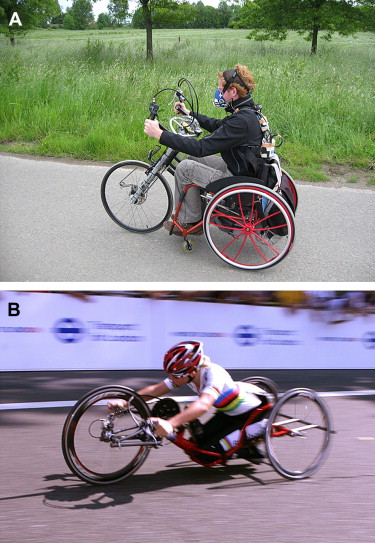
With the use of the “fifth wheel”, the hand-rim wheelchair can be converted into a multigear-based hand cycle for daily use ( www.doubleperformance.nl ). Here, persons sit in a supine position, comparable to a hand-rim wheelchair seating position. As was shown in different experiments, these hand cycles must be preferred in outdoor wheeling for longer distances over the hand-rim wheelchair. According to DoublePerformance, a hand-bike expertise center in the Netherlands and Belgium, hand bikes can be classified as shown in Fig. 2 . All existing types of (three-wheeled) hand bikes are shown, classified according to whether they are powered by arms alone or by both arms and the trunk. Also, values for frontal area are given, which are important for aerodynamics as is described below. Two-wheeled hand cycles also exist today, but have not been subject of experimental studies so far.
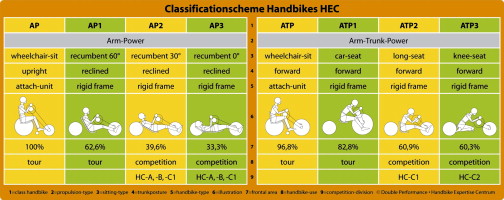
Hand-bike configuration
In sports, frames with a low center of gravity have been developed to optimize aerodynamics in performance. In Paralympics, hand cycles are rigid-frame, three-wheeled (two rear and one front wheel) and propelled by the arms using pedals and a gearing system in front of the athlete (see Fig. 1 B). Different hand-cycle configurations with different sitting postures are used (see Fig. 2 ), including those in which subjects lie completely supine (with low air friction, but with limited use of upper-body muscles), as well as those where subjects are in a kneeling position and actively involve the trunk function in propulsion (obviously at the cost of a higher air friction) (see Fig. 2 ). Those are all tricycle systems. A two-wheeled racer is also available ( www.doubleperformance.nl ). In the year 2000, the kneeling position was first introduced in hand-cycle competition by Kees van Breukelen ( www.doubleperformance.nl ) and today competitors are classified in arm power–only or arm-trunk power competition, depending on sitting position. Athletes compete in three functional divisions, men and women separated: lower-limb function partly or completely impaired and (1) complete loss of trunk stability, (2) a limited loss of trunk stability, or (3) a minimal loss of trunk stability ( www.paralympics.org ).
From an ergonomics perspective, wheeling and hand-cycle performance depends on vehicle mechanics, the hand-cycle–user interface, and the work capacity of the user/athlete ( Fig. 3 ). Each of these components has different characteristics that affect performance in sports and daily life. Such a systematic ergonomics evaluation and subsequent optimization affect performance, freedom of mobility, and endurance.
In daily life, where rolling resistance and internal friction are dominant resisting forces, maintenance and vehicle mechanics (mass, mass distribution, wheel/tire characteristics, and alignment) are critical. The flowchart (see Fig. 3 ) illustrates elegantly this complexity of performance-influencing factors within a combined physiologic and biomechanical framework of hand-cycling performance.
As stated above, in wheeled sports, different hand-cycle designs are used; important performance criteria include speed and time, but also performance capacity and ability level. With increasing velocity, good aerodynamics is increasingly important given the high velocities and their direct nonlinear effect on power requirement. Minimization of frontal area therefore is critical. In such Olympic sports as cycling and speed skating, minimizing frontal plane area and thus lowering frictional losses are equally important. Yet, aerodynamic design will affect the functional use of upper-body muscles.
In addition to aerodynamics and associated upper-body functions, other factors need to be considered when building a hand cycle for a specific user to improve comfort and mechanical efficiency. Zipfel and colleagues mention crank alignment, seat angle and position relative to cranks, crank handle type, footrest alignment, wheel camber, wheel-base length, gear ratios, seat-to-floor height, materials, and components. Zipfel and colleagues also mention current design problems of commercially available hand cycles: limited adjustability, poor trailing, poor aerodynamics, cables that get in the way of pedaling, minimal shock absorption, noncompatible components, gearing problems (which make it difficult to pedal uphill), difficulty with transfers, heaviness and bulkiness with transportation, and finally footrests dragging on the ground when making sharp turns. Developers have addressed most of these problems with a second-generation hand bike. In developing a third-generation hand bike, more advanced materials, finite element analysis software, and continued incorporation of bicycle technology and overall design innovation are necessary. Further advancements clearly also require continued biomechanical and physiologic study to obtain evidence-based design guidelines.
Hand-bike configuration
In sports, frames with a low center of gravity have been developed to optimize aerodynamics in performance. In Paralympics, hand cycles are rigid-frame, three-wheeled (two rear and one front wheel) and propelled by the arms using pedals and a gearing system in front of the athlete (see Fig. 1 B). Different hand-cycle configurations with different sitting postures are used (see Fig. 2 ), including those in which subjects lie completely supine (with low air friction, but with limited use of upper-body muscles), as well as those where subjects are in a kneeling position and actively involve the trunk function in propulsion (obviously at the cost of a higher air friction) (see Fig. 2 ). Those are all tricycle systems. A two-wheeled racer is also available ( www.doubleperformance.nl ). In the year 2000, the kneeling position was first introduced in hand-cycle competition by Kees van Breukelen ( www.doubleperformance.nl ) and today competitors are classified in arm power–only or arm-trunk power competition, depending on sitting position. Athletes compete in three functional divisions, men and women separated: lower-limb function partly or completely impaired and (1) complete loss of trunk stability, (2) a limited loss of trunk stability, or (3) a minimal loss of trunk stability ( www.paralympics.org ).
From an ergonomics perspective, wheeling and hand-cycle performance depends on vehicle mechanics, the hand-cycle–user interface, and the work capacity of the user/athlete ( Fig. 3 ). Each of these components has different characteristics that affect performance in sports and daily life. Such a systematic ergonomics evaluation and subsequent optimization affect performance, freedom of mobility, and endurance.
In daily life, where rolling resistance and internal friction are dominant resisting forces, maintenance and vehicle mechanics (mass, mass distribution, wheel/tire characteristics, and alignment) are critical. The flowchart (see Fig. 3 ) illustrates elegantly this complexity of performance-influencing factors within a combined physiologic and biomechanical framework of hand-cycling performance.
As stated above, in wheeled sports, different hand-cycle designs are used; important performance criteria include speed and time, but also performance capacity and ability level. With increasing velocity, good aerodynamics is increasingly important given the high velocities and their direct nonlinear effect on power requirement. Minimization of frontal area therefore is critical. In such Olympic sports as cycling and speed skating, minimizing frontal plane area and thus lowering frictional losses are equally important. Yet, aerodynamic design will affect the functional use of upper-body muscles.
In addition to aerodynamics and associated upper-body functions, other factors need to be considered when building a hand cycle for a specific user to improve comfort and mechanical efficiency. Zipfel and colleagues mention crank alignment, seat angle and position relative to cranks, crank handle type, footrest alignment, wheel camber, wheel-base length, gear ratios, seat-to-floor height, materials, and components. Zipfel and colleagues also mention current design problems of commercially available hand cycles: limited adjustability, poor trailing, poor aerodynamics, cables that get in the way of pedaling, minimal shock absorption, noncompatible components, gearing problems (which make it difficult to pedal uphill), difficulty with transfers, heaviness and bulkiness with transportation, and finally footrests dragging on the ground when making sharp turns. Developers have addressed most of these problems with a second-generation hand bike. In developing a third-generation hand bike, more advanced materials, finite element analysis software, and continued incorporation of bicycle technology and overall design innovation are necessary. Further advancements clearly also require continued biomechanical and physiologic study to obtain evidence-based design guidelines.
Synchronous versus asynchronous
One of the issues that has received some research attention in hand-cycling is the propulsion mode. In contrast to hand cycles in the middle of the twentieth century, which were mainly based on mere bicycle technology, today’s hand cycles all use a synchronous arm mode (ie, both hands propel in phase). This has considerable physiologic benefits (higher efficiency, peak power, lower local strain) as was demonstrated in different studies. Both arms move in the same angular pattern, thus preventing a conflict with the simultaneous steering task, which is expressed in a higher-efficiency and peak-power output. With an apparent constant external workload at the same speed and slope on a motor-driven treadmill for synchronous and asynchronous propulsion, synchronous hand-cycling appears more efficient, with less strain (lower arms/hands) and higher peak performance capacity (speed, power). Some have suggested that the lower peak performance and mechanical efficiency in asynchronous cycling can be explained by increased cocontraction of the muscles in the upper extremities and trunk to combine power production with stable steering. Indirectly, this explains the absence of systematic and consistent differences between synchronous and asynchronous arm-crank exercise, where no steering is required. With a synchronous crank setup, “trunk power,” a new type of propulsion, can be used, as described by, among others, Zipfel and colleagues. However, he use of trunk power is possible by athletes or users with functional trunk muscles and with good abdominal strength. Crank arms can be longer, wider, and positioned further from the body. By leaning forward with each push, the mass of the upper body is used to add power to the stroke. Thus, not only arm, chest, and upper-back muscles are used, but also the abdominal muscles and the lower back contribute to propulsion.
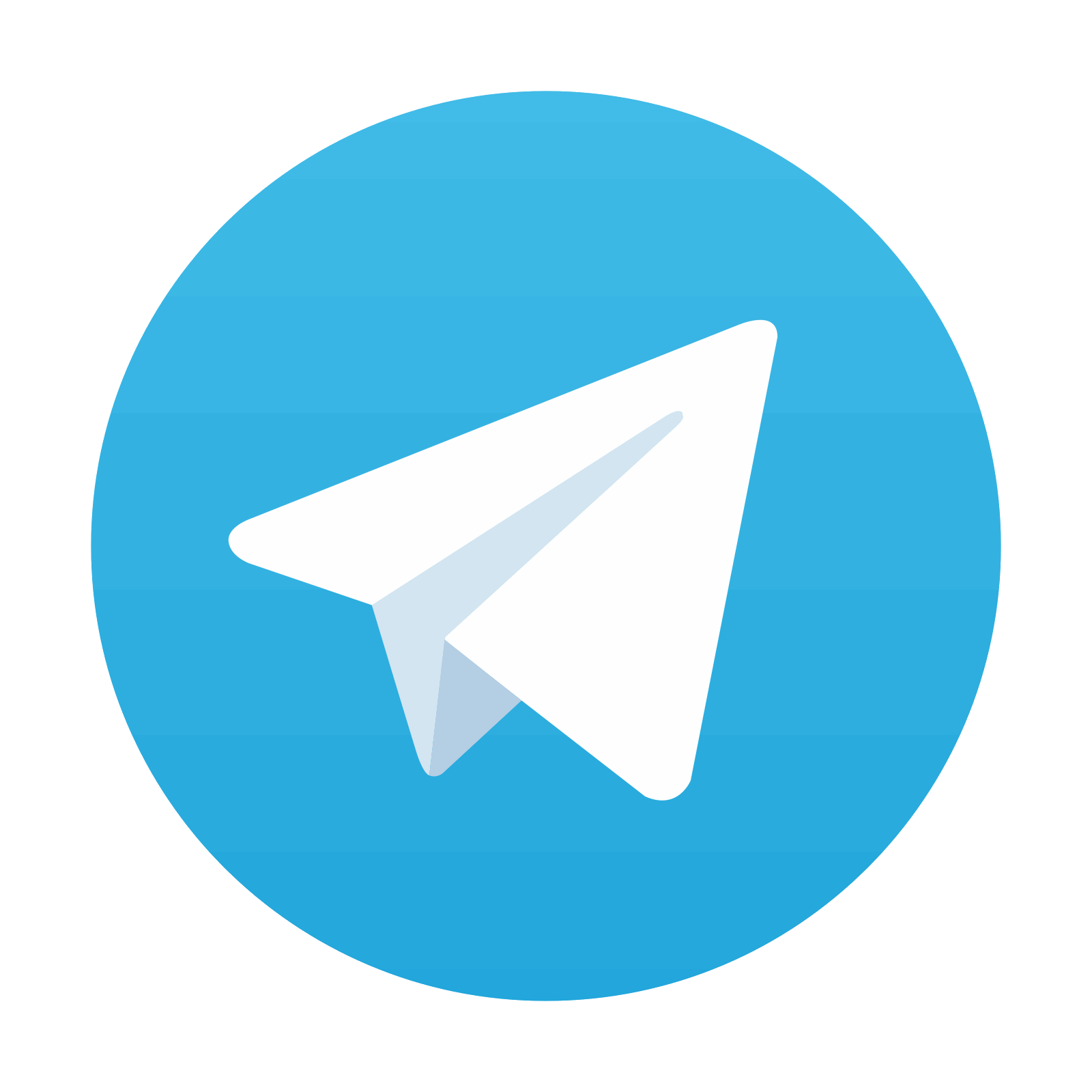
Stay updated, free articles. Join our Telegram channel

Full access? Get Clinical Tree
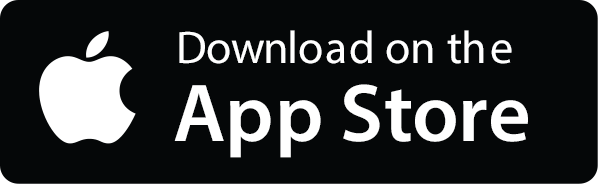
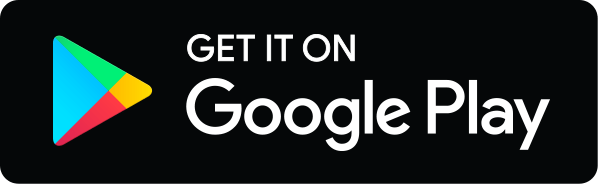