Gross Anatomy of the Hip
Ray C. Wasielewski
Sharat Kusuma
Aaron G. Rosenberg
Pelvic Bony Anatomy
The bony pelvis is formed by the sacrum and coccyx which serve to connect the right and left innominate bones (the os coxae) which is formed from three separate ossification centers: the ilium, the ischium, and the pubis. In addition to these primary centers, secondary centers at the iliac crest, anterior inferior spine, ischial tuberosity, pubic symphysis, and triradiate cartilage at the center of the acetabulum all contribute to the final shape and structure of the pelvic bones. At age 13 to 14, the mostly ossified bones of the ilium, ischium, and pubis unite at the acetabulum, forming a Y-shaped triradiate cartilage that proceeds to fusion by age 15 to 16. The other secondary centers unite and fuse between the ages of 20 and 22.
The acetabular surface is orientated approximately 45 degrees caudally and 15 degrees anteriorly (1,2). While it has a mostly circular contour in its superior margin, it has only enough hemispherical depth to allow for 170 degrees of femoral head coverage. Within the acetabulum, femoral head coverage is augmented by the labrum, which runs circumferentially around the acetabular perimeter. It becomes thickened at the base of the fovea, where it is called the transverse acetabular ligament. Two strong osseous columns of bone surround the acetabulum anteriorly and posteriorly, transmitting stresses between the trunk and lower extremities (Fig. 2.1). These columns vary in thickness as they pass in front of and behind the acetabulum.
Surgical Considerations
In general, expanding the acetabular diameter by reaming to more than one-third of the anatomic diameter (i.e., reaming a size 54 acetabulum beyond a size 72) risk creating a pelvic discontinuity, rendering the columns incompetent (3). Overreaming the acetabulum during total hip arthroplasty (THA) may also prevent adequate circumferential hoop stresses when press-fitting the acetabular component into an undersized acetabulum. Excessive reaming of the columns will also decrease the available bone depth, limiting the screw purchase needed for peripheral and transacetabular screws to augment acetabular component fixation. Reaming one-quarter of the acetabular diameter is more likely to be safe (i.e., reaming a size 56 acetabulum to a size 70), preserving approximately 75% of the cross-sectional bone of the anterior and posterior columns (3).
Important surgical landmarks within the acetabulum or arthroplasty include the anterior and posterior brim, the base of the fovea, and the transverse acetabular ligament. The anterior and posterior brim can help determine if appropriate acetabular component anteversion and flexion are present. The base of the fovea serves as a guide to the extent to which the acetabulum can be reamed medially. It is important to locate the fovea when large medial osteophyte obscures the inferior aspect of the acetabulum. Failure to remove this osteophyte with adequate medial reaming may result in a lateralized acetabular component. However, medialization may be contraindicated in the patient with an inflammatory arthropathy, where protrusion of the hip may have occurred, often resulting in obliteration of the fovea. In such a case, only acetabular expansion is required along with morselized bone grafting of the medial wall defect.
The transverse acetabular ligament provides a landmark which can aid in identifying the inferior-most aspect of the acetabulum, particularly when the acetabulum is dysplastic. It allows the inferior-most aspect of the acetabulum to be identified even in the congenitally dislocated hip (CDH) where the femoral head has never been within the acetabulum. This ligament also provides a physical restraint to drifting of the acetabular reamer inferiorly in cases where the superior acetabular bone is sclerotic. However, when ossified it may tend to force reamers superiorly, particularly in cases where the superior acetabular bone is osteopenic (e.g., in transplant patients and patients on steroids).
Understanding the Relationship of the Interior Acetabular Surface
The anterior superior iliac spine (ASIS) is an extra-acetabular landmark that is helpful for identifying the location of competent acetabular bone on which to anchor the acetabulum, as well as a guide for the placement of transacetabular screws. It should be noted that as the acetabulum is moved superiorly between the columns, bone stock for screw placement decreases, as do the areas for safe transacetabular screw placement. The quadrant systems, as described by Wasielewski et al. (4), are useful in avoiding injury to neural
and vascular structures and will be discussed in greater detail later in this chapter.
and vascular structures and will be discussed in greater detail later in this chapter.
The Femur
The femur is the longest and strongest bone in the human body. Its length is necessary to accomplish the biomechanical needs of gait while its strength is needed to transmit both muscular and weight-bearing forces. It is mostly cylindrical throughout it length, and it is anteriorly and laterally bowed in its midportion. The proximal metaphysis and neck are anteverted (externally rotated about the long axis) by approximately 15 degrees in relationship to a line tangential to the posterior aspect of the femoral condyles (2,5,6).
Surgical Considerations
The extent of bowing is clinically relevant because when excessive, it may not be possible to utilize long straight implants without considerable undersizing. Abnormalities of anteversion may make it difficult to utilize fixed stems (i.e., anteversion not adjustable) without considerable undersizing of the component or osteotomy to correct the rotational abnormality. To quantitate abnormal anteversion prior to THA, a CT scan can be used to evaluate the anteversion. It will be easier to approach and dislocate the excessively anteverted hip (as in CDH) through an anterolateral surgical approach. Conversely, a retroverted hip (old slipped capital femoral epiphysis) may be more easily accessed from a posterolateral approach.
The angle between the femoral shaft and the neck is approximately 125 degrees. In most hips, the center of the femoral head is at the level of the tip of the greater trochanter. As the neck–shaft angle increases, the center of the head comes to lie above the level of the trochanter (resulting in coxa valga). A decreased neck–shaft angle results in coxa vara. Also, the distance between the center of the femoral head and the lateral aspect of the trochanter can vary independent of the neck–shaft angle (although patients with increased valgus tend to have less offset, whereas patients with increased varus have more offset). These variants are important because if they are anatomically normal, they need to be reconstructed with the use of femoral components with similar offset and neck–shaft angulation. If the variant is pathologic, it is necessary to re-establish normal hip joint kinematics and leg length.
The proximal femoral metaphyseal orientation and shape have great variability (7,8,9,10,11). Although internal dimensions tend to correlate with one another, it is impossible to predict which configuration is present unless CT scans of the proximal femur are obtained prior to surgery. It is not until the neck cut is made during femoral arthroplasty that this geometry is best appreciated and assessed. The canal configuration may create problems for a cementless stem with fixed proximal geometries. Not only must cementless stems fit the anterior–posterior and medial–lateral dimensions of the canal, they also need to maximize the endosteal contact down the length of their porous coating. The proximal endosteal femoral geometry is demonstrated adequately for surgical planning on preoperative radiographs. Endosteal expansion of the isthmus with age results in the stovepipe femoral configuration (12,13). The proximal femur of younger patients tends to have a trumpetlike or champagne-fluted configuration. Because these different configurations affect the ability of the porous coating of a particular implant to be adequately apposed to subchondral bone, Dorr (14) and Spotorno (15) have developed indexes to characterize proximal femoral configuration. The Dorr index is a ratio of the canal diameter at the level of the lesser trochanter to the canal diameter at a point 10 cm distal (Fig. 2.2). As the canal calcar isthmus ratio (the Dorr index) approaches 1, prosthesis fill proximally and distally is compromised. Patients with stovepipe configurations may require more porous surface on their femoral implants to adequately contact the endosteal femoral surface, or a cemented implant may be considered. The ability of these indexes to predict the success of cementless femoral implants is still being elucidated. Singh et al. (16) have created an index to grade the loss of trabeculae that occurs with osteopenia. Because many of these
trabeculae are removed with the required neck resection for removal of the femoral head at arthroplasty, this osteopenia measurement is likely less important than the quality of bone against the ingrowth surface. The influence of bone quality on successful hip replacement is presently being determined (17,18).
trabeculae are removed with the required neck resection for removal of the femoral head at arthroplasty, this osteopenia measurement is likely less important than the quality of bone against the ingrowth surface. The influence of bone quality on successful hip replacement is presently being determined (17,18).
The Anatomic and High Hip Center Quadrant Systems
The nerves and vessels that course around the acetabulum and proximal femur are demonstrated in this and many other anatomy and surgery texts (4,19,20,21,22,23,24,25,26,27,28). The acetabular anatomy and surrounding nerves and vessels can be easily understood by using the acetabular quadrant system (4). Use of this system allows the surgeon to know the location of intrapelvic structures with respect to fixed points of reference within the acetabulum. For primary or revision acetabular arthroplasty, a line drawn from the ASIS through the center of the acetabulum defines anterior and posterior quadrant locations. If this line is then bisected with a perpendicular at its midpoint, four quadrants are formed. The anatomic and high hip center (HHC) quadrant systems (Fig. 2.3) can be used to locate the safe and dangerous zones for the transacetabular placement of screws; but they also can be used as a guide for retractor placement, for drilling acetabular anchoring holes for graft fixation, or to estimate bone depth in a specific acetabular zone.
The use of the anterior quadrants for the placement of screws or anchoring holes, or to help secure retractors may endanger the external iliac artery and vein and the obturator nerve, artery, and vein. The external iliac vessels lie opposite the anterior superior quadrant, and the obturator neural and vascular structures lie opposite the anterior inferior quadrant. These structures lie close to the pelvic bone, with little protective interposition of soft tissue or muscle. The risk of injury is further exacerbated by the lack of bone in the anterior quadrants (Fig. 2.4A). Violation of the inner acetabular bone opposite the anterior quadrants, with cement or transacetabular screws, should be avoided whenever possible (29). Retractor placement over the anterior column should be done with care.
The polar zone of the acetabulum is often violated during excessive reaming, placement of a cement anchoring hole, or transacetabular screw placement. Occasionally, medial migration of the acetabular component can occur with implant failure. This medial acetabular zone is opposite the external iliac vein and the obturator nerve, artery, and vein that course along the superior quadrilateral surface. The risk to these structures is increased during aging by the relatively small obturator internus muscle and the shallow acetabular bone depth opposite the structures.
The sciatic nerve and the superior gluteal nerve and vessels course opposite the posterior superior quadrant, and the inferior gluteal and internal pudendal structures are opposite the posterior inferior quadrant. In contrast to the shallow bone in the anterior quadrants, the bone depth in the posterior quadrants is 25 mm or greater in the central regions (Fig. 2.4A). Screws and anchoring holes can be placed relatively safely in these zones. In addition, the sciatic nerve can be gently displaced during retractor and screw placement, reducing the likelihood of injury. The sciatic notch is easily palpable, and the superior gluteal nerve and vessels can be protected. The inferior gluteal and internal pudendal neural and vascular structures are not palpable at the level of the ischial spine. They are relatively mobile and can be protected if retractors are placed directly against the bone of the posterior column.
The quadrants for an HHC acetabulum—although constructed similarly—are different from those formed at the normal anatomic acetabulum. HHC quadrants are formed by drawing a line from the ASIS through the center of the newly positioned acetabulum. A second line drawn perpendicular to the first at the acetabular midpoint forms four clinically useful HHC acetabular quadrants (Fig. 2.3B).
The peripheral halves of the posterior superior and posterior inferior HHC quadrants contain the best available bone stock (Fig. 2.4B) and are relatively safe for transacetabular screw placement (Fig. 2.3B). The entire anterior superior and anterior inferior HHC quadrants, as well as the central half of the posterior superior and posterior inferior quadrants, should be avoided because of the close proximity of intrapelvic structures and the paucity of protective musculature on the inner wall of the pelvis opposite these zones.
The modified HHC and anatomic acetabular quadrant systems provide the surgeon with a reproducible intraoperative guide to the location of periacetabular neural and vascular structures. Knowledge of the mechanisms by which the nerves and vessels are injured, along with the ability to locate structures with respect to surgical landmarks, will allow the surgeon to minimize injuries during THA.
Hip Joint Capsule and Ligaments
The articular capsule of the hip is strong and dense, contributing substantially to joint stability (Fig. 2.5). The capsule attaches along the anterior and posterior periphery of the acetabulum just outside the acetabular labrum. Making anterior and posterior incisions between the capsule and labrum allow retractors to be placed safely over the anterior and posterior columns. Inferiorly, the capsule is attached to the transverse acetabular ligament. Running distally the capsule attaches anteriorly to the femur along the intertrochanteric line, but has an arched free border that results in only partial covering of the femoral neck posteriorly (Fig. 2.5B). The femoral neck is thus intracapsular anteriorly, but posteriorly the basicervical portion and intertrochanteric crest are extracapsular. Most of the capsular fibers of are longitudinally orientated as they traverse from the pelvis to the femur,
except for the circular fibers of the zona orbicularis located posteriorly and inferiorly (Fig. 2.5B,C).
except for the circular fibers of the zona orbicularis located posteriorly and inferiorly (Fig. 2.5B,C).
![]() Figure 2.5. Periarticular ligaments of the hip joint. A: Anterior view of the hip joint. B: Posterior view of the hip joint. C: Coronal section of the hip joint. |
Two strong accessory ligaments, the iliofemoral and the pubofemoral ligaments, reinforce the anterior portion of the capsule. While the ischiofemoral ligament reinforces the posterior capsule.
Surgical Considerations
The iliofemoral ligament—often referred to as the Y ligament of Bigelow—is a fan-shaped ligament that resembles an inverted letter Y (Fig 2.5A,B). The apex of the ligament is attached to the lower portion of the anterior inferior iliac spine (AIIS), and the diverging fibers of the Y fan out to attach along the intertrochanteric line. The fibers of the iliofemoral ligament become taut in full extension, providing a checkrein to hip extension beyond neutral. The superior portion may resist excessive external rotation. When this ligament is contracted, a flexion/internal rotation contracture results, which may require release THA. It is particularly important to correct this internal rotation contracture if a posterior approach to the hip is done (otherwise a tendency toward hip internal rotation will result). An anterolateral approach may be preferred as the approach itself releases this contracture.
The pubofemoral ligament (Fig. 2.5A) is a thickening of the inferior and medial part of the anterior capsule arising from the pubic portion of the acetabular rim and the obturator aspect of the superior pubic ramus, passing below the neck of the femur and blending with the inferior-most fibers of the iliofemoral ligament. The fibers of the pubofemoral ligament become taut in hip extension and abduction. In trying to correct a hip adduction contracture at arthroplasty, these fibers may need to be released to provide for adequate hip abduction.
The ischiofemoral ligament reinforces the posterior surface of the capsule arising from the ischial portion of the acetabular rim. Its fibers spiral laterally and upward, arching across the femoral neck to blend with the fibers of the zona orbicularis. The spiral fibers tighten during extension but loosen or unwind during hip flexion. Other fibers traverse horizontally and attach to the inner surface of the greater trochanter, providing a check to internal hip rotation (27).
The twisted orientation of the capsular ligaments surrounding the hip joint provides for a screw home effect in full extension. Hip extension coils and tightens these ligaments, making extension the close-packed position of the joint and the position of maximum stability (27). Interestingly, in full extension the articular surfaces of the joint are not in optimal contact. The position of optimal articular contact (flexion, abduction, and external rotation) is the loose-packed position because flexion and lateral rotation tend to uncoil the ligaments. Because the joint surfaces are neither maximally congruent nor close packed, the hip joint is at greatest risk for traumatic dislocation when flexed and adducted (30,31).
Hip Joint Musculature
The muscles of the hip joint operate as part of a closed kinematic chain-link system. The muscles are unique in their length, large cross section, and large areas of origin and insertion (Fig. 2.6). In combination with the large range of motion available at the hip joint, result in muscle function that is dependent on limb position (Table 2.1). The average range of motion accommodated at the undiseased hip is flexion to 120 degrees, extension to 30 degrees, abduction to 45 to 50 degrees, adduction 20 to 30 degrees, internal rotation to 35 degrees, and external rotation to 45 degrees (32,33). Normal gait on level ground requires a minimum of 30 degrees of flexion, 10 degrees of hyperextension, and 5 degrees of abduction, adduction, and both internal and external rotation (34). Walking on uneven terrain or stairs will increase the need for joint range beyond that required for level ground, as will activities such as sitting in a chair or sitting cross-legged (35,36,37,38,39).
Table 2.1 Muscles of the Hip Joint: Muscle Group Actions and Innervations | ||||||||||||||||||||||||||||||||||||||||||||||||||||||||||||||||||||||||||||||||||||||||||||||||||||||||||||||||||||||||
---|---|---|---|---|---|---|---|---|---|---|---|---|---|---|---|---|---|---|---|---|---|---|---|---|---|---|---|---|---|---|---|---|---|---|---|---|---|---|---|---|---|---|---|---|---|---|---|---|---|---|---|---|---|---|---|---|---|---|---|---|---|---|---|---|---|---|---|---|---|---|---|---|---|---|---|---|---|---|---|---|---|---|---|---|---|---|---|---|---|---|---|---|---|---|---|---|---|---|---|---|---|---|---|---|---|---|---|---|---|---|---|---|---|---|---|---|---|---|---|---|
|
Flexors
The primary hip flexors are the iliopsoas, rectus femoris, and sartorius. These anterior hip muscles include the quadriceps consisting of the three anterior thigh muscles: vastus lateralis, vastus intermedius, and vastus medialis along with the rectus femoris, the only quadriceps that crosses both the hip and the knee joints. The two origins of the rectus include a direct head that arises from the AIIS, and an indirect head that blends with the superior acetabular labrum.
The iliopsoas muscle consists of two separate muscles, the iliacus muscle and the psoas major muscle, which converge together into a common tendon to insert onto the lesser trochanter of the femur. The iliopsoas muscle has a broad origin including the iliac crest and fossa, sacral ala, and iliolumbar and sacroiliac ligaments (the iliac contribution); and the sides of the bodies of the 12th thoracic through fourth lumbar vertebrae, transverse processes of the first through the fifth lumbar vertebrae, and the intervertebral disks (the psoas contribution). Its tendon is often seen traversing across the inferior aspect of the hip joint just outside the hip capsule. When an inferior capsular resection is required, it serves as an important landmark for the depth to which dissection can be carried. If this tendon is excessively taut (secondary to a hip flexion contracture pre-arthroplasty or after significant surgical hip lengthening), the tendinous portion can be tenotomized just distal to the point it crosses over the pelvic brim, leaving the muscular portion intact.
The rectus femoris muscle crosses both the hip and knee joint. It has two origins, a straight head originating from the AIIS, and a reflected head originating from just above the anterior inferior acetabular rim. These heads combine into a central aponeurosis which proceeds down the thigh and inserting as a common tendon into Gerdy’s tibial tuberosity. The rectus femoris flexes the hip joint and extends the knee joint. The position of the knee during hip flexion does affect its ability to generate force at the hip. The rectus femoris provides its strongest contribution to hip flexion when the knee is flexed. Knee flexion preloads the quadriceps muscles, strengthening its force across the hip joint. On the other hand, simultaneous hip flexion and knee extension considerably shorten this muscle across both joints, minimizing preload and decreasing its ability to generate a hip flexion moment. When a hip flexion contracture exists (or when the
contracted hip has been lengthened by arthroplasty), release of the reflected head and transverse release of the fascia along the undersurface of the rectus may help improve knee flexion with the hip extended. After adequate release, the knee should be able to be bent to 90 degrees with the hip in the fully extended position.
contracted hip has been lengthened by arthroplasty), release of the reflected head and transverse release of the fascia along the undersurface of the rectus may help improve knee flexion with the hip extended. After adequate release, the knee should be able to be bent to 90 degrees with the hip in the fully extended position.
The sartorius muscle arises from the ASIS. It also crosses the hip and knee joints to insert on the medial aspect of the proximal tibia in association with the other pes anserinus muscles. The sartorius flexes and abducts the hip, and it also flexes the knee. Although it crosses the knee, it is not substantially affected by knee position because only a relatively small change in length occurs with knee motion.
The tensor fascia lata muscle originates lateral to the sartorius on the anterolateral lip of the iliac crest its muscle fibers enclosed between the layers of the fascia lata which blend together distally to form the iliotibial band. The tensor fascia flexes, abducts, and weakly rotates the hip medially, although the tensor’s contribution to hip abduction may be dependent on simultaneous hip flexion (40,41) and the most important contribution of the tensor fascia lata may be in maintaining tension in the iliotibial band to support knee extension during stance.
Other secondary flexors are the pectineus; the adductor longus, brevis, and magnus; and the gracilis muscles; as well as the anterior portions of the gluteus minimus and medius muscles. Each is capable of contributing to hip joint flexion depending on hip position. The gracilis crosses both the hip and knee joints and acts as a hip flexor when the knee is extended but not when the knee is flexed (42,43).
Extensors
The primary hip joint extensors are the gluteus maximus and hamstring muscles with assistance from the ischiocondylar fibers of the adductor magnus. Posteriorly, the massive gluteus maximus muscle arises from the posterior gluteal line, iliac crest, and a portion of the posterior surface of the sacrum and coccyx, the tendinous aspect of the sacrospinous and sacrotuberous ligaments, and the underside of the gluteus aponeurosis. About three-fourths of the gluteus maximus tendon blend with the tendon of the tensor fascia muscle to form the tethering band of the iliotibial tract which crosses both the hip and knee joints and is thickened between the iliac crest tubercle and the tibia. This band overlies the trochanteric bursa against the greater trochanter and
assists in stabilizing the pelvis during gait as well as actively abducting the hip. One-fourth of the gluteus maximus tendon inserts directly into the proximal femur at the gluteal tuberosity and the lateral intermuscular septum (Fig. 2.7) to function as the prime decelerator of the hip during gait.
assists in stabilizing the pelvis during gait as well as actively abducting the hip. One-fourth of the gluteus maximus tendon inserts directly into the proximal femur at the gluteal tuberosity and the lateral intermuscular septum (Fig. 2.7) to function as the prime decelerator of the hip during gait.
The gluteus maximus moment arm generates the maximum hip extension strength (to resist trunk flexion) when the hip joint is in neutral position (44) and laterally rotates the femur and stabilizes the knee through its insertion in the iliotibial tract.
The hamstring muscles also contribute to hip extension. These include the long head of the biceps femoris, the semitendinosus, and the semimembranosus muscles. They originate at the ischial tuberosity and extend the hip and flex the knee. However, their combined strength is less than that of the gluteus maximus. Hamstring strength increases with hip flexion, whereas maximus strength decreases as the hip flexes beyond neutral (44).
Abductors
Abduction of the hip is brought about predominantly by the gluteus medius and gluteus minimus muscles. The tensor fascia lata may significantly contribute to abduction but only during concomitant hip flexion. The gluteus medius arises from both the external surface of the ilium and the
underside of the tensor fascia. The extensive origin of the gluteus medius serves as a powerful anchor for the muscle and maximizes the abductor function by directing tension laterally along the iliac crest through the fascia lata and iliotibial tract. The gluteus medius has anterior, middle, and posterior parts that function asynchronously during movement at the hip (45). While all fibers contribute significantly to hip abduction, the anterior fibers are active in hip flexion and medial rotation, whereas the posterior fibers function during extension and lateral rotation. Although it is a prime abductor, its anterior insertion into the greater trochanter also makes it an internal rotator of the hip. Hip reconstructions that increase the tension of the gluteus medius may also cause internal rotation as a result. Its fibers are oriented in an oblique fashion and can be differentiated from the gluteus maximus and minimus by this characteristic during difficult surgical dissections through this region.
underside of the tensor fascia. The extensive origin of the gluteus medius serves as a powerful anchor for the muscle and maximizes the abductor function by directing tension laterally along the iliac crest through the fascia lata and iliotibial tract. The gluteus medius has anterior, middle, and posterior parts that function asynchronously during movement at the hip (45). While all fibers contribute significantly to hip abduction, the anterior fibers are active in hip flexion and medial rotation, whereas the posterior fibers function during extension and lateral rotation. Although it is a prime abductor, its anterior insertion into the greater trochanter also makes it an internal rotator of the hip. Hip reconstructions that increase the tension of the gluteus medius may also cause internal rotation as a result. Its fibers are oriented in an oblique fashion and can be differentiated from the gluteus maximus and minimus by this characteristic during difficult surgical dissections through this region.
The gluteus minimus lies beneath the gluteus medius and contributes about one-fifth of the abductor power to the hip. The muscle arises from the ilium between the anterior and inferior gluteal lines. The muscle becomes tendinous over the anterior capsule and inserts partially into the capsule superiorly deep to the medius insertion along the anterior portion of the greater trochanter.
Surgical Considerations
Using the free-body formula (46), the force required by the abductor musculature (FAB) to maintain hip equilibrium during gait is b/a × 5 W/6, where a is the abductor moment arm, b is the distance from the center of gravity to the hip center, and W is body weight. Thus, FAB ≈ 2.5 W. After THA, until the abductor musculature can generate this force, the patient will have Trendelenburg sign on attempted single limb stance or have a Trendelenburg lurch with ambulation (47). The Trendelenburg lurch is the body’s attempt to compensate for abductor weakness by bringing the center of gravity closer to the hip center (i.e., the patient leans toward the operative side with weight bearing decreasing the moment arm). The distance the patient with abductor weakness must shift his weight over the affected limb will be proportional to the magnitude of abductor weakness. Interestingly, the particular approach utilized for THA (e.g., Kocker, Hardinge, transtrochanteric) does not seem to influence the rate of postoperative Trendelenburg gait (48,49).
THA may also cause the gluteus minimus muscle to be tight—resulting in an internal rotation contracture—when the hip is lengthened from a shortened contracted position. It may be necessary to release the undersurface of the gluteus minimus from caudad to cephalad to allow for appropriate hip external rotation and prevent a tendency toward internal rotation that can lead to posterior hip dislocation.
Adductors
Adductor muscles of the hip include the adductor brevis, adductor longus, adductor magnus, pectineus, and gracilis. The adductor longus, brevis, and magnus muscles arise from the external surfaces of the inferior pubic ramus and the ischial ramus, fanning out laterally to insert along the linea aspera along the posterior aspect of the femur. The gracilis originates on the inferior pubic ramus and the edge of the symphysis pubis, inserting on the medial surface of the proximal tibia as part of the pes anserinus.
Surgical considerations
The combined strength of this muscle group (isometric torque of adduction) is greater than that of the abductor musculature (50). Adduction contractures are frequently found in pathologic conditions of the hip joint. Because adduction contractures left uncorrected may result in a dislocation diathesis, an adductor tenotomy through a separate medial incision should be considered at the start of joint replacement arthroplasty to address this problem. To complete the release of a severe adduction contracture, release of the pubofemoral ligament and inferior capsule should be done when the hip joint is exposed.
External Rotators
The short muscles of external rotation include the obturator internus and externus, the superior and inferior gemellus, the quadratus femoris, and the piriformis muscles. The anterior convexity of the neck, together with the backward projection of the intertrochanteric crest, gives these muscles excellent mechanical advantage in externally rotating the hip joint via insertion on the posterior border of the greater trochanter (9). The obturator internus muscle originates from the inner perimeter of the obturator foramen of the pelvis and emerges through the lesser sciatic foramen to insert on the medial surface of the greater trochanter. The two gemelli muscles, closely associated with the obturator internus muscle, blend together as they insert into the greater trochanter. The piriformis muscle emerges from the greater sciatic
foramen and inserts into the upper border of the greater trochanter. The surrounding structures are referenced relative to this tendon, prefaced by inferior and superior based on whether they exit above or below the piriformis. The sciatic nerve passes usually below the piriformis to lie on top of the short external rotators (Fig. 2.7). If the surgeon tags the rotators with suture and reflects them posteriorly, the nerve is protected. However, it is important to note that in 10% the common peroneal division of the sciatic nerve can pass through separate divisions of the piriformis. The obturator externus muscle originates on the external perimeter of the obturator foramen, crossing posterior to the hip joint to insert into the trochanteric fossa. Its tendon lies just outside the posterior inferior joint capsule and can be used to reinforce the inferior closure of the posterior tissues. The quadratus femoris muscle is a thick quadrangular muscle that originates on the ischial tuberosity and inserts on the quadrate line of the femur that runs caudad from the intertrochanteric crest. Removal of this muscle is often necessary to adequately expose the lesser trochanter for neck resection level determination.
foramen and inserts into the upper border of the greater trochanter. The surrounding structures are referenced relative to this tendon, prefaced by inferior and superior based on whether they exit above or below the piriformis. The sciatic nerve passes usually below the piriformis to lie on top of the short external rotators (Fig. 2.7). If the surgeon tags the rotators with suture and reflects them posteriorly, the nerve is protected. However, it is important to note that in 10% the common peroneal division of the sciatic nerve can pass through separate divisions of the piriformis. The obturator externus muscle originates on the external perimeter of the obturator foramen, crossing posterior to the hip joint to insert into the trochanteric fossa. Its tendon lies just outside the posterior inferior joint capsule and can be used to reinforce the inferior closure of the posterior tissues. The quadratus femoris muscle is a thick quadrangular muscle that originates on the ischial tuberosity and inserts on the quadrate line of the femur that runs caudad from the intertrochanteric crest. Removal of this muscle is often necessary to adequately expose the lesser trochanter for neck resection level determination.
Internal Rotators
The muscles producing internal rotation of the hip joint do so secondarily, as they all have other primary functions. These muscles all have lines of pull anterior to the hip joint—directed from lateral to medial at some point through the normal hip range of motion—contributing to internal rotation. The more consistent medial rotators are the anterior fibers of the gluteus medius and minimus and the tensor fascia lata muscles. Other muscles that contribute to internal rotation are listed in Table 2.1.
Extrapelvic Vasculature
Common Femoral Vessels
The common femoral artery is an extension of the external iliac artery as it passes under the inguinal ligament. It courses directly anterior and medial to the hip capsule, from which it is separated by only the iliopsoas tendon (Fig. 2.8). The femoral artery is defined proximally at the level of the inguinal ligament and distally at the adductor hiatus of the thigh. The femoral triangle, bounded proximally by the inguinal ligament, medially by the adductor longus, laterally by the sartorius, and at the floor by the iliacus, psoas major, pectineus, and adductor brevis, contains from medial to lateral the femoral nerve, artery and vein.
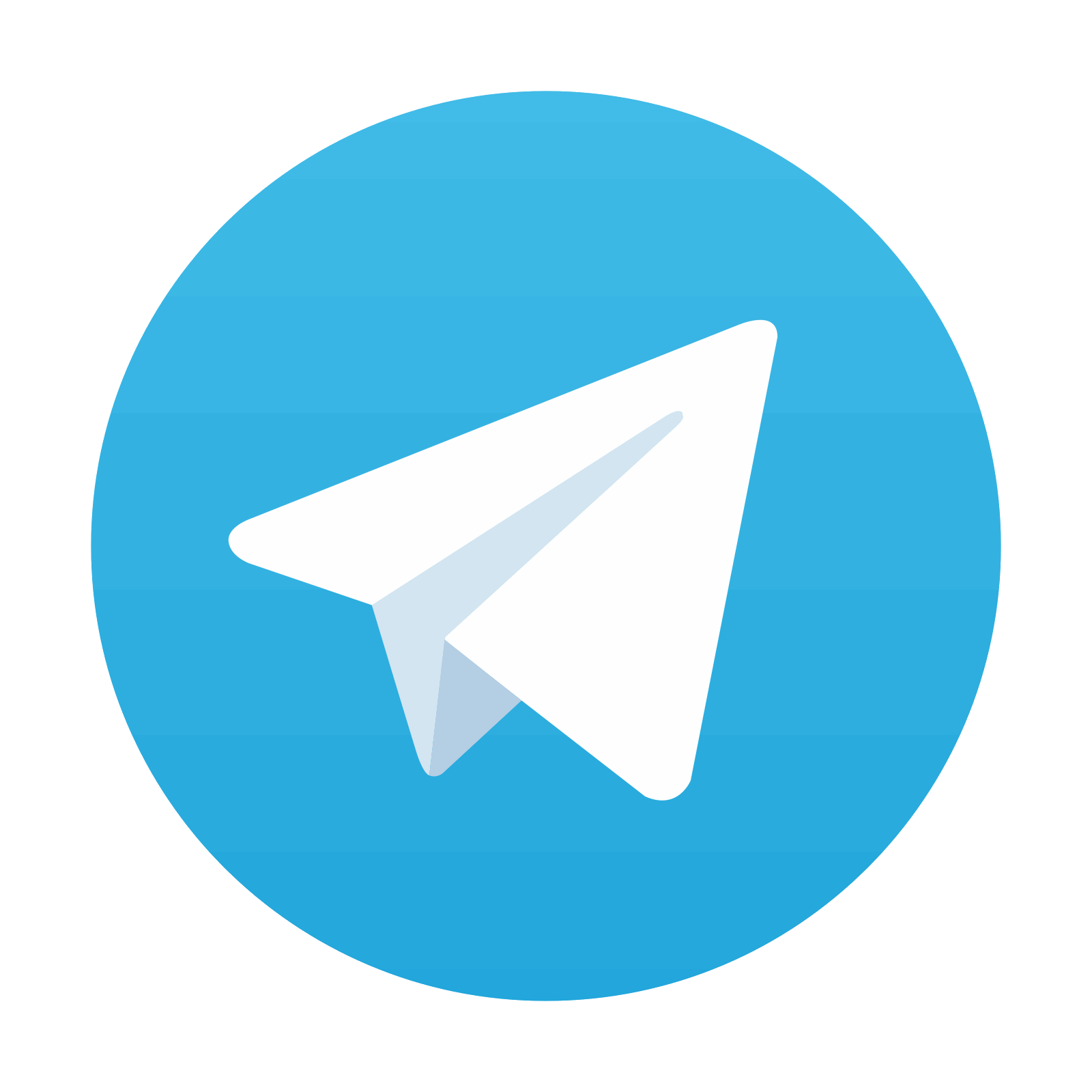
Stay updated, free articles. Join our Telegram channel

Full access? Get Clinical Tree
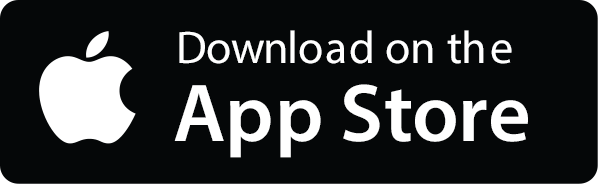
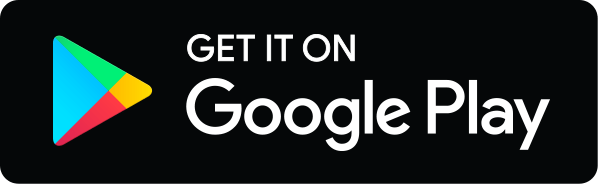