Glucocorticoids remain part of the treatment strategy in many rheumatic diseases, because of their anti-inflammatory and immunosuppressive actions. Unfortunately, their clinically desired effects are linked to adverse effects, especially at higher dosages and longer duration of treatment. In this review, we describe new insights into the mechanisms of anti-inflammatory glucocorticoid actions and provide an update on recent approaches to improve the risk/benefit ratio of glucocorticoid therapy. Improved knowledge of the immunomodulatory role of endogenous glucocorticoids has evolved, and we report on the therapeutic potential of targeting glucocorticoid pre-receptor metabolism for metabolic and inflammatory diseases.
Introduction
Glucocorticoids (GCs) are part of the treatment strategy in many rheumatic diseases, because of their anti-inflammatory and immunosuppressive effects . Their efficacy and fast onset of action, especially at higher dosages, allow treatment of severe flares and acute exacerbations, as well as bridging the time lag of other drugs. In vasculitis, systemic GCs are used for remission induction and maintenance therapy . In rheumatoid arthritis (RA), systemic GCs at low (≤7.5 mg prednisone equivalent) to medium (>7.5 and ≤30 mg prednisone equivalent) doses provide benefit as an initial treatment, partly through disease-modifying properties . Moreover, intra-articularly applied GC therapy is appropriate in almost all forms of (aseptic) arthritis .
However, due to the pleiotropic actions of GCs, this therapy is associated with the risk of diverse adverse events (AEs), especially at higher doses and longer duration of therapy . The variety of AEs comprises diabetes mellitus/glucose intolerance, hypertension, obesity, osteoporosis, infections and many other complications not mentioned here . Thus, for safety reasons in diseases such as RA, it is recommended to taper off GCs as rapidly as possible . The estimation of the risk of developing GC toxicity is difficult, as in most studies AEs have not been assessed systematically .
This chapter focusses on the chance of creating qualitatively improved new drugs for clinical practice that have the clinically desired efficacy of GCs but reduced or, provocatively, no typical AEs. We will first provide an update on the mechanisms of GC actions and then describe recent developments of new GCs and GC applications. The second part will report on immunostimulatory effects of endogenous GCs and discuss the therapeutic potential of 11β-hydroxysteroid dehydrogenase (HSD) inhibition in diabetes and arthritis. Third, we will present new tools for management of systemic GC therapy and monitoring of AEs, which are especially important for obtaining a true AE profile.
Mechanisms of GC action
Four different mechanisms are considered to mediate the therapeutic effects of GCs: the classic genomic mechanisms caused by activation of cytosolic GC receptors (cGCR); secondary non-genomic mechanisms provoked by the cGCR; non-genomic effects of membrane-bound glucocorticoid receptors (mGCR); and nonspecific, non-genomic effects caused by interactions with cellular membranes, including mitochondrial membranes . New insights have been gained regarding, particularly, the classical genomic actions; these have been recently extensively reviewed in Ref. .
Classical genomic actions are the most important mechanisms of GC action and lead to changes in gene expression. These actions are caused by passage of GC molecules through the plasma membrane, high-affinity binding to the inactive cGCR, formation of the activated GC/cGCR complex and translocation of the complex into the nucleus. In ‘transactivation’ the dimerised GCR–protein complex binds to the promoter of GC-regulated genes, finally leading to up-regulated synthesis of certain regulatory proteins by transcriptional activation. In ‘transrepression’ GCR monomers interfere (‘tethering’) with the activity of (pro-inflammatory) transcription factors such as activator protein 1 (AP-1) and nuclear factor κB (NF-κB), leading to down-regulation of (pro-inflammatory) protein synthesis. In contrast to non-genomic effects, which occur rapidly within seconds or minutes, genomic processes require at least 30 min before significant changes can be observed in regulatory protein concentrations. Usually, it takes hours to days for changes to occur at the cell, tissue or organ level .
Until recently, it was widely believed that the repressive, anti-inflammatory effects of GCs were dependent mainly on ‘transrepression’ , whereas the AEs and metabolic actions of GC therapy were dependent on ‘transactivation’. This view has been somewhat revised recently, with the suggestion that key anti-inflammatory actions of GCs are caused by gene activation . Briefly, this class of genes includes the NF-κB inhibitor IκB and dual specificity phosphatase (DUSP) 1, also known as MKP-1 (mitogen activated protein kinase (MAPK) phosphatase 1), a crucial anti-inflammatory gene, as well as interleukin (IL)-10, a potent immunomodulatory and anti-inflammatory cytokine, GC-induced leucine zipper (GILZ), a protein for which the mechanism of action is unclear but which interacts with, and inhibits the function of, NFκB and AP-1, as well as annexin A1, a calcium-dependent phospholipid binding protein . Observations of the immunomodulatory effects of GILZ in human RA synovial cells, and in an in vivo model of RA, support the hypothesis that GILZ is a key GC-induced regulator of inflammation in RA . Moreover, evidence that the effect of GILZ on bone loss might be due to the opposite of the effect of GCs suggests that manipulation of GILZ can be used in dissociating the beneficial anti-inflammatory effects of GC from their negative metabolic repercussions .
Mechanisms of GC action
Four different mechanisms are considered to mediate the therapeutic effects of GCs: the classic genomic mechanisms caused by activation of cytosolic GC receptors (cGCR); secondary non-genomic mechanisms provoked by the cGCR; non-genomic effects of membrane-bound glucocorticoid receptors (mGCR); and nonspecific, non-genomic effects caused by interactions with cellular membranes, including mitochondrial membranes . New insights have been gained regarding, particularly, the classical genomic actions; these have been recently extensively reviewed in Ref. .
Classical genomic actions are the most important mechanisms of GC action and lead to changes in gene expression. These actions are caused by passage of GC molecules through the plasma membrane, high-affinity binding to the inactive cGCR, formation of the activated GC/cGCR complex and translocation of the complex into the nucleus. In ‘transactivation’ the dimerised GCR–protein complex binds to the promoter of GC-regulated genes, finally leading to up-regulated synthesis of certain regulatory proteins by transcriptional activation. In ‘transrepression’ GCR monomers interfere (‘tethering’) with the activity of (pro-inflammatory) transcription factors such as activator protein 1 (AP-1) and nuclear factor κB (NF-κB), leading to down-regulation of (pro-inflammatory) protein synthesis. In contrast to non-genomic effects, which occur rapidly within seconds or minutes, genomic processes require at least 30 min before significant changes can be observed in regulatory protein concentrations. Usually, it takes hours to days for changes to occur at the cell, tissue or organ level .
Until recently, it was widely believed that the repressive, anti-inflammatory effects of GCs were dependent mainly on ‘transrepression’ , whereas the AEs and metabolic actions of GC therapy were dependent on ‘transactivation’. This view has been somewhat revised recently, with the suggestion that key anti-inflammatory actions of GCs are caused by gene activation . Briefly, this class of genes includes the NF-κB inhibitor IκB and dual specificity phosphatase (DUSP) 1, also known as MKP-1 (mitogen activated protein kinase (MAPK) phosphatase 1), a crucial anti-inflammatory gene, as well as interleukin (IL)-10, a potent immunomodulatory and anti-inflammatory cytokine, GC-induced leucine zipper (GILZ), a protein for which the mechanism of action is unclear but which interacts with, and inhibits the function of, NFκB and AP-1, as well as annexin A1, a calcium-dependent phospholipid binding protein . Observations of the immunomodulatory effects of GILZ in human RA synovial cells, and in an in vivo model of RA, support the hypothesis that GILZ is a key GC-induced regulator of inflammation in RA . Moreover, evidence that the effect of GILZ on bone loss might be due to the opposite of the effect of GCs suggests that manipulation of GILZ can be used in dissociating the beneficial anti-inflammatory effects of GC from their negative metabolic repercussions .
Selective GCR agonists (SEGRAs) or selective GCR modulators (SGRMs)
With this paradigm change, the concept of selective GCR agonists (SEGRAs) was challenged. This concept had promised that addressing dimer-independent function only (i.e., transrepression) almost exclusively would show potent GC therapeutic activity with reduced AEs . The initial animal studies showed good prospects . ZK-245186 (now BOL-303242-X or mapracorat) was demonstrated to show topical anti-inflammatory action in rodent irritant contact dermatitis and T cell-mediated contact allergy models, and a better safety profile including skin atrophy and hyperglycaemia was found . Mapracorat is now tested in topical application also at the eye . However, querying the concept, several studies in a mouse knock-in strain with a dimerisation-deficient GCR (GR dim mice) had shown that GCs in these mice could indeed treat irritant dermatitis and suppress inflammatory regulators, such as tumour necrosis factor (TNF) or matrix metalloproteinase (MMP) −1 and −13 , successfully. Nevertheless, they failed to exert a full anti-inflammatory response in contact allergy, just like endogenous GCs fail in septic shock and in early response during wound healing . Furthermore, in these mice, typical AEs of GC application persisted, such as GC-induced inhibition of bone formation . Therefore, it was suggested that depending on the process that is treated, SEGRAs could be therapeutically more or less effective and not all AEs of GC therapy may be reduced . It seems to be questionable whether SEGRAs will become clinically relevant in rheumatic diseases in the near future or even at all . However, recently it was described for the first time that selective GCR modulators (SGRM), namely the non-steroidal, plant-derived Compound A (CpdA), can act against inflammation, while not impairing osteoblast differentiation . Furthermore, SGRMs, although identified on the basis of impaired transcriptional activation, paradoxically may be capable of inducing expression of DUSP 1 and other genes with anti-inflammatory roles .
mGCRs
Non-genomic effects mediated by membrane-bound receptors (mGCRs) have been reported for steroids and thyroid hormones . We had detected mGCR on human peripheral blood mononuclear cells by high-sensitivity immunofluorescent staining (in small numbers) using an antibody against the cGCR . Relationships between the expression level of mGCR on monocytes and disease activity in RA as well as down-regulation of mGCR by GC treatment in systemic lupus erythematosus were reported .
In further studies, membrane-linked GCRs on human T-cells were demonstrated with rapid inhibition of T-cell receptor signalling by GCs . New data show that reduction of GCR messenger RNA (mRNA) in Hek293 T cells decreased cGCR and mGCR expression, indicating that the human GCR gene encodes for both cGCR and mGCR (Strehl et al., Arthritis Rheum; 2011, in press). Furthermore, dexamethasone-BSA treatment led to induced phosphorylation of multiple kinase substrates including p38 MAPK, demonstrating functional activity of the mGCR (Strehl et al., Arthritis Rheum; 2011, in press).
Clinical relevance of non-genomic effects of GCs has been shown in asthma . In rheumatic diseases, clinical relevance of non-genomic actions at higher doses can be assumed, although it is difficult to prove . We can only speculate whether drugs selectively targeting mGCR would exert strong non-genomic GC actions without evoking the classical (genomic) AEs. For other steroids, membrane receptor targeting drugs are already under pre-clinical development . However, lazaroids (membrane-only active GCs), which have been clinically tested as neuroprotective agents, had to be abandoned because of lack of clinical efficacy .
Long-circulating liposomal GCs
Conventional GCs can be improved by targeted delivery via carrier systems, for example, within long-circulating liposomes, recently extensively reviewed by van den Hoven et al. By this route, GCs accumulate directly at the site of inflammation. The efficacy for liposomes was demonstrated several fold in animal models of RA . Application of liposomes promises therapeutic persistence with a reduction of the typical GC-related AEs . However, potential toxicity issues have not been explored in detail . To date, only one clinical study has been published . No liposomal formulation for the treatment of RA has been marketed yet, nor is any in clinical development now . Therefore, one should be careful about claiming great expectations on the basis of these achievements for the future management of RA .
Combination of GCs with dipyridamole or nitric oxide
Combining GCs with agents that work in synergy can increase the anti-inflammatory activity and could improve the risk/benefit ratio of GCs by reducing the effective dose. GCs have been linked to nitric oxide (NO), which was suggested to enhance anti-inflammatory effects of GCs in animal models, while it is slowly released from these drugs . Recently, the combination of prednisolone and the anti-thrombotic drug dipyridamole was shown to produce anti-inflammatory activity in several animal models, which required only a subtherapeutic dose of prednisolone . However, nitro-GCs and GCs combined with dipyridamole have only been studied in vitro and in animal models; human studies are needed for confirmation.
GC chronotherapy
Currently, the most advanced approach to improve the risk/benefit ratio of GCs, which has already led to a new drug available on the market, is low-dose prednisone chronotherapy with modified release (MR) prednisone . Here, drug release is timed to chronobiological rhythms of RA . MR prednisone has been shown to have greater efficacy for long-term, low-dose GC treatment in patients with RA, with a significant reduction in morning joint stiffness, in addition to all known therapeutic effects with conventional prednisone and a similar safety profile . It has to be established whether treatment with MR prednisone also does have a dose-sparing effect in comparison to conventional GCs, with thereby reduced AEs.
Immunosuppressive versus immunostimulatory effects of GCs
An area of increasing interest is the role of endogenous (physiological) GCs in arthritis and immunomodulation . Endogenous GC hormones are regulated via the hypothalamic–pituitary–adrenal (HPA) axis and, as indicated by the name, mainly control glucose metabolism, and also electrolyte and fluid homeostasis as well as immune and stress responses. Between 1940 and the 1980s, it was thought that an initial cortisol surge serves to stimulate defence mechanisms that included the first attack of the immune system . Thereafter, the pendulum swung to the opposite side, and it was correctly realised that cortisol has also a prominent immunosuppressive role . In recent times, the immunomodulating role of GCs has been redefined to be not exclusively immunosuppressive, because several in vitro and in vivo studies demonstrated immunostimulatory effects of endogenous GCs in human and animal studies . Many of the observations suggest that the effects of GCs follow a dose–response curve with permissive or even immunostimulatory effects at physiologic concentrations of GCs and immunosuppressive effects at pharmacologic concentrations . Besides GC concentration, the timing of GCs administration in relation to immune stimulation is decisive in determining whether GCs exhibit pro- or anti-inflammatory properties . Recent data demonstrate that GC administration 1 h after endotoxin (lipopolysaccharide, LPS) challenge is immunosuppressive, whereas administration of the same GC dose prior to LPS challenge augments immune responses . Prior exposure to GCs also potentiates LPS-induced mechanical allodynia and spinal neuroinflammation . This is in concordance with previous studies, where GCs given 12 or 144 h before LPS administration led to higher cytokine levels compared with LPS alone, whereas GCs given immediately before and concomitantly with LPS attenuated the immune response . Time-dependent effects on T-cell activation were demonstrated, too . In line with previous findings, it has been shown recently that GCs exert opposing effects on macrophage function dependent on their concentration, with enhancement of NO secretion and expression of pro-inflammatory cytokines at low concentrations and repression at high concentrations . Other immunostimulatory effects on cytokine expression had been found, for example, on macrophage migration inhibitory factor (MIF) . GCs stimulate leucocyte redistribution by promotion of the survival and proliferation of neutrophils, by enhancement of the effects of granulocyte macrophage colony-stimulating factor (GM-CSF) and promotion of the shedding of L-selectin from neutrophils . GCs also promote the clearance of foreign antigens, toxins, microorganisms and dead cells by facilitating opsonisation, activation of scavenger systems and stimulation of phagocytotic ability .
GC pre-receptor metabolism
Insights gained in recent years suggest extensive pre-receptor metabolism to be crucial for the action of endogenous GCs, beyond GC plasma concentrations and GCR density . 11β-HSD 1, though being bidirectional, predominantly converts low-active cortisone to the more active cortisol by its reduced nicotinamide adenine dinucleotide phosphate (NADPH)-dependent reductase activity, thereby increasing intracellular GC concentration . 11β-HSD 1 is mainly expressed in the liver, adipose tissue and brain, and expression can be induced in fibroblasts, muscles and immune cells . 11β-Hydroxysteroid dehydrogenase 2 (11β-HSD 2) converts cortisol to cortisone. It is mainly expressed in tissues that also express the mineralocorticoid receptor (especially the kidneys), allowing aldosterone to bind to this receptor . Mutations of the 11β-HSD 2 gene explain inherited forms of hypertension in the syndrome of apparent mineralocorticoid excess .
11β-HSD inhibitors in diabetes mellitus
The phenotypic and metabolic similarities between the metabolic syndrome and Cushing’s syndrome sparked great interest in the therapeutic potential of reduction of cortisol formation in the liver and adipose tissue by inhibition of 11β-HSD 1 . Overexpression of 11β-HSD 1 in adipose tissue is associated with obesity in both humans and rodents and, in transgenic mice additionally causes hypertension and insulin resistance . Indeed, knockout of 11β-HSD 1 in rodents led to reduction of insulin resistance, prevention of stress-induced obesity, improvement of glucose tolerance and enhancement of insulin-secretory responsiveness . Recently, data from a first clinical study with an 11β-HSD 1 inhibitor were presented . Twelve weeks of treatment with NCB13739 (200 mg) added to metformin in patients with type 2 diabetes reduced HbA1c by 0.6%, fasting plasma glucose concentrations by 1.33 mmol l −1 and homeostasis model assessment-insulin resistance by 24% as compared with placebo . Reductions were also noted in concentrations of total cholesterol, low density lipoprotein (LDL) cholesterol and triglycerides in patients with hyperlipidaemia . A potential issue related to the use of these inhibitors is stimulation of the HPA axis, potentially leading to problematic hyperandrogenism in women . In the study, a reversible dose-dependent elevation in adrenocorticotrophic hormone, generally within the normal reference range, was observed. Basal cortisol homeostasis, testosterone in men and free androgen index in women were unchanged . Many other selective inhibitors of 11β-HSD 1 appear to be under development for the treatment of type 2 diabetes and other components of the metabolic syndrome . However, further clinical studies are urgently required . Other possible indications for 11β-HSD 1 inhibitors are treatment of osteoporosis and cognitive decline .
The 11β-HSDs and arthritis
By contrast, the role of endogenous GCs in and their contribution to the susceptibility and severity of arthritis remain inconclusive . It has been shown that pro-inflammatory cytokines are able to modulate intracellular GC metabolism . IL-1β and TNFα stimulate 11β-HSD 1 expression and down-regulate 11β-HSD 2 expression in osteoblasts and fibroblasts . The existence of substantial GC metabolism in the joint was confirmed in primary cultures of synovial fibroblasts isolated from synovial tissue biopsy specimens of RA patients, with a dramatic induction of 11β-HSD 1 activity in synovial fibroblasts by TNFα and IL-1β, strongly correlating with systemic inflammatory parameters . However, the situation is complex, as in RA patients 11β-HSD 2 expression was found in synovial macrophages, normally not expressing 11β-HSD 2 . 11β-HSD 2 was identified as one of the genes most up-regulated in early RA . It will have to be clarified whether this is part of an adaptive response to inflammation or renders the cells insensitive to GCs treatment .
The therapeutic potential of modifying the metabolism of endogenous GCs with regard to inflammation and arthritis is unclear. On the one hand, inhibition of endogenous GCs appears to increase arthritic activity. IL-6 production in synovial-derived fibroblasts from patients with RA was significantly reduced in the presence of 100 mmol l −1 cortisone, being prevented by co-treatment with an 11β-HSD 1 inhibitor . The administration of metyrapone, a 11β-hydroxylase inhibitor reducing adrenal GC synthesis (which can be used for treatment of resistant Cushing’s syndrome) increased disease activity in RA . Moreover, in a model of adjuvant arthritis in rats synovial 11β-HSD 1 up-regulation was reversible by anti-TNF and anti-IL-1β treatment, but, treatment with carbenoxolone, a non-selective inhibitor of 11β-HSD 1, resulted in a significant exacerbation of arthritis and increase of synovial TNFα, cyclooxygenase-2 (COX-2) and osteopontin mRNA expression . On the other hand, inactivation of endogenous GCs in osteoblasts yielded the opposite effect. In a model of K/BxN mouse serum-induced arthritis in mice, we found that selective overexpression of 11β-HSD 2 in osteoblasts significantly reduced the extent of arthritis . These data suggest that osteoblasts modulate the immune-mediated inflammatory response via a GC-dependent pathway, and could point to a therapeutic potential of 11β-HSD 1 inhibition for modulating arthritis by interfering with these immunostimulatory effects. In line with this, preliminary evidence suggested that glycyrrhetinic acid, the biologically active metabolite of glycyrrhizin contained in the roots of liquorice ( Glycyrrhiza spp.), which is a well-known non-selective inhibitor of 11β-HSDs, was able to inhibit adjuvant- and pristane-induced arthritis; however, no results have been published so far . Liquorice is traditionally used by practitioners of alternative medicine to treat individuals with ulcers, bronchitis, cough, allergies, adrenal insufficiency and arthritis Overexposure to liquorice can lead to hypertension . Anti-inflammatory effects of liquorice and roasted liquorice extracts have been demonstrated in murine macrophages as well as in a model of collagen-induced arthritis (CIA) in mice . Recently, a set of 11β-HSD 2-inhibiting glycyrrhetinic acid derivatives has been characterised , extending the spectrum of 11β-HSD-inhibiting drugs. These studies make clear that the role of endogenous GCs in inflammation and bone/cartilage destruction in auto-immune arthritis is very complex and has to be subject to further investigation. However, there appears the possibility of a dual therapeutic potential for 11β-HSD modulation in arthritis/inflammation and concurrent metabolic diseases (see above).
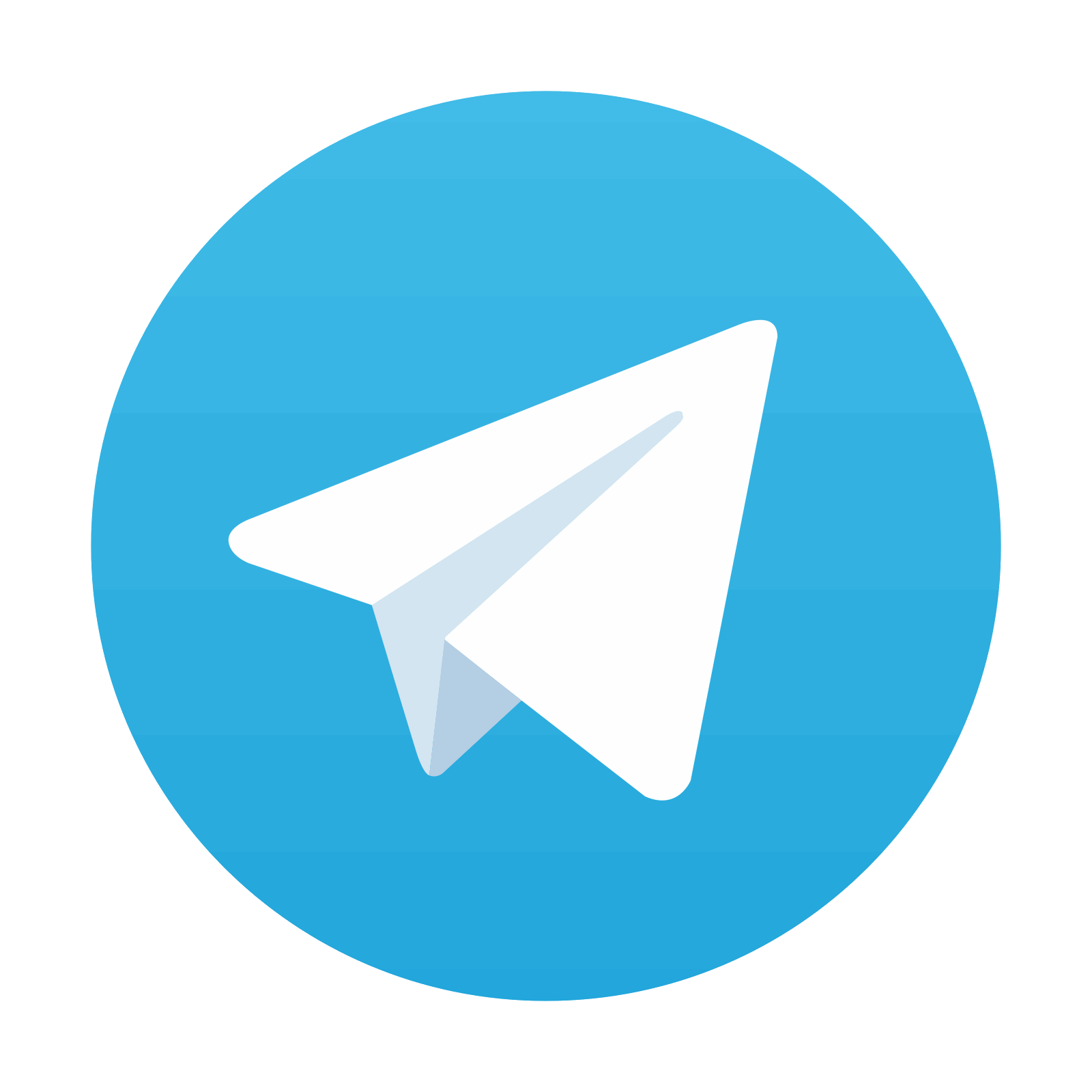
Stay updated, free articles. Join our Telegram channel

Full access? Get Clinical Tree
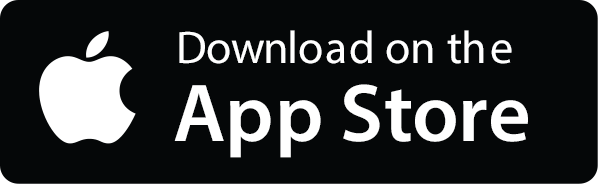
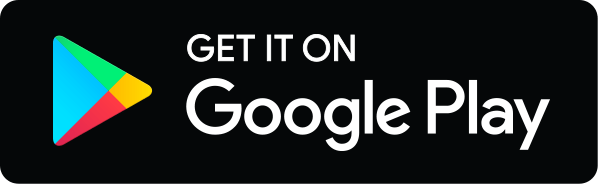