2
Functional Anatomy
Sharon R. Flinn and Lori DeMott
Normal Postural Mechanics
There are observable muscle behaviors that influence our posture and purposeful movements, also termed as our functional anatomy. Important distinctions should be made between anatomic characteristic of body positions, both at the initial static position of a kinematic event and then the return to our neuro-muscular-skeletal equilibrium of balance.1 Static posture is the stationary position held against gravity, whereas dynamic posture refers to a series of positions that constantly change during movement and function. Both postures require equilibrium of the muscle system and are observed during relaxation, standing, sitting, or lying down.1
The neutral resting balance of our anatomy is the state of default or the zero position of the body.2 The zero position is different from the anatomical position of the body. It represents the normal resting balance position where the upper extremities align themselves in space against gravity and where movement ceases and loads are removed. In zero position, the upper extremities are positioned in the midrange of glenohumeral and forearm rotation, the wrist is positioned in approximately ten degrees of extension, and the finger joints are positioned in approximately 45 degrees of flexion. Abnormal changes in functional anatomy cannot be understood without knowledge of the normal resting balance position. Fig. 2-1 illustrates zero position.
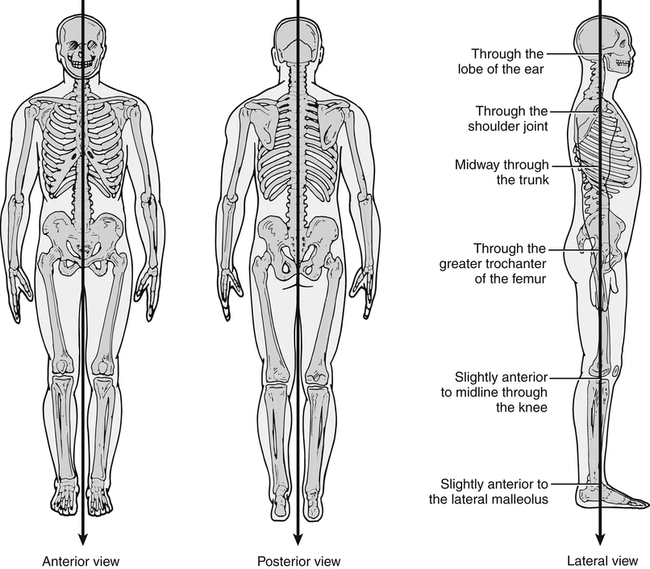
A, Anterior view. B, Posterior view. C, Lateral view. (Modified from Cameron MH, Monroe LG: Physical rehabilitation: evidence-based examination, evaluation, and intervention, St Louis, 2007, Saunders Elsevier.)
The upper extremities return to this default resting position, or zero position, in most static body postures. It is an assumed position of joint alignment where the tone of muscle activity is minimal, where the origins and insertions are in a “resting” tone, and where the tension of the joint is in a relaxed, balanced position. Tone is defined as the continuous and passive-partial contraction of the muscle or the muscle’s resistance to passive stretch during the resting state.3
Functional anatomy, defined by postural kinematic expressions, is fundamentally based on a predictable design. Skeletal conformation is observed and analyzed by envisioning the underlying bone positions. By knowing the preset configuration, or default design, you can visually construct the muscle anatomy and its contribution to posture. The gross structure, knowledge of fiber alignment, location, and the origins and insertions from your human anatomy references will assist you in understanding the natural tone and potential force of a specific muscle. The angles and lines that are produced as well as the conformation of our body are indications as to how synergistic muscles and peripheral nerves are performing. Typically, the points of reference are anatomical landmarks and are observed in two body planes. The coronal plane is vertical and divides the body into anterior and posterior halves.2 From the coronal plane, we draw a linear line, or plumb line, that forms an axis of reference. From the lateral view of the client, the alignment of the ear, shoulder, lateral elbow, posterior hip, anterior knee, and lateral malleolus are body landmarks that are located close to, if not directly within, the axis of reference. From the axis, you can observe the flexion, extension, anterior, and posterior adaptations of the body. Similarly, the sagittal plane is vertical and divides the body into right and left right halves.2 Postures viewed from all sides include obvious body markers, such as head position, shoulder height and glenoid orientation, clavicle angle, scapular position, antecubital fossa (carry angle), hand orientation, and hip height. Fig. 2-1, A and B, illustrates the body in the sagittal plane and provides the anterior and posterior views of the body. Fig. 2-1, C, illustrates the body in the coronal plane and provides a lateral view of the body with a clear view of the arm position.
Bones, Ligaments, and Joints
The joint allows freedom of movement from one to three planes. Two bony segments move in relationship to each other. Usually one segment is stable while the other moves in relation to the base. At the joint there can be more than one articulation. For example, the glenohumeral joint has scapulo-humeral, sterno-humeral, and clavico-humeral articulations.2 The control and stability of the joint’s axis of rotation directly relates to the articular orientation of the bones. Without rules of engagement for the joint, the simplest movement can become weakened by loss of mechanical advantage. This is seen in joint dislocation and a segmental bone fracture, whereby the movement is not guided and irregular angulations are observed. Conversely, if the segments of bone are not congruent and there is altered space that changes the axis of movement, the normal extrinsic muscle pull can be offset and the movement will present with distorted mechanics that are less than desired.
The normal skeletal system of the upper extremity can be mapped to determine the normal default state of zero position. An imaginary overlay of the skeleton on the conformation of the body creates the approximate location of the joints and bone segments. These structures assist you in recognizing important landmarks. An example of important landmarks from Fig. 2-1 is the space between the arm and body. Fig. 2-2 identifies the mapping specific to the hand. Important landmarks for the hand are nail positions, the space between the index and thumb, the cascading flexion of the fingers, the mass of the thenar eminence, and the prominence of the ulnar head.
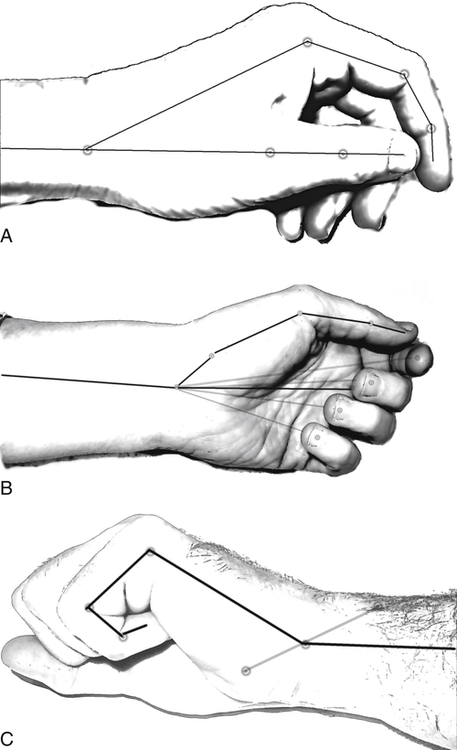
Altered configuration of the joint’s soft tissue matrix or bony constructs lead to the joint’s failure to glide and can result in various types of joint collapses and deformities.3 With joint laxity, the axis shifts into the direction of the weakened, degraded tissue integrity. Frequently, this is seen with attritional changes of the glenohumeral joint where the anterior capsulo-ligaments are weakened and attenuated. A shift in the joint axis results in changes in the anterior and posterior capsule and in the formation of adhesions.
Similar attritional changes often are observed with joint dysfunction to the carpometacarpal (CMC) joint of the thumb. Synovitis of the joint causes bone surface erosions and ligament laxity. Laxity of the stabilizing deep anterior oblique ligament (DAOL), called the beak ligament, causes the joint to sublux in a radial and palmar direction. Over time, the degenerative thumb CMC joint exhibits changes in appearance as seen with a prominent ledge that extends from the radial side of the base of the thumb. The loss of joint stability changes the characteristic of the surrounding muscular anatomy. The abductor pollicis brevis weakens and the adductor pollicis muscle contracts, unopposed. The thumb CMC joint is drawn into adduction and rotational supination. The imbalance of the thumb intrinsics change the axis of rotation, decrease the transmission of flexion forces across the metacarpal joint, and move the resting position of the MP joint from a flexed posture to extension. Over time, the metacarpal joint hyperextends as the mechanical advantage from the extensor pollicis brevis (EPB) muscle further aggravates the adductor pull on the CMC joint and the increasing severity of the contracture. The interphalangeal (IP) joint compensates for loss of metacarpal joint flexion and overly flexes to produce a functional tip pinch. The cascading events are self-perpetuating as the normal joint forces become pathologic. The anatomy of the arthritic thumb presents with imbalances, principally at the CMC joint level, with bone erosions and capsular instability that contribute to the distal joint changes at rest and during dynamic loads. Fig. 2-3 illustrates mapping of a normal and pathological CMC joint. The dots estimate the joint location, the stars are the landmarks, and the dashes are the bone segments. The disparities between the two thumbs are clear. Compared to the normal thumb, imbalances in the pathological thumb are identified by three visible landmarks; increased nail rotation, MP joint hyperextension, and a protuberance at the CMC joint caused by the subluxing metacarpal.
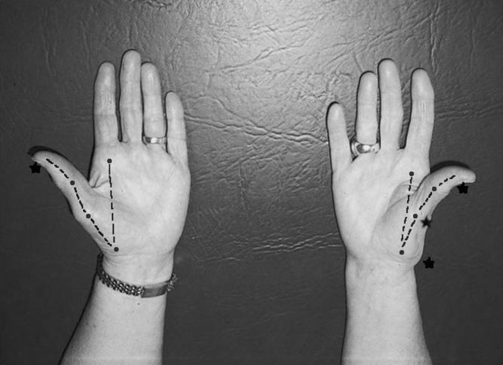
Muscles and Tendons
Muscles work in groups and patterns of movement. Individual muscles have lengthening, contractile, excitable, and recoil characteristics. Contractility allows a muscle to shorten with force, to lengthen passively, and to move. Excitability allows a muscle to respond to a stimulus and to maintain chemical potentials across its cell membranes. Extensibility allows a muscle to be stretched, repeatedly and considerably, as needed, without being damaged. Elasticity allows a muscle to return to its normal length after being stretched or shortened. The end result is a force application. For example, a coordinated neuromuscular event occurs during the conscious decision to make a fist. The wrist extensors stabilize the wrist in approximately 35 degrees of extension, the extrinsic extensor digitorum communis (EDC) elongates into a full extensible position as the antagonist muscle, and simultaneously the extrinsic flexor digitorum profundus (FDP) and flexor digitorum superficialis (FDS) contract in their role as agonist muscles. The coordinated muscle contractions differentiate the glide that allows terminal distal joint flexion. Simultaneously, contraction of the intrinsic lumbrical and interossei muscles increase metacarpal joint flexion, stabilize and control the joint, and allow the digits to converge into a tucked position within the palm. The characteristics of the musculotendinous structures that contribute to muscle balance are located in Box 2-1.3
Nervous System
The peripheral, central, and autonomic nervous systems all combine to form one internal communication system for sensing and responding to internal and external stimuli.4 The motor and sensory functions for the forearm, wrist, and hand are provided by the peripheral nervous system; consisting of the median, ulnar, and radial nerves. Each nerve has efferent motor and afferent sensory fibers arranged in bundles of axons. The axons are protected by layers of dense connective tissues called the epineurium, perineurium, and endoneurium. Each layer has a unique role to support the innermost structures of the nerve, modulate compressive and tensile forces, and allow gliding between nerve fascicles and the surrounding anatomical structures.5 Electrical impulses are rapidly conducted via the nodes of Ranvier along a neural pathway, which originates at the spinal cord and brainstem and terminates in the fingers and toes. Due to the continuous nature and physiology of the peripheral nervous system, motions at a distal site, such as the wrist joint, increase the strain at the cord level of the brachial plexus. Similarly, contralateral flexion of the cervical spine increases the strain in the cords of the brachial plexus and three major nerves in the arm.4 Under tension, the neural tissue may have difficulty conducting electrical impulses, assuring adequate blood supply, and providing adequate axonal transport especially during movement.5
Several biomechanical properties are necessary to protect the nerve from tensile, shear, and compressive forces that occur across multiple joints in various postures and dynamic movements of the upper extremity during everyday activities.5 The properties of the nerve protect it from the forces generated from whole body movements resulting from everyday activities. These protections are due to the nerve’s capacity to change its length during movement, slide or glide relative to the surrounding interfacing tissue during movement, and tolerate increases in pressure or compression from the surrounding interfacing tissue.5 When the nerve does not tolerate normal stresses, a “sensitized” protective state may result as the upper extremity joints usually adjust by flexion or extension to limit the nerve excursion. Symptoms can include increased pain or aberrant movements in the absence of altered nerve conduction.4,5
Observations of static and dynamic postures are essential to evaluate the neurodynamics of the peripheral nervous system in its entirety and the changes which can result from limited excursion or traumatized segment(s) of the nerve.4 As simply observed in an acute nerve injury, the upper arm is held tightly in shoulder adduction, internal rotation, and elbow flexion. In the presence of long-standing trauma to the nerve, imbalances in motor function can be observed. For example, hyperextension of the thumb MP joint during resistive lateral pinch can be observed in persons with ulnar nerve palsy. A zigzag deformity is observed as a result of absent function of the adductor pollicis muscle and imbalances created from a weakened intrinsic extensor mechanism and overpowering extrinsic long flexor.
Scenario 1
How does proper cervical alignment facilitate normal functioning of blood vessels and nerve roots?
Proper cervical alignment of vertebrae, muscle, and soft tissue facilitates normal conduction and excursion of nervous tissue in nerves C1 to C8. In addition, alignment of these structures also provides for normal blood flow within the vertebral artery and the vertebral and deep cervical veins, insuring adequate blood flow and drainage for the cervical structures related to upper extremity function. Fig. 2-4 illustrates the anterior rami of nerves C5 to T1, which form the brachial plexus and innervate the entire upper extremity.
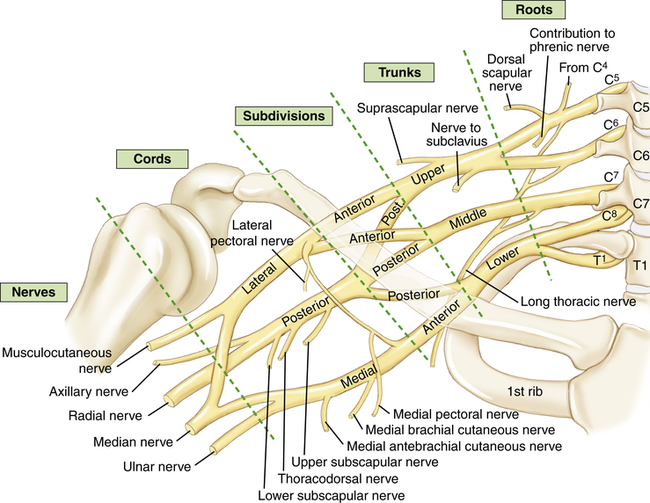
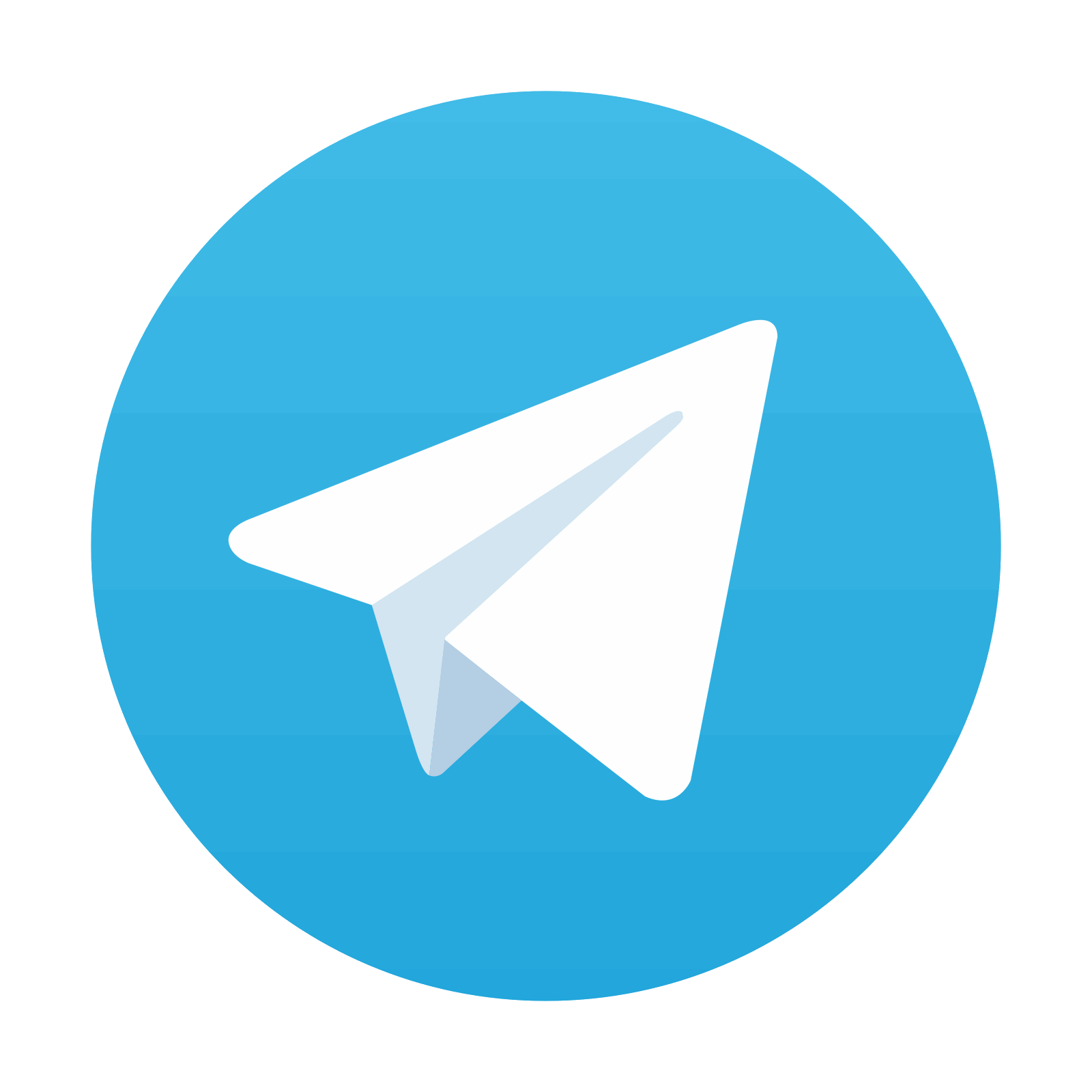
Stay updated, free articles. Join our Telegram channel

Full access? Get Clinical Tree
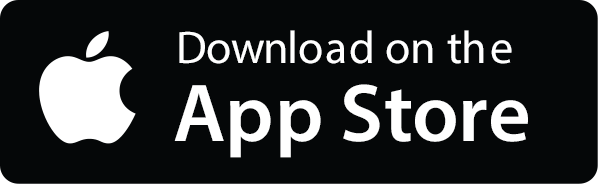
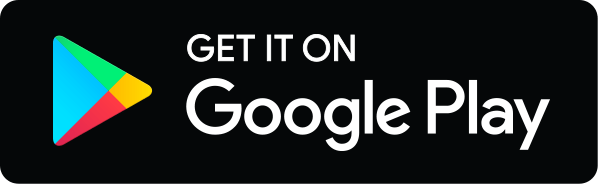