Fracture considerations
Timothy L. Kauffman and Carleen Lindsey
Introduction
The fracture of a bone has a profound impact on any member of the aging population, as the consequences may negatively impact independence and can even lead to death (Leslie et al., 2013). Fractures in aging individuals are usually associated with low bone mineral density (BMD) and osteoporosis (see Chapter 18), as defined by the World Health Organization in Box 60.1. It is estimated that there are nearly 9 million osteoporotic-related fractures a year worldwide. One in five females over the age of 50 in developed countries is likely to sustain a hip fracture with associated high morbidity and mortality (WHO, 2007). The proportion of all fractures from osteoporotic bone reported from international community-based populations is 0.716–0.924. These data include males and females aged 50 years and older (Morrison et al., 2013).
In a population study of all persons over age 49 from Manitoba, 5-year survival after hip fracture was 51% females and 36% males. The average first year costs were 23 361 Canadian dollars for hip fractures and total healthcare costs for all incident fractures were $194 million (Leslie et al., 2013). In the US there were about 2 million osteoporotic fractures in 2010 with costs projected to exceed $18 billion (Levis & Theodore, 2012).
A bone fractures when a force or stress is placed upon it that is greater than the bone can withstand. Bone has a tensile strength of approximately 140 MPa (megapascals; one MPa equals 145 pounds per square inch) in the second decade of life, and it decreases to approximately 120 MPa by the eighth decade of life. The fracture threshold for the vertebrae and the epiphyseal areas of the femur is a bone density of less than 1 g/cm3 (Gerhart, 1995). Even though the stiffness and strength of trabecular bone depends on bone density and the direction of loading, trabecular bone appears to be more susceptible to failure from shear rather than compressive loads (Sanyal et al., 2012). The major physical difference between trabecular bone and cortical bone is the increased porosity exhibited by trabecular bone. This porosity is reflected by measurements of the apparent density (i.e. the mass of bone tissue divided by the bulk volume of the test specimen, including mineralized bone and marrow spaces). In the human skeleton, the apparent density of cortical bone is about 1.8 g/cm3, whereas the apparent density of trabecular bone ranges from approximately 0.1 to 1.0 g/cm3
Fragility fractures are usually related to lower BMD; however, urinary markers of bone resorption including collagen cross-linked N, C-telopeptide and free deoxypyridinoline may be predictive of hip fracture independent of bone mass (Garnero et al., 2009). Additionally, the large majority of fractures result from falls and physical interventions are successful but probably not utilized as widely as they should be (see Chapters 15, 16, 58, 59).
Normal fracture healing
Normal fracture healing can be divided into three overlapping phases. First, there is an immediate inflammatory phase in which there is bleeding resulting from the injury to the bone and surrounding soft tissue, and a hematoma forms (Fig. 60.1A). The bone cells at the fracture line die. The reparative, or proliferative, phase starts shortly after the injury, usually 24–48 hours if a good local blood supply to the fracture exists (Fig. 60.1B). Good reduction and immobilization of the fracture also help the bone during the reparative phase. Osteogenic cell proliferation lifts the fibrous layer of the periosteum from the bone and, somewhat more slowly, the osteogenic cells of the bone marrow cavity also proliferate (Fig. 60.1C). This proliferation gradually forms a collar, or callus, around the fracture line, which usually takes place in 2–4 weeks, but radiographic evidence of external callus formation may not appear until 3–6 weeks. A bone scan usually reveals increased metabolic activity shortly after the fracture and before the callus can be seen on a radiograph (McRae, 2008).
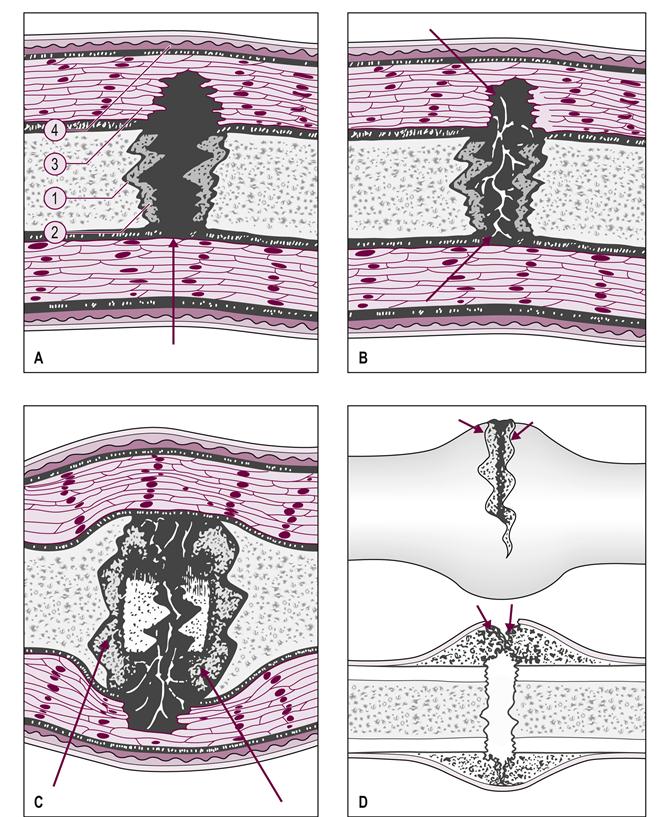
(A) Bleeding occurs from the bone ends, marrow vessels, and damaged soft tissues, with the formation of a fracture hematoma that clots (closed fracture is illustrated). (1, periosteum; 2, haversian systems; 3, muscle; 4, skin.) (B) The fracture hematoma is rapidly vascularized by the ingrowth of blood vessels from the surrounding tissues and, for some weeks, there is rapid cellular activity. Fibrovascular tissue replaces the clot, collagen fibers are laid down and mineral salts are deposited. (C) New woven bone is formed beneath the periosteum at the ends of the bone. The cells responsible are derived from the periosteum, which becomes stretched over these collars of new bone. If the blood supply is poor, or if it is disturbed by excessive mobility at the fracture site, cartilage may be formed instead and remain until a better blood supply is established. (D) If the periosteum is incompletely torn, and there is no significant loss of bony apposition, the primary callus response may result in establishing external continuity of the fracture (‘bridging external callus’). Cells lying in the outer layer of the periosteum itself proliferate to reconstitute the periosteum. (Reproduced from McRae R 2008 Practical Fracture Treatment, 5th edn, by permission of Churchill Livingstone.)
The remodeling phase starts during proliferation as the osteogenic cells begin to differentiate into osteoblasts, which start to form bony trabeculae that bridge the living and dead bone across the fracture line (Fig. 60.1D). Some of the osteogenic cells differentiate into chrondrocytes and form cartilage in the fracture callus, which eventually calcifies, becoming bone. Osteoclasts gradually remove the necrotic bone at the fracture site. The callus, consisting mostly of cancellous bone that has now formed across the fracture site, is fusiform. The cancellous bone is slowly remodeled into compact bone and, finally, the original fracture line is no longer discernible.
Fracture repair in the aging individual
The rate of fracture repair in the aging patient should always be considered to be similar to that of a younger person with early callus formation in 2–4 weeks and bony bridging over the fracture in 6 weeks, as shown on radiographs. However, a host of factors such as degranulating platelets, cytokines, transforming factor-beta, interleukins 1 and 6, prostaglandin E2 and tumor necrosis factor alpha (Borelli et al., 2012), are crucial to this normal progression. Osteoporotic bone may not heal as well as bone with normal tissue density. The inflammatory response to the injury and the blood supply may be inadequate, and failure to immobilize the fracture site also delays the healing process. Morphogenetic proteins and growth factors in fracture healing and adequate nutrition are crucial. Diabetes and hypovitaminosis, especially vitamins D and C, have deleterious effects on bone repair. Polytrauma, chronic inflammation (Borelli et al., 2012), overall health or frailty as well as impaired cognition can also delay the healing process (see Chapter 18).
In the case of open reduction and internal fixation of a fracture, there is greater risk of further bone injury, called a stress riser, due to the orthopedic hardware. The use of screws and plates may weaken or pull out from bone that is already osteopenic.
Special fractures in the elderly
Not all fractures in the elderly are considered to be complete fractures. Stress fractures, also referred to as insufficiency fractures, occur in areas of repeated trauma when bone remodeling is insufficient to repair the stresses of repetitive loading. Clinically, this is a particular concern when treating a patient who is at risk for increased bone fragility. Orthopedic internal fixation devices can become stress risers and cause increased loosening at the bone–appliance interface (Kim et al., 2011). People with spinal cord injury who have been fitted with a new orthotic device may develop stress fractures due to new movement abilities. The sedentary and overweight elderly, especially with low vitamin D (Breer et al., 2012), are at risk when they start new, strenuous physical activities. Physically, these patients will present with pain, swelling and warmth. Stress fractures or pseudofractures may arise in bone that has faulty mineralization, which is associated with inadequate repair of microtraumas.
Microfractures of the bony trabeculae have been demonstrated. The proclivity of these microfractures to cause pain is unclear. However, they may progress and lead to the silent fractures that are recognized on radiograph but may be old fractures. Despite the radiograph evidence of fracture, a patient can be unaware of having experienced any frank trauma – hence the term ‘silent fracture’. This may be one of the causes of the lumbar kyphosis that is seen in some individuals who spend excessive amounts of time sitting.
Occult fractures, also referred to as insufficiency fractures, are best diagnosed with bone scan or magnetic resonance imaging (MRI). This type of fracture is usually intramedullary and undisplaced, and frequently occurs as a result of some minor or major trauma, but radiograph examination is negative. Typically, occult fractures occur in the proximal femur or humerus after a fall, but they have also been reported in the sacrum, acetabulum, calcaneus, tibia and spine. Quickly these patients present with moderate to severe pain and tenderness. There is a concomitant reduction in range of motion and strength and, if in the femur, there is a marked antalgic gait. In nursing and rehabilitation this type of fracture should be treated seriously even if it has not been confirmed on initial radiograph. If pushed too aggressively, complete disruption of bone may occur. Protected ambulation with a walker is requisite while the femoral or pelvic occult fracture heals.
A pathological fracture results from primary or metastatic malignant tumors in bone. These types of fractures usually present as pain without any reported history of trauma; however, at times, metastatic bone disease is found in a patient who is being radiographed because of trauma. Significantly, these patients complain of increased pain at night and of being awakened by the pain. The pain frequently increases with bedrest and the severity increases with time. Those presenting with primary tumors of the breast, prostate, thyroid, kidney, or other organs should be suspected of having metastatic disease if pain complaints fit these descriptions. Standard radiographs are helpful for specific bony sites; however, for diffuse bone metastasis, a nuclear medicine bone scan may be important for a total skeletal evaluation (see Chapter 14).
Future methods of promoting fracture healing
As stated above, natural fracture repair in the elderly may not proceed in precisely the same pattern as repair proceeds in younger individuals. However, several medical and physical methods for enhancing bone repair are being investigated. A number of factors have been found that influence fracture repair, including fibroblast growth factor, platelet-derived growth factor, transforming growth factor 13 and bone morphogenic protein. Insulin-like growth factor may stimulate fibroblast proliferation (Glass et al., 2011; Borelli et al., 2012).
Ceramic composites of calcium phosphate have been used for bone grafts. Electrical stimulation and ultrasound at specific parameters are two physical modalities that are currently being used to promote fracture healing. Fracture treatment in the future is likely to involve active intervention to promote healing and thereby reduce morbidity.
Therapeutic interventions
Osteoporosis-related fractures
Osteoporosis is caused by increased action of osteoclasts (cells that absorb bone) or decreased action of osteoblasts (cells that lay down bone). It affects cancellous (trabecular) bone more than cortical bone. The areas of the human skeleton that are most likely to fracture as a result of osteoporosis are neck of the femur, vertebral bodies, humerus and wrist (WHO, 2007). A vertebral compression fracture (VCF) is common in an individual with osteoporosis, and it is often the first indication that a person has osteoporosis. Estrogen is protective of bone and prevents osteoporosis, whereas long-term steroid use has the effect of weakening the bone and increasing osteoporosis. Weight-bearing exercise has been shown to be protective of bone strength, and is associated with decreased bone resorption and increased osteogenesis (Kemmler et al., 2013). Postmenopausal women in Western culture are at the highest risk (see Chapters 18 and 57 for further discussion of this subject).
Treatment of someone with an osteoporosis-related fracture consists of promoting healing, preventing deformity and facilitating the individual’s return to full functioning. This type of fracture should not be viewed as an isolated event. It is usually the harbinger of future fractures. Thus, prevention of future fractures should be part of the treatment plan (Eisman et al., 2012). In working with a patient who has had a compression fracture, the nurse or therapist should screen carefully for any signs of neurological compromise. By definition, compression fractures do not involve the posterior portion of the vertebral body and so do not involve a risk of protrusion of fractured bone into the spinal canal. If neurological signs are present, the client should be referred for studies to determine the presence of burst fracture or fracture dislocation.
Pharmacological interventions to promote healing
Persons with osteoporotic fractures are likely to be given medications to reduce risk of future fractures. These include bisphosphonates, estrogen, estrogen agonist/antagonist (formerly SERMs), parathyroid hormone, calcitonin and denosaumab. Strontium renelate is available in Europe but not in the United States of America. These medications may exhibit some potential negative effects and there are reports of jaw osteonecrosis and atypical femoral shaft fractures associated with bisphosphonate use. Bisphosphonates inhibit bone resorption and may cause impaired bone formation which is then responsible for these unusual fractures (Rizzoli et al., 2011).
The nurse or therapist treating someone who is taking bisphosphonates may assist by ensuring that these medications are being taken correctly. They must be taken on an empty stomach with 8 fluid ounces (236.6 ml) of water. The individual should be upright after ingestion and should wait 30 minutes before eating. The side-effects of gastrointestinal upset may be worsened if these guidelines are not followed. Another medication that inhibits bone resorption is salmon calcitonin, which is either injected or used as a nasal spray. It may be given to those who cannot take any of the above medications.
Pain management
An individual with a vertebral compression fracture (VCF) is likely to have pain with movement and might need instruction in log-rolling (moving with no trunk rotation while rolling). The use of a neoprene lumbosacral corset with gel-foam lumbar support, clavicle strap, Spinomed (Pfeifer et al., 2004) or Jewett brace may prevent extraneous motion and facilitate mechanically sound trunk muscle use to minimize pain. In our experience, modalities such as gentle manual therapy, postural taping (Bennell et al., 2010), cold, heat and pulsed ultrasound with high-voltage galvanic electric stimulation are effective in reducing pain during the 6-week acute healing phase (see Chapters 67 and 68). Caregivers also need to be instructed in safe transfers using the pelvis for contact guarding rather than putting any compression through the trunk. They also need to be made aware of bed and chair positioning with spinal alignment such that lumbar lordosis is supported and forward head with kyphotic posture minimized.
Water exercises can be done, as the buoyancy of water provides a comfortable, gravity-free environment, but the client must eventually transition to a land-based program in order to develop strength and polish the skills necessary to live in a gravitational environment (see Chapter 73).
Prevention of further injury
Although the effects of a diet change on bone strength will take longer to be seen, the individual who has suffered a fracture may be amenable to changes in diet that will help to prevent future fractures. Referral to a registered dietician is indicated (see Table 60.1 for recommended intake for calcium and Table 60.2 for vitamin D) and further information about diet, calcium and patient education can be found in Chapter 18).
Table 60.1
Recommended dietary allowance (RDA) for calcium
Age | Male | Female | Pregnant | Lactating |
0–6 monthsa | 200 mg | 200 mg | ||
7–12 monthsa | 260 mg | 260 mg | ||
1–3 years | 700 mg | 700 mg | ||
4–8 years | 1000 mg | 1000 mg | ||
9–13 years | 1300 mg | 1300 mg | ||
14–18 years | 1300 mg | 1300 mg | 1300 mg | 1300 mg |
19–50 years | 1000 mg | 1000 mg | 1000 mg | 1000 mg |
51–70 years | 1000 mg | 1200 mg | ||
71+years | 1200 mg | 1200 mg |
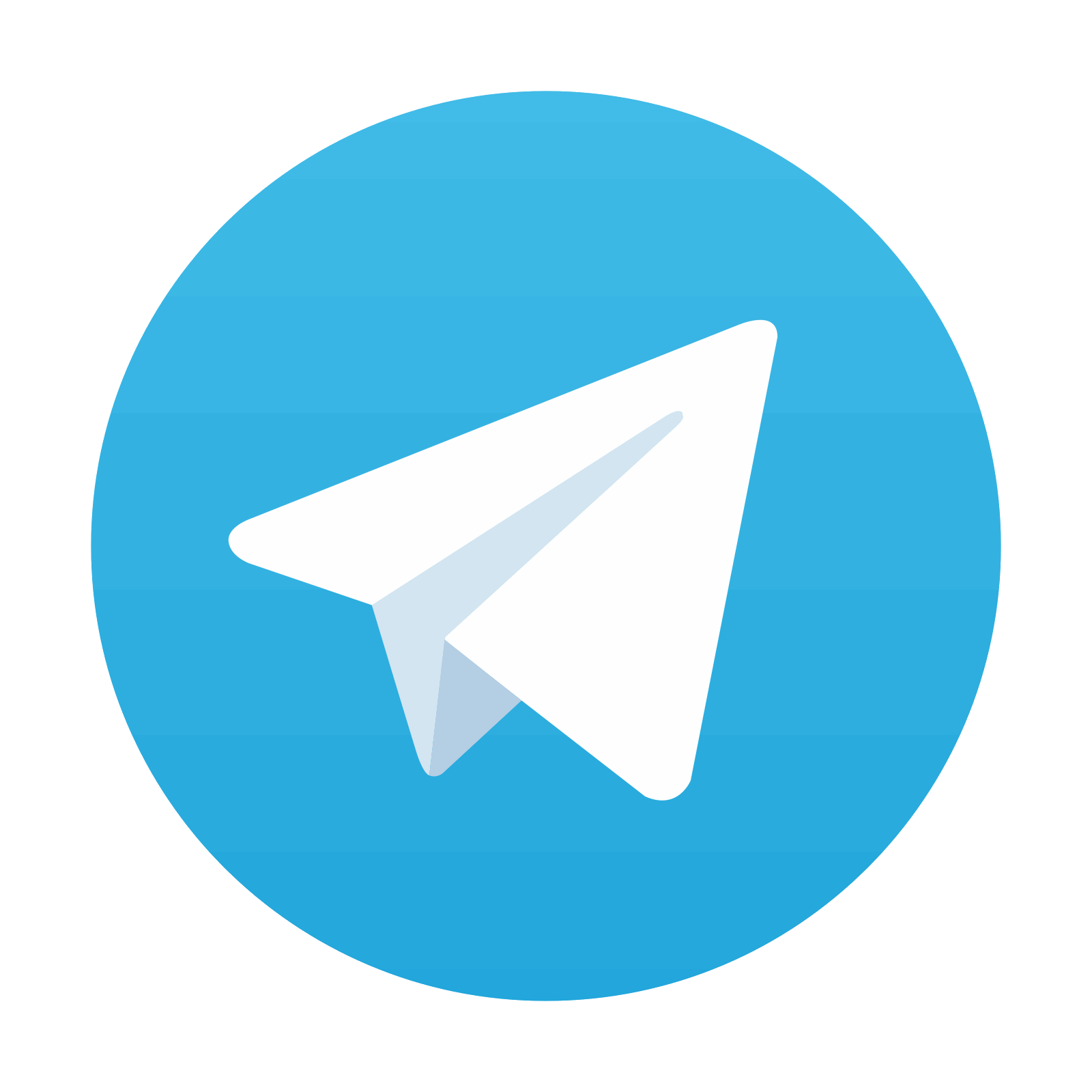
Stay updated, free articles. Join our Telegram channel

Full access? Get Clinical Tree
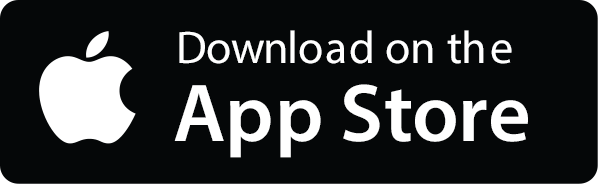
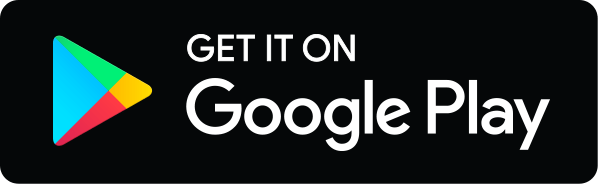