Invasive cardiac procedures
Chris L. Wells
Introduction
Invasive procedures for the treatment of cardiac pathologies, such as catheterization, angioplasty and bypass surgery, have become commonplace over the past 40 years. Over 4.5 million invasive cardiac procedures are completed annually in the United States of America (CDC National Center for Health Statistics, 2012) and there have been many advances in the surgical management of cardiac disease, with significant advancements in minimally invasive cardiovascular procedures. The age and number of comorbidities of elderly patients have increased the complexity of the procedures performed; unfortunately, however, these factors affect outcomes such as a return to prior level of function and quality of life. This chapter will briefly discuss the various invasive procedures for the management of heart disease, particularly coronary artery disease.
Catheterization
Cardiac catheterization remains the gold standard procedure in the diagnosis of cardiac function and cardiac disease (see Fig. 44.1 for a description of the clinical name of the pressures within each of the chambers of the heart and their average values). A right heart catheterization (RHC) can be used to assess the volume and pressures within the cardiopulmonary system and is completed by placing a catheter within the right side of the heart, typically via the internal jugular, femoral or brachial veins. This catheter can measure how much blood is returning to the heart, referred to as preload, by recording the pressure within the right atrium, which is clinically referred to as central venous pressure. The physician can measure the function of the right ventricle and pulmonary vascular system by measuring the volume and pressure through the right ventricle and into the pulmonary trunk (pulmonary arterial pressure). The balloon at the end of the catheter can be inflated and used to indirectly measure the preload of the left side of the heart (pulmonary capillary wedge pressure). This procedure can also be used to estimate blood gases, cardiac output and function of the tricuspid and pulmonic valves and to detect septal defects. Finally, RHC can be used to diagnose pulmonary hypertensive diseases, assess extent and location of embolism, take a tissue biopsy and evaluate the responsiveness to medications used to improve heart function and decrease pulmonary hypertension.
A left heart catheterization (LHC) is commonly used to diagnose coronary atherosclerosis; this helps to determine the state of perfusion of the myocardium. The catheter is passed into the arterial system through the femoral or brachial artery. At the time of the coronary arteriography, a ventriculography can be completed for assessment of left ventricular function, including description of wall motion and function of the mitral and aortic valves and measurement of the ejection fraction, blood gases, the blood volume of the left ventricle, and cardiac output. Heart catheterization can also be used to assess the health of the extracardiac major blood vessels of the body (DiMario & Sirtaria, 2005). More recently transcatheter aortic valve replacements are being performed for individuals who are not candidates for surgical intervention for the traditional median sternotomy approach for aortic valve replacement (Webb et al., 2012). Clinical trials are underway that are investigating mitral valve repairs via percutaneous catheter as well (Rogers & Franzen, 2011).
Therapeutic intervention
Activity restrictions will vary depending on whether a patient has a RHC or a LHC. If the patient undergoes only a RHC, once the catheter is removed from the vein, pressure is applied to the site for 2–5 minutes to ensure that bleeding has stopped. The patient is then allowed out of bed and can resume activities as tolerated and as medically indicated. After undergoing a LHC, direct pressure is applied for 5–20 minutes or until the bleeding has stopped after which a pressure dressing is applied and the limb immobilized. The physician may insert a vascular plug or a suture to seal the puncture site of the artery but the patient will typically need to be on bedrest. If the femoral artery is the site for catheterization, the patient will be on bedrest for 4–6 hours. If the brachial artery is the site for the LHC, the patient may be allowed out of bed in 2–4 hours but the extremity will need to be elevated and immobilized for 4–6 hours. When the patient and the extremity are permitted to be mobilized, it is important for the clinician to inspect the arterial site for bleeding or the development of a hematoma before and after the therapeutic intervention. If bleeding persists or a hematoma has developed, it is critical to notify the physician in order to control the bleeding and assess the artery for the development of an aneurysm. The therapist should also complete a motor and sensory examination to determine if there are any focal deficits, particularly involving the femoral or median nerve distribution depending the arterial approach. There is also the risk of renal dysfunction because of the dye that is used during the arteriography, which may lead to peripheral edema, muscle weakness, changes in mental status and heart failure, which the therapist will need to assess and adjust the rehabilitation plan.
Percutaneous coronary intervention (PCI) angioplasty
Percutaneous coronary intervention (PCI) – previously referred to as a percutaneous transluminal angioplasty (PTCA) or angioplasty for short – may be performed in the cardiac catheterization laboratory when a diagnosis of coronary atherosclerosis has been confirmed. For a noncalcified discrete lesion involving the proximal artery of one or two vessels, PCI with or without stent placement is a common medical option for management of coronary disease (Wu et al., 2011). Heart function and comorbidities, such as diabetes mellitus and acute myocardial infarction (MI), particularly ST segment elevated MI, should also be taken into account when the specific invasive procedure is selected (Kalesan et al., 2012).
By performing a PCI, it may be possible for the cardiologist to open the occluded artery and restore blood flow. A guide wire and catheter are inserted using the same procedure as in LHC. The guide wire is advanced through the atherosclerotic lesion and a dilatation catheter balloon is inserted over the guide wire. The balloon is then inflated, with the goal of redistributing the atherosclerotic plaque. The result is an enlargement of both the lumen and the overall diameter of the vessel. The balloon is then deflated and an angiography repeated to assess the effectiveness of the PCI. The patient is typically administered heparin or bivalirudin to decrease the risk of thrombus formation and nitroglycerin may be administered into the coronary artery to prevent vasospasm. The PCI can be repeated if necessary or be performed on other involved arteries. The catheter sheath may not be removed immediately after the procedure in order to have rapid access if the patient experiences angina or signs suggesting ischemia or vasospasm.
Although PCI is a minimally invasive procedure and is associated with an approximate acute success rate of 90% (Tresukosol et al., 2010), the procedure is associated with several complications. Venous thrombosis and embolization may occur, causing a cerebrovascular accident (CVA) or occlusion of another coronary vessel creating further ischemia or infarction. During the procedure, there is a risk of perforating or dissecting the coronary artery, which could lead to tamponade or MI. Tamponade or artery dissection requires emergency surgical intervention to stop bleeding and preserve myocardial function. The catheter can also cause life-threatening arrhythmia, bleeding or infection, and the development of a pseudoaneurysm may occur at the entrance site of the catheter, usually the femoral artery. Percutaneous coronary intervention is associated with a complication rate of 4.1%; in total, 29% of these complications are from arterial dissection. The restenosis rate has been reported to be 4% to 40% and, of patients who suffer from restenosis, the arterial closure leads to MI in up to 50% of cases, with mortality rates reported between 20 and 40%. Approximately 20% of patients will require surgical intervention (Heir et al., 2010).
Despite the advances in medical technology, there is a 30% chance of restenosis within the first year after a PCI. Restenosis within the first 6 months post-PTCA is associated with cell proliferation, macrophage infiltration, platelet agitation and neovascularization that leads to narrowing or occlusion of the coronary artery. After 6 months, it is believed that restenosis is caused by further progression of the coronary artery disease (Heir et al., 2010).
Stents
The use of an endovascular stent in PCI has been associated with a decreased rate of restenosis and reinfarction compared with the use of a PCI alone. The benefit of stents is that a larger lumen can be achieved and there is a decrease in elastic recoil of the artery (Kalesan et al., 2012). These stents may also be placed after a PCI when there is an acute restenosis. In the US, over a million PCI procedures are completed annually with over half of the individuals also receiving an intra-arterial coronary stent (Centers for Disease Control and Prevention 2012). The stent is guided into place across the atherosclerotic plaque over the guide wire. Once in position, the stent either self-expands or a balloon is inflated to disrupt the lesion and dilate the coronary artery to restore myocardial perfusion.
There are two basic types of stents used today to manage coronary artery disease: bare metal stents and drug-eluting stents, which are used 75% of the time (CDC, 2012). The implantation of a stent decreases the reocclusion rate, referred to as in-stent stenosis, and reinfarction rate when compared to angioplasty alone (Guagliumi et al., 2011; Kalesan et al., 2012). A stent can be coated with heparin or another drug that actively interrupts the development of restenosis. Thus, drug-coated or drug-eluting stents can be covered with such drugs as sirolimus or rapamycin. Sirolimus actually decreases endothelial function and affects platelet physiology (Lemos et al., 2003) and rapamycin inhibits cell proliferation (Arjomand et al., 2003). Drug-eluting stents are associated with a decrease in early restenosis and reinfarction and improvements in mortality rates compared to bare metal stents. Bare metal stents have a decrease in late restenosis, 1 year after implant, especially if the patient has recently experienced an ST segment elevation myocardial infarction (Douglas et al., 2009; Kalesan et al., 2012). Along with a drug-eluting stent, the patient is typically placed on an anticoagulation medication for 6–12 months to decrease risk of thrombosis formation and aspirin indefinitely to reduce incidence of future MI. If stenosis occurs, angina symptoms will return and further intervention is required to stabilize myocardial function. This may include inserting another stent, PCI, atherectomy, laser or radiation therapy or coronary artery bypass.
Atherectomy
There are four general types of atherectomy procedure that can be used to debulk or remove a thrombosis or atherosclerotic plaque and restore coronary blood flow. Atherectomy can be used independently or in conjunction with PCI or stent deployment. The primary function of the atherectomy is to mechanically remove the plaque. A directional atherectomy (side-cutting) is best used when the lesion is located at a bifurcation or is eccentric and complicated. The rotational atherectomy uses a circular abrasive method and an atherosclerotic extraction device with cutting blades at the end of the endovascular instrument to debulk the artery. Laser has been successfully used to vaporize tissue in the case of ‘in-stent’ stenosis. Finally, a cutting balloon angioplasty, which is an atherotomy as opposed to an atherectomy, excises the lesion and dilates the artery by using a balloon catheter with a microsurgical blade (Guagliumi et al., 2011). Atherectomy as well as PCI is associated with the possibility of microembolic activity that can result in arterial occlusion of distal smaller arteries leading to other ischemic sites.
Lasers
The atherosclerotic lesion can be managed by an ablative laser atherectomy procedure. Direct ablation by laser is indicated for a lesion in a saphenous vein graft, in aorta–coronary artery ostial stenosis, for a fibrotic or calcified lesion, for a lesion that affects a diffuse area or in-stent restenosis. Based upon the cellular makeup of the plaque, the correct wavelength can vaporize the lesion. The most common complication of this application of lasers is perforation of the vessel, which may occur at a 5–10% rate (Topaz et al., 2009).
Lasers are also being used to conduct the Maze procedure (see below) for the treatment of atrial fibrillation and transmyocardial revascularization. These procedures will be briefly discussed later in this chapter.
Radiation
Intracoronary radiation, also known as brachytherapy, is being utilized to manage restenosis of treated coronary artery disease. The use of isotopes inhibits smooth muscle proliferation, delays the healing process and prevents the remodeling of the treated arteries. When brachytherapy is used in stent restenosis, there is a reduction of future restenosis by 50% (Price et al., 2007).
Therapeutic intervention
The therapeutic intervention during the acute phase after a catheterization procedure is similar to that described above for a LHC. Because the patient has been diagnosed with coronary artery disease and there is the risk of restenosis, it is important that the clinician educates the patient on the importance of compliance with routine medical check-ups and the use of medications, and the patient is able to recognize the signs and symptoms related to myocardial ischemia and MI. In the long term, it is important that the patient begins to minimize his or her cardiac risk factors, such as cessation of smoking, management of hypertension and diabetes, proper diet and weight management, and participate in a regular exercise program. Patients with heart disease benefit from cardiac rehabilitation where they are monitored during exercise, provided with valuable education, and develop a network of social support.
Coronary artery bypass surgery
In the presence of multivessel coronary disease, complex diffuse lesions, left main arterial disease, multivessel disease with left ventricular dysfunction and in patients with diabetes, coronary artery bypass graft (CABG) surgery is the invasive procedure of choice and is the most common cardiac surgical procedure performed (Sundt, 2013). The purpose of performing CABG surgery is to restore perfusion to viable myocardium by diverting blood around the atherosclerotic plaque to perfuse the myocardium distal to the occlusion.
Typically, the surgical approach is a median sternotomy, particularly for a multivessel bypass procedure. For isolated left anterior descending artery (LAD) blockages, CABG procedures are being carried out using a small anterior thoracotomy approach, referred to as a minimally invasive direct coronary artery bypass (MIDCAB) or keyhole CABG. See below for more details on MIDCAB procedures.
The vascular tissue that is harvested for the bypass procedure can be either arterial or venous in nature. Traditionally, the saphenous vein has been used to bypass the lesion by making an anastomosis of the vein to the aortic root and to a point distal to the lesion or stenosis. The vein is sutured in the reverse direction to prevent the valves within the veins from obstructing blood flow. Other sources of grafts or conduits continue to be investigated because the saphenous vein graft (SVG) has a modest rate of stenosis. In total, 15% of SVGs will become occluded within the first year because of hyperplasia and accelerated atherosclerosis and 50% are occluded within 10 years (Holzhey et al., 2012).
The left, right or both internal thoracic (mammary) arteries (IMA) are common grafts for bypassing the left anterior descending artery and right coronary artery, respectively. The left mammary artery, which has a 71% patency rate 15 years after the surgical procedure (Locker et al., 2012), is most commonly used because the use of the right mammary artery or both IMA is associated with sternal wound complications (Cahalin et al., 2011). Other arteries of the torso, such as the right gastroepiploic and inferior epigastric arteries have been employed as conduits but are not commonly used because of the difficulty in harvesting the arteries. In a large meta-analysis, it has been reported that there is an increase in long-term survival when a multiartery bypass procedure is performed when compared to left IMA and SVG, despite being more difficult surgically (Locker et al., 2012). The radial artery is also a common arterial source, but the surgeon must assure sufficient blood flow to the hand and it is a challenge to avoid sensory loss.
Traditionally, when undergoing this procedure, the patient is placed on cardiopulmonary bypass (CPB) to stop the heart and allow the CABG surgery to be completed. The CPB circulates blood to allow for full cardiopulmonary support while the heart is not beating and the grafts are sutured in place. This is a necessary procedure based upon the size and location of the coronary arteries, hemodynamic stability and left ventricular function. Unfortunately, there are adverse effects associated with CPB, particularly in the geriatric population. With the use of CPB there is a diffuse systemic inflammatory response with association of increased activation of platelets, coagulation and fibrinocytes activity. The aorta is manipulated in order to go onto CPB which is associated with increased embolic activity that increases risk for stroke and MI (Cooper et al., 2012). These adverse effects include higher incidence of perioperative or postoperative stroke, postoperative MI and deaths (Afilalo et al., 2012; Cooper et al., 2012). The following risk factors are associated with increased surgical complications and the surgeon should consider completing the CABG off CPB if medically appropriate to improve the individual’s outcomes: age, diabetes mellitus, renal insufficiency, decreased left ventricular function, peripheral arterial disease and chronic obstructive pulmonary disease (Cooper et al., 2012).
Certain risks are involved when a patient is undergoing a CABG. The surgery may be complicated by MI, arrhythmia, incisional and sternal infections, and failure to wean from mechanical ventilation, bleeding, stroke or acute renal failure. The procedure also carries a 1–3.6% mortality rate, which can be higher in patients with postoperative complications or in patients with coexisting disease like diabetes, declining left ventricular function and untreated heart failure. New onset of atrial fibrillation after open heart procedure like CABG is associated with increased length of hospital stay, and higher short- and long-term mortality rates. The older adult has an increased risk of developing postoperative atrial fibrillation, along with history of prior stroke, history of hypertension and peripheral arterial disease and longer CPB time (Kaw et al., 2011).
Minimally invasive direct coronary artery bypass
Depending upon the blood vessels that are occluded, the stability of the patient and the function of the heart, the surgeon may opt to perform the bypass through a small anterior thoracotomy, referred to as minimally invasive direct coronary artery bypass (MIDCAB). The goals of a thoracotomy approach are to decrease the surgical trauma caused by a median sternotomy, improve recovery and reduce the length of the hospital stay and can be completed without CPB support. The MIDCAB is performed through a small anterior thoracotomy incision in the left fourth or fifth intercostal space. Typically, the left IMA is the conduit of choice to bypass the left anterior descending artery.
Commonly the left internal mammillary artery (sometimes called the internal thoracic artery) is used to bypass an occlusion involving the LAD. This approach has more recently been combined with the PCI procedure which has permitted surgeons to address multivessel disease, especially for individuals who were at high complication risks for a standard CABG. This combined approach to surgically managing CAD is referred to as a hybrid procedure. The approach that is used depends on the involved arteries, heart function, stability of the conduction system, the need for cardiopulmonary bypass support and the surgeon’s training (Afilalo et al., 2012; Holzhey et al., 2012). The other benefit of a MIDCAB is that the procedure can be performed without cardiopulmonary bypass support and aortic manipulation that results in a decrease in mortality, stroke and postoperative MI (Afilalo et al., 2012; Cooper et al., 2012).
Therapeutic intervention
Patients who undergo a median sternotomy approach to cardiac surgery are commonly instructed in sternal precautions with the goal to modify the use of the upper extremities to reduce sternal wound complications. The clinician needs to be aware there is insufficient published evidence on what these precautions should be to prevent wound complications while restoring function in the early postoperative period. Sternal precautions vary from facility to facility and can be individualized for each surgeon with a facility. Commonly the precautions include weight lifting limitations, range of motion (ROM) restrictions, particularly elevation above shoulder height, and restriction in basic functional mobility and activities of daily living. There have been no published reports that have linked the performance of range of motion, the use of upper extremities, or performance of functional activities and sternal wound complications. Risk factors associated with surgical complications include obesity, surgical use of bilateral internal mammillary arteries, diabetes mellitus, prolonged mechanical ventilation and prolonged CPB time just to name a few (Cahalin et al., 2011).
With little consensus on sternal precautions, the information provided in this section is the clinical advice from this author. Commonly, the incisions are covered with only a dry dressing in the immediate postoperative phase and in the presence of a draining wound. The sternal wound may remain covered until the patient is weaned from mechanical ventilation support. If the saphenous vein or radial artery are harvested, the involved extremity will typically be ice-wrapped for 24–48 hours to control edema. Female patients with larger breasts have an increased risk of wound complication so it is advised that a bra is worn to decrease tension on the incision.
In terms of shoulder ROM, when a median sternotomy is the surgical approach, ROM is performed within the tolerance of the patient, allowing unilateral and bilateral flexion and abduction above shoulder height to tolerance. Commonly external rotation combined with abduction above 90° degrees is painful and not tolerated. If a patient has various risk factors that are associated with wound complications the surgeon or the therapist may want to consider limiting humeral elevation to shoulder height.
Acute care rehabilitation includes restoring functional mobility, increasing ambulation tolerance and preparing for discharge. Strengthening and functional mobility exercises are also performed within the patient’s tolerance, using proper body mechanics to protect the sternum and incision. Using the upper extremities for functional mobility and activities of daily living should be modified to facilitate approximation of the surgically fractured site of the sternum and reduce tissue tension.
Mobility should be initiated as soon as the patient is alert and hemodynamically stable. Out-of-bed activities may begin as early as 3–6 hours in the postoperative period, but commonly begins 12–24 hours postsurgically. Transfers out of bed are typically tolerated with a side-lying to sit transfer and using the nondependent arm to push up while using the dependent arm to splint the sternum (see Fig. 44.2). The lower extremities should not be moved off the bed until the patient is fully side-lying to reduce the activation of the abdominal muscles thus reducing tension to the inferior position of the sternal wound.
< div class='tao-gold-member'>
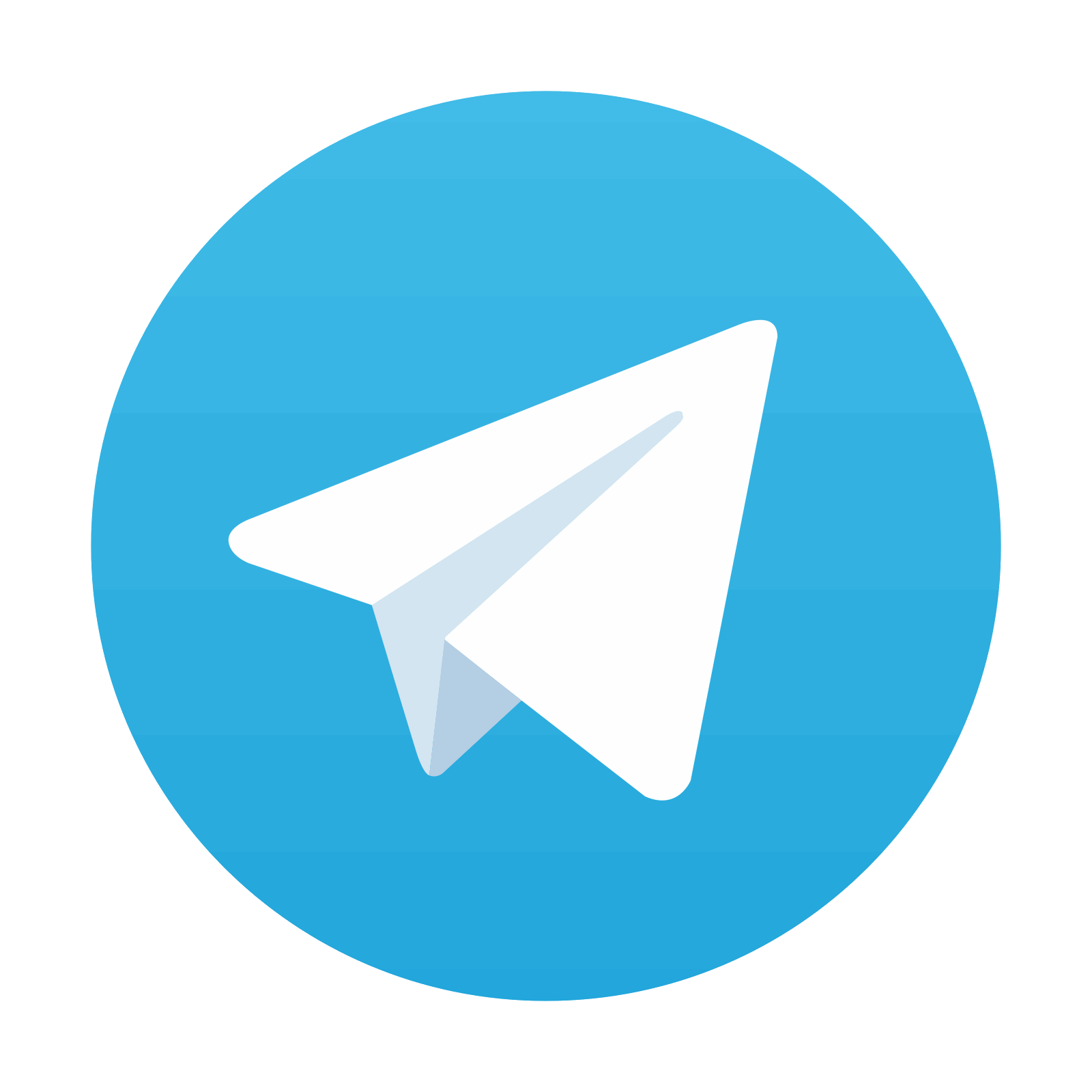
Stay updated, free articles. Join our Telegram channel

Full access? Get Clinical Tree
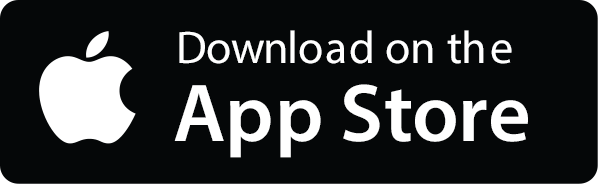
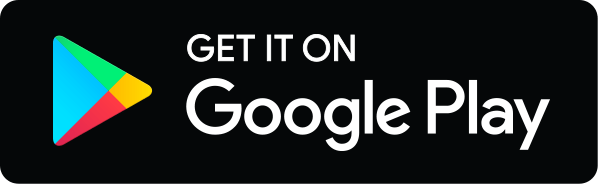