Biomechanical factors play an important role in the health of diarthrodial joints. Altered joint loading – associated to obesity, malalignment, trauma or joint instability – is a critical risk factor for joint degeneration, whereas exercise and weight loss have generally been shown to promote beneficial effects for osteoarthritic joints. The mechanisms by which mechanical stress alters the physiology or pathophysiology of articular cartilage or other joint tissues likely involve complex interactions with genetic and molecular influences, particularly local or systemic inflammation secondary to injury or obesity. Chondrocytes perceive physical signals from their environment using a variety of mechanisms, including ion channels, integrin-mediated connections to the extracellular matrix that involve membrane, cytoskeletal and intracellular deformation. An improved understanding of the biophysical and molecular pathways involved in chondrocyte mechanotransduction can provide insight into the development of novel therapeutic approaches for osteoarthritis.
Introduction
Osteoarthritis is a painful and debilitating disease of the synovial joints, affecting an estimated 12–15% of the population 25–74 years of age . The prevalence of this disease increases significantly with age, with radiographic evidence observed in over 70% of the population over age 65. Considering the extensive impact and consequences of this disease, its aetiology is not fully understood. Indeed, osteoarthritis likely represents a family of diseases that have a similar end point, but with multi-factorial aetiopathogenesis involving genetic, molecular and environmental influences, particularly biomechanical stress. In fact, in many cases, biomechanical factors appear to play a critical role in the events that lead to the initiation and progression of osteoarthritis ( Fig. 1 ).

Osteoarthritis is distinctively characterised by progressive degenerative changes in the morphology, composition and mechanical properties of the articular cartilage, subchondral bone, synovium and other joint tissues. Under normal physiological conditions, the components of the cartilage extracellular matrix (ECM) are in a state of slow turnover, retained in a homeostatic balance between the catabolic and anabolic events of the chondrocytes. These activities are controlled through the processing of both genetic and environmental information, which includes the action of soluble mediators (e.g., growth factors and cytokines), local matrix composition and biophysical factors, including mechanical or osmotic stresses. In particular, the progressive degeneration of articular cartilage indicates that the normal balance of metabolic activities of the chondrocytes has been severely disrupted. Indeed, many studies have shown that the mechanical stress environment of the joint is an important factor that influences (and presumably regulates) the activity of the chondrocytes in vivo . In this regard, knowledge of the role of specific mechanical phenomena in regulating chondrocyte metabolism under inflammatory conditions would provide new insights into the aetiopathogenesis of osteoarthritis. Furthermore, growing evidence indicates that pro-inflammatory cytokines and mediators, and their interactions with mechanical stress may be in part responsible for the catabolic events that occur in osteoarthritic cartilage.
The effects of mechanical loading on cartilage in vivo
Under physiologic conditions, joints of the body are subjected to millions of cycles of loading resulting in forces of up to 10 times body weight passing through the joints. Under normal circumstances, such loads have no adverse effects on the cartilage or other joint tissues, and furthermore, clinical data indicate that a variety of exercises are safe and effective for individuals with osteoarthritis . In fact, exercise for osteoarthritis has been strongly recommended in treatment guidelines by both the American College of Rheumatology and European League Against Rheumatism (EULAR). Furthermore, obesity is potentially the primary risk factor for joint disease that is directly modifiable. In particular, obesity is a strong risk factor for osteoarthritis incidence, progression and disability . While these effects have been assumed to be related to altered gait and increased joint stresses secondary to increased body weight , there is increasing evidence of the presence of systemic inflammation due to obesity that may interact with biomechanical factors to promote the progressive degradation of joint cartilage in other joints such as the hand . The benefits of exercise in an arthritic population include not only weight loss, but also decreased systemic inflammation, increased muscle strength, flexibility, energy, a sense of well-being, better coping abilities, quality of sleep, decreased blood pressure and fewer heart attacks .
Clinical and animal studies of altered joint loading have provided strong evidence that ‘abnormal’ loads can lead to alterations in the composition, structure, metabolism and mechanical properties of articular cartilage and other joint tissues. Abnormal loading may be caused by a variety of factors such as obesity, immobilisation, joint instability, overuse or trauma. For example, obesity is strongly associated with osteoarthritis , and a decrease of 5 kg of body weight has been shown to decrease the risk of osteoarthritis by over 50% . Altered joint loading due to instability or injury of the joint is now well known to be a significant risk factor for the onset and progression of osteoarthritis . Impact loads cause significant damage to the articular cartilage, including splitting of the ECM, increased cellular activity, increased tissue hydration and remodelling of the subchondral bone . These characteristics are generally consistent with the early stages of osteoarthritis, suggesting that hyperphysiologic stresses may be an important factor in the aetiopathogenesis of this disease. Joint instability, induced by ligament transection or meniscectomy , also induce profound and repeatable changes in joint tissues which mimic changes seen in early human osteoarthritis, including increased hydration, collagen disruption and matrix turnover accompanied by decreased tissue stiffness in tension, compression and shear (e.g., Refs. ). Articular cartilage and synovial fluid from these models of osteoarthritis show significant increases in various biomarkers (reviewed in Ref. ) that are correlated with histologic damage in the joint . Inflammatory mediators and cytokines seem to play an important role in these altered loading models of osteoarthritis, although the precise relationships between biomechanical factors and inflammation are not fully understood. Nonetheless, previous studies have shown decreased osteoarthritis severity in animal models through the administration of nitric oxide synthase inhibitors or interleukin-1 receptor antagonists .
Importantly, similar models have been developed and validated in small animals such as mice and rats . In particular, destabilisation of the medial meniscus (termed ‘DMM’ in mice and ‘MMT’ in rats) provides a fairly reproducible model of knee osteoarthritis in mice that progresses to moderate-to-severe levels within weeks post-surgery . The DMM model has sufficient sensitivity to show disease modification, as observed with the ADAMTS-5 knockout mouse , suggesting that instability-induced osteoarthritis could be potentially ameliorated using pharmacologic inhibitors of the aggrecanase pathways.
The effects of mechanical loading on cartilage in vivo
Under physiologic conditions, joints of the body are subjected to millions of cycles of loading resulting in forces of up to 10 times body weight passing through the joints. Under normal circumstances, such loads have no adverse effects on the cartilage or other joint tissues, and furthermore, clinical data indicate that a variety of exercises are safe and effective for individuals with osteoarthritis . In fact, exercise for osteoarthritis has been strongly recommended in treatment guidelines by both the American College of Rheumatology and European League Against Rheumatism (EULAR). Furthermore, obesity is potentially the primary risk factor for joint disease that is directly modifiable. In particular, obesity is a strong risk factor for osteoarthritis incidence, progression and disability . While these effects have been assumed to be related to altered gait and increased joint stresses secondary to increased body weight , there is increasing evidence of the presence of systemic inflammation due to obesity that may interact with biomechanical factors to promote the progressive degradation of joint cartilage in other joints such as the hand . The benefits of exercise in an arthritic population include not only weight loss, but also decreased systemic inflammation, increased muscle strength, flexibility, energy, a sense of well-being, better coping abilities, quality of sleep, decreased blood pressure and fewer heart attacks .
Clinical and animal studies of altered joint loading have provided strong evidence that ‘abnormal’ loads can lead to alterations in the composition, structure, metabolism and mechanical properties of articular cartilage and other joint tissues. Abnormal loading may be caused by a variety of factors such as obesity, immobilisation, joint instability, overuse or trauma. For example, obesity is strongly associated with osteoarthritis , and a decrease of 5 kg of body weight has been shown to decrease the risk of osteoarthritis by over 50% . Altered joint loading due to instability or injury of the joint is now well known to be a significant risk factor for the onset and progression of osteoarthritis . Impact loads cause significant damage to the articular cartilage, including splitting of the ECM, increased cellular activity, increased tissue hydration and remodelling of the subchondral bone . These characteristics are generally consistent with the early stages of osteoarthritis, suggesting that hyperphysiologic stresses may be an important factor in the aetiopathogenesis of this disease. Joint instability, induced by ligament transection or meniscectomy , also induce profound and repeatable changes in joint tissues which mimic changes seen in early human osteoarthritis, including increased hydration, collagen disruption and matrix turnover accompanied by decreased tissue stiffness in tension, compression and shear (e.g., Refs. ). Articular cartilage and synovial fluid from these models of osteoarthritis show significant increases in various biomarkers (reviewed in Ref. ) that are correlated with histologic damage in the joint . Inflammatory mediators and cytokines seem to play an important role in these altered loading models of osteoarthritis, although the precise relationships between biomechanical factors and inflammation are not fully understood. Nonetheless, previous studies have shown decreased osteoarthritis severity in animal models through the administration of nitric oxide synthase inhibitors or interleukin-1 receptor antagonists .
Importantly, similar models have been developed and validated in small animals such as mice and rats . In particular, destabilisation of the medial meniscus (termed ‘DMM’ in mice and ‘MMT’ in rats) provides a fairly reproducible model of knee osteoarthritis in mice that progresses to moderate-to-severe levels within weeks post-surgery . The DMM model has sufficient sensitivity to show disease modification, as observed with the ADAMTS-5 knockout mouse , suggesting that instability-induced osteoarthritis could be potentially ameliorated using pharmacologic inhibitors of the aggrecanase pathways.
Obesity, mechanical loading and osteoarthritis
Obesity (or factors associated with obesity) is a primary risk factor for osteoarthritis . Within the past two decades, the prevalence of obesity has risen dramatically such that two-thirds of adults in the United States are now overweight or obese , with similar increases in the prevalence of obesity in Europe and worldwide . Significant evidence shows that obesity alters gait and joint biomechanics, although not necessarily to increase the overall magnitude of joint loads or torques . A systematic review of this topic showed that at self-selected speed, obese individuals walk slower, with shorter and wider steps, and have longer stance durations compared with normal-weight individuals . While these altered biomechanics can affect the load-bearing regions of the articular cartilage, the role of such changes as risks factors for osteoarthritis is not fully understood.
The effects of obesity on the joint historically have been dismissed as simply increased ‘wear-and-tear’; however, there is increasing evidence of multi-factorial, systemic links between obesity and osteoarthritis . For example, only a small reduction in body weight (5 kg), and in particular body fat (2.4%), is associated with significant improvements in lowering the risk or progression of osteoarthritis . Furthermore, obesity is associated with increased osteoarthritis of the hand , which is not a ‘weight-bearing’ joint per se .
Obesity, biomechanics and inflammation
In addition to alterations in joint mechanics, obesity has been associated with a state of low-grade chronic systemic inflammation . Adipose tissue is a source of local and systemic inflammation and excess adiposity has been implicated in a number of diseases, such as type 2 diabetes, cardiovascular disease and cancer . A number of studies have shown that pro-inflammatory cytokines associated with adipose tissue (i.e., adipokines), such as interleukin-1 (IL-1), IL-6, tumour necrosis factor alpha (TNF-α), leptin, adiponectin, etc., have a strong influence on cartilage biology . These adipose-derived cytokines, or ‘adipokines’, are believed to play important roles in a number of diseases related to the metabolic syndrome. In obese but otherwise healthy women, weight loss of 3 kg due to dietary restriction causes a marked decrease in serum IL-6 levels . In fact, IL-6 has been proposed as a critical factor in the link between inflammation, obesity, stress and coronary heart disease . In addition, psychosocial stressors have been shown to further increase plasma IL-6 levels . These intriguing findings suggest that the link between obesity and osteoarthritis may not be solely biomechanical, but may in fact reflect the influence of biomechanical, metabolic and psychosocial factors on the joint in the presence of systemic inflammation.
While obesity is clearly a risk factor for clinical osteoarthritis, only a few animal models have been reported for studying the relationship between obesity and osteoarthritis (reviewed in Ref. ). These generally have involved rodent models that are either diet induced with high-fat feeding or are overweight/obese inbred strains that spontaneously develop osteoarthritis. High-fat diet-induced obese mouse models, especially the C57BL/6J strain, have been extensively studied for their utility as animal models of human obesity . When fed a low-fat diet, C57BL/6J mice remain lean and display normal insulin levels and blood pressure. However, when fed a high-fat diet, C57BL/6J mice develop central adiposity, hyperinsulinemia, hyperglycemia and hypertension – many of the features of human obesity and metabolic syndrome . C57BL/6J mice fed a high-fat diet also develop osteoarthritis-like changes at an earlier age and to a more severe degree than C57BL/6J mice fed a lower-fat control diet . Within these models, the incidence of osteoarthritis is similar between males and females , and lard-based fat supplements induce osteoarthritis more severely than vegetable-based fat supplements . High-fat feeding also induces osteoarthritis in rats . Two inbred strains of spontaneous osteoarthritis – the STR/Ort mouse and the Hartley guinea pig – are heavy compared to other mouse and guinea pig strains, respectively, and therefore may be considered obese animal models of osteoarthritis . Interestingly, a recent study has shown via quantitative trait analyses that STR/ort mice possess three chromosomal locations associated with body weight or fatty acid metabolism . In other studies, body weight restriction in the Hartley guinea pig by limiting food greatly reduced the severity of osteoarthritis in the knee joints .
These findings suggest that in these animal models of osteoarthritis, body weight and/or body fat is a mediating factor in the development of spontaneous osteoarthritis of the knee. However, recent studies in obese mice have shown that leptin-impaired mice, which become morbidly obese, do not exhibit knee osteoarthritis . Furthermore, mice fed an extremely high-fat diet (60% fat by kcal) exhibit knee osteoarthritis that is partially ameliorated by exercise, in the absence of any weight loss . These findings suggest that adiposity (or weight), in and of itself, may not be a risk factor for joint degeneration, but rather, that interactions among joint loading, diet and local or systemic inflammation may be responsible for the initiation and progression of osteoarthritis .
Overall, these in vivo studies emphasise the relationship between mechanical loading and the health of the joint, and suggest that the normal mechanical regulation of chondrocyte activity may be detrimentally influenced by the presence of pro-inflammatory mediators and cytokines in the joint. Taken together, they suggest that a critical level and manner of joint loading is required to regulate the normal homeostatic balance of cartilage anabolism and catabolism. The interactions of biomechanical and inflammatory mechanisms involved in the progression of cartilage degeneration are not known. Currently, there is significant evidence to implicate the role of various cytokines, specifically IL-1, leptin and TNF-α, in these processes . Taken together, the collective disuse and exercise studies suggest that degenerative changes are associated with hyperphysiologic magnitudes of loading or by alterations in the normal loading pattern of the joint (e.g., loading of normally unloaded regions of cartilage).
In vitro studies of the effects of mechanical loading on cartilage metabolism
In vitro explant models of mechanical loading provide systems in which the biomechanical and biochemical environments of the chondrocytes can be better controlled as compared to the in vivo situation. Considerable research effort has been directed towards understanding the processes by which biophysical signals are converted to a biochemical signal by the chondrocyte population. Clarification of the specific biophysical factors and biological signalling mechanisms in normal and inflamed cartilage would provide a better understanding of cartilage physiology and may also yield new insights on the pathogenesis of osteoarthritis. This information could potentially reveal new targets for the prevention or treatment of disease.
Explant models of cartilage loading have been used in a number of different loading configurations, including unconfined compression, indentation, tension and osmotic and hydrostatic pressure. The general consensus of these studies is that static compression suppresses matrix biosynthesis, and cyclic and intermittent loading stimulate chondrocyte metabolism (e.g. Refs. ,). These responses have been reported over a wide range of loading magnitudes, and exhibit a stress-dose dependency (reviewed in Ref. ). Excessive loading (e.g., high magnitude, long duration) seems to have a deleterious effect, resulting in cell death, tissue disruption and swelling . Injurious compression (high-loading rate) can cause damage to the collagen fibril network and increased tissue swelling . Increased proteoglycan release and nitric oxide (NO) production were also observed, suggesting that mechanical stress alone can stimulate cell death as well as a range of biomechanical and biochemical alterations to the matrix. Interestingly, mechanical compression can induce a dose-dependent increase in the production and release of a variety of osteoarthritis biomarkers, including proteoglycan epitopes such as 3B3, 5D4 and cartilage oligomeric matrix protein .
Importantly, mechanical stress has been shown to influence directly the inflammatory cascade of chondrocytes to induce or inhibit the production of pro-inflammatory mediators such as NO or prostaglandin E2 (PGE 2 ), depending on culture configuration and specific loading regimen. For example, chondrocytes in monolayer exhibit increased production of NO in a manner that can be inhibited by hydrostatic pressure , whereas cyclic stretch in monolayer can produce an anti-inflammatory response against the effects of IL-1 . Mechanical compression of cartilage explants induces production of NO and PGE 2 , although chondrocytes embedded in an agarose matrix show an opposite effect suggesting that interactions with the native ECM may influence this response.
Mechanisms of mechanical signal transduction in cartilage
In response to loading of the cartilage ECM, chondrocytes are exposed to a diverse array of biophysical signals that vary with time and location in the tissue, consisting of stress, strain, fluid flow, fluid pressure, electrokinetic effects and changes in the tissue-fixed charge density, in addition to changes in the shape and volume of the chondrocytes . Determining the relative contribution of each factor has been difficult, but several studies have pursued a ‘reductionist’ approach in an attempt to determine the biophysical mechanisms involved in the chondrocytes’ response to mechanical stress . There is growing evidence that chondrocytes sense these physical signals through the integrated actions of ion channels, integrin-mediated connections to the ECM and intracellular or membrane deformation . In this regard, the pericellular matrix, which completely encapsulates all chondrocytes, is likely to serve as a transducer of the physical signals in the cells’ environment . Thus, mechanical alterations that occur in the microenvironment of the chondrocytes with aging or degeneration may further alter the cells’ response to loading .
Furthermore, the cartilage ECM is inherently negatively charged due to the large concentration of the anionic proteoglycan aggrecan that attracts cations to counterbalance the fixed charge. While the tissue components are intrinsically incompressible , compression of cartilage results in pressurisation and exudation of the interstitial water, increasing the apparent concentration of proteoglycans, and thus the local fixed charge density . Thus, chondrocytes can experience fluctuations in extracellular osmolarity, resulting in the activation of intracellular signalling cascades and acute volume change, followed by active volume regulation, which involves cytoskeletal F-actin restructuring as well as solute transport and extracellular Ca ++ influx, which is amplified by release from intracellular stores . One novel potential candidate, involved in chondrocyte osmo-mechanotransduction, is the Ca ++ -permeable, non-specific cation channel TRPV4 of the vanilloid subfamily of transient receptor potential (TRP) channels, which has recently been shown to control the mechano-osmotic transduction cascade in chondrocytes . An understanding of the precise sequence of events by which mechanical signals are converted to intracellular signals will hopefully provide new insights into the development of pharmacologic and physical therapies that can modify the course of osteoarthritis.
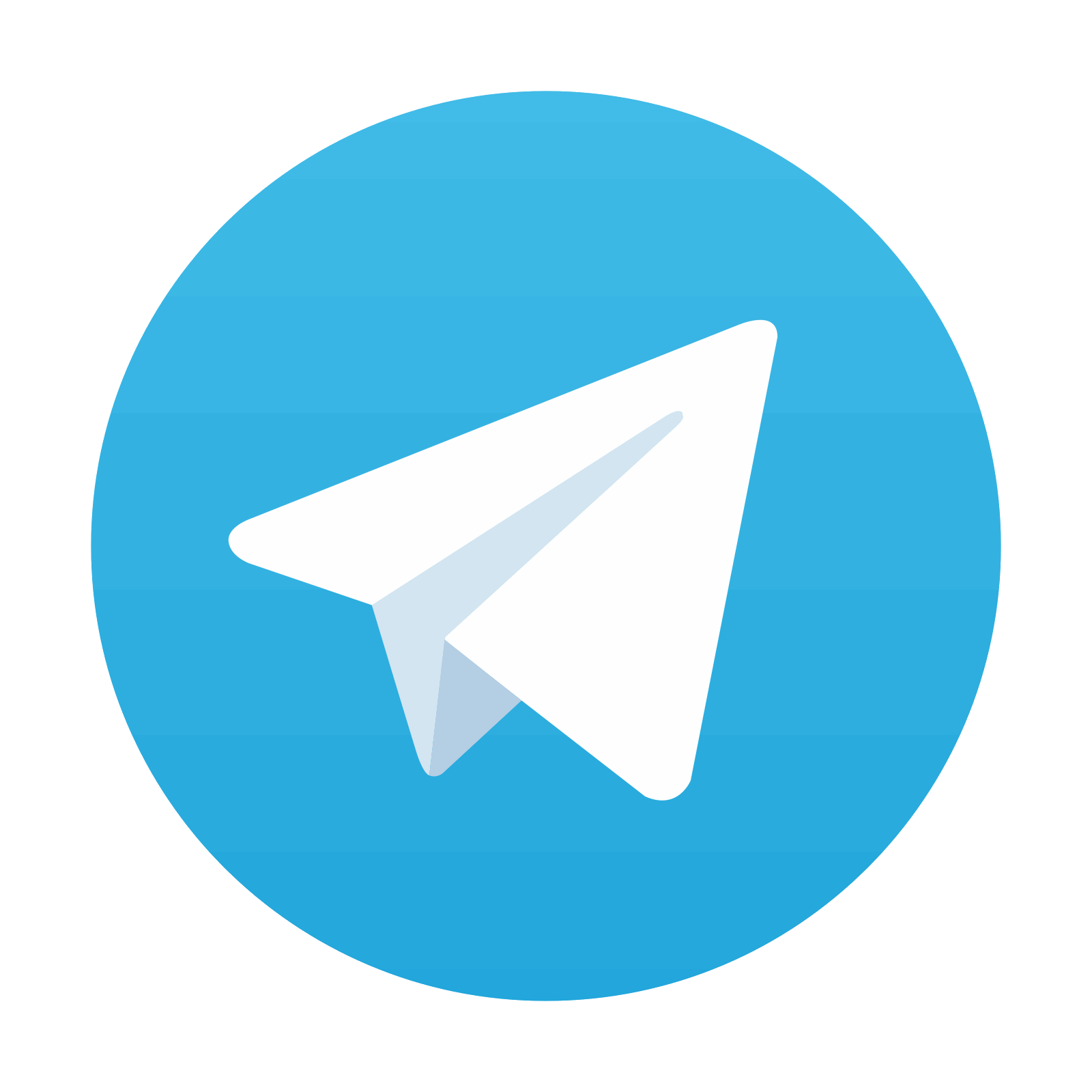
Stay updated, free articles. Join our Telegram channel

Full access? Get Clinical Tree
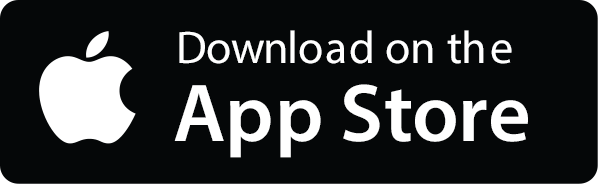
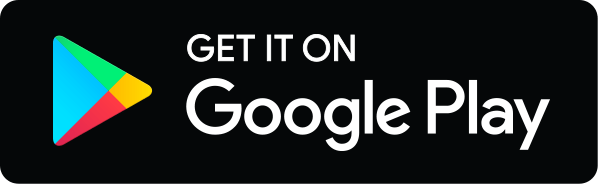