Chapter 53 Functional Toxicology
Introduction
The wide array of persistent toxicants in our environment has undoubtedly caused or contributed to a great diversity of health threats. Prominent biologists Colborn and Myers1 documented the precarious state of the human as a species in the best-selling book Our Stolen Future. Although the environment–cancer connection has been acknowledged for many years, this book documented the increasing evidence that chemical pollutants pose a significant threat to our fertility, our intelligence, and many other aspects of health as well. Furthermore, the scientific literature is beginning to reflect an increasing awareness that polysymptomatic disorders such as multiple chemical sensitivity syndrome, sick building syndrome, chronic fatigue syndrome, and the Gulf War syndrome are all strongly associated with toxic chemical exposures.2,3 Recently, the Collaborative on Health and the Environment formed an initiative to bring together researchers, clinicians, government officials, environmental activists, educators, and others with common concerns about the impact of the environment on human health. This initiative, with over 6000 individual and organizational members, is becoming a catalyst to raise awareness of the growing evidence linking environmental chemical influences to a wide array of human health conditions.4 This organization maintains a database of chemicals and related health conditions. It has also established numerous working groups to bring together researchers and other professionals for focusing on the relationship between environment and specific health conditions, along with solutions to address these issues.
Humans reside on the top of the food chain and are subject to the phenomenon of bioconcentration or biomagnification, as are other species near or at the top of the food chain.5 Additionally, long lives, occupational exposures, household chemicals, prescription drugs, and dental amalgams are some of the many reasons why humans are in a class of their own for magnitude and diversity of chemical exposure.
Chemical threats to human health exist in both organic and inorganic forms. The actual number of xenobiotic chemicals that are encountered on a daily basis is vast. It is becoming clear that few, if any, of these toxicants act alone but, instead, generally act in synergy with other chemicals.6 Chemical synergy can increase a chemical’s toxicity by several hundredfold, resulting in “superinteractions” far exceeding even those of an additive nature.7 Synergistic chemical reactions may occur even before the toxicants enter the human body, changing the chemical’s nature and complicating their toxicologic evaluation even further.8 In addition, many toxins are now known to exert toxic effects at levels vastly lower than originally assumed. For example, cadmium was shown to have estrogen-mimicking effects in tissue at a level of 100,000th of a part per billion.9
Common Toxins That Affect Human Health
Organohalogens
Organohalogens are chemicals that are generally highly persistent in the natural environment, as well as the human body. The major categories of organohalogens are the organochlorine pesticides, polychlorinated biphenyls (PCBs), polybrominated diphenyl ethers (PBDEs), plastic residues, dioxins, and organic byproducts of water chlorination. All of these agents are subject to bioaccumulation in the food chain and are highly persistent within the human body.10,11
One of the first man-made chemicals to be recognized as a persistent contaminant of the food chain with a distinct presence in human tissues was the organochlorine dichlorodiphenyltrichloroethane (DDT). Several other organochlorine pesticides, as well as the family of PCBs and fire retardants (PBDEs), have been established to be highly persistent and widely distributed as human contaminants. These agents were introduced and strongly promoted, in part, because they have minimal short-term toxicity. However, they have been established to be highly disruptive to the endocrine system and have been linked to problems such as breast cancer, infertility, and premature onset of puberty.12,13 More subtle effects of endocrine-disrupting organohalogens, particularly effects on behavior and neuropsychological function, have been well described.14,15 The ubiquitous and highly persistent nature of this group of chemicals makes them of grave concern to human health.
Organophosphates
In the 1930s, Nazi scientists synthesized the organophosphate nerve agents Tabun and Sarin while looking for more effective pesticides. Although Sarin and Tabun are now classified as weapons of mass destruction and are officially banned from most nations, they formed the prototypes for the currently used organophosphate pesticides. Pesticides in use today are acutely less toxic than their parent chemical warfare agents. However, they use the same basic mechanism for exerting toxicity, and they have no known safe dose at which no neurologic effects occur. Although their half-lives are short in the environment, because they are so ubiquitous in our diets and used so frequently in homes, on pets, and in the environment, they pose a significant risk to humans.16 Symptoms of long-term organophosphate pesticide toxicity can be subtle and cumulative. Mental and emotional dysfunction may develop so gradually that it can go unnoticed for years, or it may be attributed to aging.17
Organic Solvents
Numerous solvents are used occupationally and in the home for a multitude of purposes. Benzene, toluene, and various petrochemical solvents (e.g., gasoline) are all significantly neurotoxic in long-term, low-level exposure, and are of particular concern for pregnant women and children.18,19 Solvents have also been shown to carry significant carcinogenic potential.20
Heavy Metals
Except for the past 100 years, toxic metals were virtually absent from the general population. Adaptive physiologic mechanisms are phylogenetically unprepared for more than small trace quantities of toxic metals. Metals are not biodegradable and thus accumulate in the environment and enter the food chain. The recognition of heavy metal toxicity as a primary cause of chronic illness may be impaired by the vast economic importance of metals to the economy of all nations. The 30 years of intense fighting between scientists and industrial lobbyists over lead in gasoline illustrates the effort required to bring about changes in public health policy when it comes to metals.21 The hot debate over mercury in dental amalgams is becoming an even more intense war of vested interests.22 The reclassification of sewage sludge from a toxic waste to an acceptable source of nitrogen for fertilizers is an example of how money drives unsustainable environmental practices that are leading to a rapid accumulation of toxic metals in the environment.23 Because of their ubiquitous distribution and their well-documented toxicity, lead, mercury, arsenic, aluminum, and cadmium are of particular importance to the general population. The discussion of toxic metals in this chapter is limited to lead and mercury.
Lead
Lead has been known to affect workers for at least 1000 years, and its hazards to the reproductive process have been known for at least a century. Childhood lead poisoning was first described in Australia 100 years ago, and for 50 years it was believed that if lead did not kill the child, he or she would be not affected by any residual problems.16 However, careful follow-up of children who recovered from lead poisoning showed that most failed in school and had behavioral problems. More recent evidence confirmed that subclinical levels of lead cause measurable deficits in neuropsychological function.
Despite reduced use of lead-based paints and gasoline, lead continues to be a worldwide public health hazard. The marked effects of lead toxicity on the central nervous system (CNS)—lowering IQ and impairing memory, reaction time, and the ability to concentrate—are well known.24,25
Lead is also a significant health threat to adults. Several studies found that the bones of modern humans contain lead on the order of 1000 times greater than bones of preindustrial humans.26 Apart from its effect on the brain, lead affects the function of the kidneys and the cardiovascular system.27 Lead, at low levels, may act in synergy with other common toxicants to subtly suppress the immune system and impair health.28
Mercury
Mercury is one of the most toxic of all elements, far more toxic than lead.29 Mercury is the deadliest and most pervasive pollutant in aquatic ecosystems, and its presence is steadily rising in most regions throughout North America, primarily due to coal burning power plants and mining activities. Humans are exposed to mercury through consumption of fish (methylmercury), occupation (ionic mercury and mercury vapor), vaccinations (ethylmercury), and dental amalgams (mercury vapor). Except for populations that consume large amounts of seafood or workers in industries where mercury exists as an occupational hazard, the majority of the population is exposed to mercury through dental amalgams.30
The basic premise for regarding the amalgam filling as safe was the assumption that the amalgamation process resulted in a stabilization of the normally volatile mercury. This premise has since been proven entirely false. Since the 1980s, it has been well established that mercury vapor is continuously released from amalgam fillings. The release of this vapor into the mouth increases immediately after chewing31 or tooth brushing32 and can result in a daily absorbed dose of mercury that exceeds the excretory capacity via the urine and stool. It has now been well established and published by several authorities, including the World Health Organization (WHO), that amalgam tooth fillings are, by far, the major source of mercury exposure for the general population.30 According to the WHO’s expert committee, the daily human exposure to mercury vapor from amalgam fillings ranges from 3 to 17 mg, compared with a maximum of 2.6 mg from all other sources.
Mercury vapor released from dental amalgams is efficiently absorbed through the alveoli. After absorption through the lungs, elemental mercury vapor (Hg0) is only found transiently in the blood. Due to its high lipid solubility, elemental mercury is rapidly transported through cell membranes (including membranes of the cells comprising the blood–brain barrier). Once inside metabolically active cells, Hg0 is then oxidized by catalase to form ionic mercury (Hg2+). Hg2+ is not lipid soluble and therefore results in a high degree of retention of absorbed mercury and a tissue half-life ranging from days to decades depending on the particular organ.33,34
This phenomenon clarifies why studies repeatedly demonstrated that after placement of amalgam fillings, blood, hair, and urinary mercury levels remain relatively low, although many organs develop concentrations of mercury many times greater than that of the blood.35,36 Thus, blood, hair, or nonchallenged urinary mercury levels bear little relationship to the total body burden of mercury gradually acquired from amalgam fillings.
Once mercury enters the cell, it ultimately becomes bound covalently to the sulfhydryl groups found in glutathione and, to a lesser degree, to cysteine, biotin, lipoic acid, and coenzyme A, as well as to other protein sulfhydryl groups. The major intracellular sulfhydryl compound in mammals is the tripeptide glutathione. Glutathione and the enzyme glutathione peroxidase are probably the most important antioxidant defenses in humans.37 Mercury was shown to cause a marked reduction in glutathione production and glutathione peroxidase activity, and thus it might result in a marked rise in oxidative stress within the brain and other organs. Apart from the loss of antioxidant protection from mercury-induced inhibition of glutathione and glutathione peroxidase, mercury results in a marked increase in free radical generation through Fenton reactions and other mechanisms.38
In addition to its key role in antioxidant defenses, glutathione is also a critical component in the liver’s detoxification mechanisms. Enzymes within the liver must form conjugates between glutathione and certain toxic metabolites, organic xenobiotics, and heavy metals to enable these toxins to be eliminated from the body. This process of glutathione conjugation makes toxic molecules more water soluble and enables their excretion via bile or through the kidney. If liver glutathione production is markedly inhibited, as occurs when mercury accumulates within hepatocytes, mercury and numerous other toxic substances may more readily accumulate throughout the body because the excretion of such substances is significantly impaired.39 Furthermore, because most mercury is excreted through the stool and urine as a glutathione conjugate, individuals with long-standing body burdens of mercury (and thus depleted glutathione production) may not demonstrate elevated levels of mercury in the urine, blood, or stool when specimens are gathered in the absence of a challenge with an appropriate metal chelating agent. Thus, tissue biopsy of target organs or a provocation test measuring urinary mercury after the administration of a chelating agent may currently be the only valid means to assess long-term mercury body burden.40
Numerous studies demonstrated the body tissue uptake and distribution of mercury from dental amalgams. Studies using whole body imaging in primates with dental amalgams clearly demonstrated that the amalgams resulted in high levels of mercury in the kidney, intestinal tract, brain, liver, and other organs.41
The impact of long-term, low-level mercury exposure is now known to adversely affect numerous other cellular and organ system processes. Ionic mercury may contribute significantly to autoimmune processes.42 Of particular concern to the natural medicine practitioner is the impact that low levels of inorganic mercury have on gut flora. Research demonstrated that multiple strains of antibiotic-resistant bacteria developed rapidly in the gut and oral cavity of both humans as well as nonhuman primates after placement of amalgam fillings.43 Furthermore, subclinical levels of inorganic mercury were shown to markedly impair the immune system’s ability to suppress the growth of Candida albicans.44
Amalgam fillings were shown to contribute to mercury accumulation in human and animal kidneys, and this was associated with a significant decrease in renal function.45 Human fertility was also shown to be significantly affected by low-level exposure to mercury vapor. A recent study that examined 7000 dental assistants demonstrated that this group experienced a fertility rate approximately 40% less than that of women who had no occupational exposure to mercury.46
Of perhaps greatest concern is the potential role of low-level, long-term mercury exposure on CNS function. It is now well established that amalgam-derived mercury accumulates in human brain tissues.47 Mercury was shown to concentrate selectively in human brain regions involved with memory function, and it might play a significant role in the etiology of Alzheimer’s disease.48 Other reports showed subclinical motor and neuropsychological deficits among dentists and dental workers compared with control subjects.49 Mounting evidence led some researchers to suggest that mercury from amalgams might play a highly significant role in the etiology of numerous mental illnesses and neuropsychological disorders (Table 53-1).50–52
TABLE 53-1 Examples of Toxic Metal Contaminants in Humans
TOXIC METAL | SOME COMMON SOURCES | EFFECTS OF LOW-LEVEL CHRONIC ACCUMULATION |
---|---|---|
Aluminum | Drinking water, antiperspirants, antiperspirant crystals, antacids, baking powder, bentonite | Immune suppression, cognitive dysfunction, dementia |
Arsenic | Preserved wood (playgrounds and garden borders), drinking water, pesticides, fish | Gastrointestinal irritation, fatigue, cardiovascular damage, peripheral nerve damage, skin changes, cancer |
Cadmium | Cigarette smoke, welding or grinding, air pollution, food from contaminated soils, chocolate | Kidney damage, cardiovascular damage, cognitive impairment |
Lead | Old paint, lead solder water pipes, contaminated soil, car batteries, sewage sludge, chocolate, popular calcium supplements | Brain and nervous system damage, decreased intelligence, high blood pressure, cardiovascular disease |
Mercury | Dental amalgam fillings, freshwater fish and larger predatory ocean fish, vaccines (thimerosal preservative), broken thermometers, spilled liquid mercury | Depression, anxiety, intellectual impairment, severe fatigue, chronic pain, immune suppression, autoimmune disorders, dementia, peripheral neuropathies |
Mechanisms of Toxic Injury
Targets of Toxicity
Xenobiotic-initiated injury may be largely described as affecting three possible targets53:
1. Toxicant agents may alter, remove, or impair the synthesis of specific molecules (e.g., phospholipids, fatty acids, proteins, nucleotides, or glutathione).
2. Toxicants may alter structural entities (e.g., mitochondria, cytoskeleton, plasma membrane, or nucleus).
3. Toxicants may create disturbances in cell signaling (e.g., cytokines, eicosanoids, hormones, calcium channels, or neurotransmitters).
Biotransformation
Most drugs, metals, and xenobiotics undergo biotransformation before being excreted through the stool, urine, or sweat. Although not every molecule is processed in this way, biotransformation typically involves phase 1 and phase 2 modifications in the liver. Most phase 1 modifications involve oxidation, but can involve demethylation, hydroxylation, or dehalogenation. Phase 2 modifications result in conjugation of xenobiotic or metal ions with a carrier molecule, such as glutathione, cysteine, sulfate, or glycine. The cytochrome P450 enzymes are essential molecules involved in the metabolism of drugs, foreign chemicals, arachidonic acid, cholesterol, steroids, and other important lipids. However, xenobiotic biotransformation by cytochrome P450 mixed function oxidases is a two-edged sword.54 On one hand, many xenobiotics are highly toxic when entering the body, and phase 1 oxidation can change the “molecular identity” of the molecule, rendering it less toxic (phase 1 detoxification).55 Also, P450 oxidation can render a xenobiotic more water soluble (more readily excreted and less prone to penetrate cell membranes). Because a significant proportion of xenobiotics are lipid soluble, they readily pass through cell membranes (and the blood–brain barrier) and may accumulate in fatty tissues, causing prolonged toxic effects. Thus, adequate activity of phase 1 liver detoxification is critical in protecting humans from toxic stress.
However, biotransformation by cytochrome P450 enzymes may play a significant role in establishing or increasing the toxic nature of a xenobiotic. Cytochrome P450 enzymes oxidize the xenobiotic entity and transform it into a reactive electrophile. This reactive intermediate, if not immediately transformed further by one of several conjugating enzymes (phase 2 detoxification), may exert toxic effects within the liver, or systemically, if it escapes from the hepatocyte. These effects may include one or more of the following reactions53:
1. Covalent binding of the reactive metabolite to:
2. Creating oxidative stress by one of three mechanisms:
Hormone Disruption
The homeodynamic balance of virtually any of the body’s hormonal systems may be disrupted through toxic stress. Such disruptions may occur through effects upon the hypothalamic, pituitary, endocrine gland axis or peripherally on hormone production or hormone receptors. The estrogenic effect of environmental agents has been a major focus in toxicology research.56 It has been recognized for some time that a number of chemicals in the environment possess estrogenic activity in various biological systems. These compounds include plant and fungal products, pesticides, plasticizers, estrogenic agents administered to livestock, and various other chemicals. It was originally postulated that estrogenic agents interacted with the estrogen receptor in an “on–off switch” fashion. This is now considered to be overly simplistic or even incorrect.57
Different estrogenic agents bind to a wide variety of estrogen receptors and create hormone-receptor complexes with variable life spans and molecular conformation. The hormone-receptor complex then binds to one of several dozen nucleotide estrogen response elements. This trimolecular complex binds to various target genes in the nucleus and either activates or suppresses transcription. Depending on the “flavor” of the hormone-receptor-estrogen response element complex, a wide variety of specific hormonally mediated effects may occur. This phenomenon helps to explain how certain estrogenic agents can be carcinogenic (organochlorine pesticides), whereas others can be anticarcinogenic (phytoestrogens). The wide variety of responses of different tissues to estrogenic influences also helps to understand how some agents, for example, affect the risk of cancer, whereas others affect behavior or sexual responsiveness.58
Chemical contaminants are now known to play a significant role in the etiology of obesity. Hormone disruption can impact adipogenesis, insulin sensitivity, mood and appetite, and may be one of the most significant influences behind the obesity and diabetes epidemics.59 Particularly surprising is the very strong dose–response relationship that has been seen between concentrations of persistent organic pollutants and diabetes.60
Toxicity of Heavy Metals
Toxic metals share many of the deleterious effects of organic xenobiotics, particularly organically bound metals (e.g., methylmercury, tetraethyl lead, and methylcyclopentadienyl manganese tricarbonyl). However, toxic metals have some peculiarities not exhibited by organic xenobiotics. For example, toxic metals act as “chemical competitors” with chemically and physically similar nutritional trace metals (e.g., cadmium competes with zinc, lead competes with calcium, mercury competes with selenium).61 This phenomenon may result in high rates of loss of the competitive nutrient metal or, alternatively, inhibition (or overactivation) of metal-dependent physiologic systems such as enzymes.
Metal-Catalyzed Oxidative Injury
In addition, non-nutrient metals may bind to molecular regions where they carry out inappropriate catalytic activities, including the generation of free radicals.38,62 The resulting oxidative damage to high-energy membrane pumps and other transport mechanisms required for the homeodynamic regulation of trace mineral and electrolyte balance may then exacerbate nutrient mineral loss even further. If membrane pumps are damaged, sharp concentration gradients of minerals and electrolytes can become blunted, leading to marked changes in the regulatory activity of the cell.53 For example, cytosolic levels of calcium are normally 1/10,000th the concentration found outside the cell or inside the endoplasmic reticulum or mitochondria. Oxidative injury to membrane pumps or calcium channels can result in a loss of this steep gradient. This rise in cytosolic calcium can result in overactivation of calcium-dependent systems, such as the calmodulin family of enzymes. This may, in turn, result in various events, such as hypertonia of skeletal muscle or activation of membrane phospholipases with subsequent degradation of membrane phospholipids and increased inflammation.
Mitochondrial Disruption
Toxic metals and a wide variety of xenobiotics also target the membranes of the mitochondria and result in marked alterations of energy metabolism.63 Toxic mitochondrial damage may lead to rapid cellular aging, cell death, or intracellular acidosis. Paradoxically, intracellular acidosis may then place the cell into a partially “hibernative” state in which it is protected from lethal injury.64 This may help to explain the high degree of functional impairment and the minimal risk of lethality in patients with conditions such as chronic fatigue syndrome or fibromyalgia. In this model, toxic mitochondrial injury results in energy depletion, clinical exhaustion, and evidence of intracellular acidosis.
Biochemical Individuality and Toxic Response
Detoxifying Enzymes and Genetic Polymorphism
A significant amount of cumulative data demonstrates that widespread genetic polymorphism for the expression of cytochrome P450 enzymes exists within the human species.65 The significance of this phenomenon has not been completely described, but several studies suggested a strong correlation between genetic deficiency of certain cytochrome P450 enzymes and susceptibility to environmentally mediated disorders such as Parkinson’s disease66 and lung cancer.67 In addition, individual variation in drug or xenobiotic metabolism due to genetic variation has been extensively investigated. Population studies showed a wide range in metabolizing ability for all detoxification pathways, including both cytochrome P450 systems and conjugating pathways.68 The most well-described polymorphism in this category was due to the absence of cytochrome P4502D6. Such individuals, comprising 5% to 10% of Caucasian populations, are referred to as poor metabolizers. These individuals have a severely impaired capacity to metabolize more than 25 therapeutic drugs and a wide range of xenobiotics.69 On the other end of the spectrum are individuals described as extensive metabolizers. Such individuals require a higher dose of several drugs to achieve therapeutic effects and may have a more robust capability to handle certain environmental toxins. Heritable polymorphisms are also known for several conjugating enzymes (phase 2 detoxification) that are extensively involved in the metabolism of exogenous chemicals.70 For example, genetic polymorphism of the glutathione-S-transferase (phase 2, glutathione conjugation) enzyme family is known to occur.71 This is well regarded as a significant factor conferring susceptibility to environmental toxins. The significance of these genotypic variants to the incidence of functional illnesses, such as chronic fatigue syndrome or fibromyalgia, is likely but has not been studied.
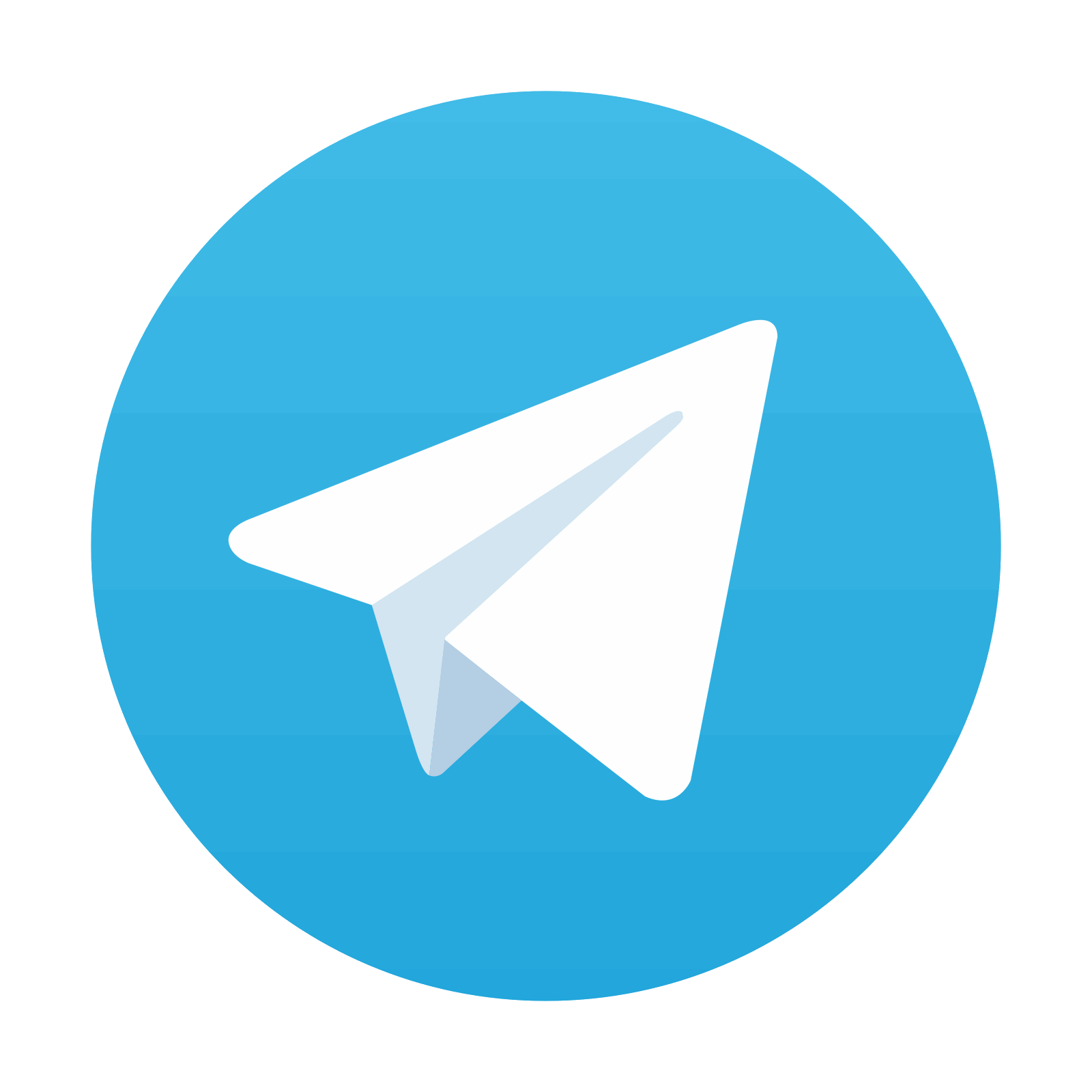
Stay updated, free articles. Join our Telegram channel

Full access? Get Clinical Tree
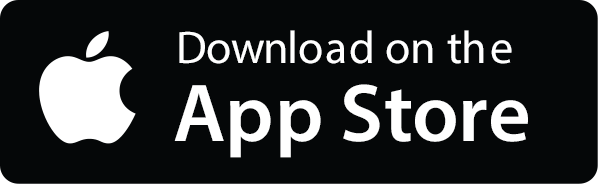
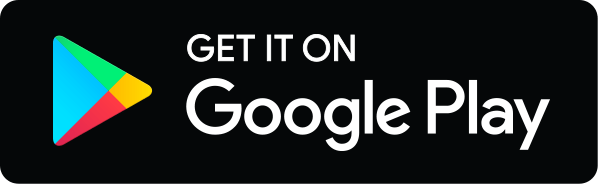