Relationships among variables that influence heat storage and ultimately exertional heat illness (EHI) risk. Activity logistics include time of day, location, and activity programming (e.g., work/rest cycles, duration, etc.). Authors assume activity logistics remain outside of an individual’s control (e.g., military and sporting activities). Bolded, modifiable EHI risk factors; unbolded, non-modifiable EHI risk factors; Tcore, core body temperature; Tambient, ambient temperature; Tskin, skin temperature
Non-modifiable Risk Factors
Many non-modifiable risk factors have been identified, with these factors falling into intrinsic or extrinsic categories. Some of these factors cannot be modified due to individual demographics and morphology, while others are limiting due to the environment and circumstances surrounding physical activity. While direct prevention strategies cannot be implemented to mitigate risk of developing EHI in individuals with non-modifiable risk factors, identification of these individuals is key. Medical providers can better prepare for potential EHI and differentially diagnose an individual with EHI when risk factors are identified through pre-participation screening.
A pre-participation examination provides vital information about an athlete’s EHI risk and should include questions and a physical exam to evaluate factors known to influence an individual’s ability to tolerate heat stress. These factors include recent exercise history, environmental conditions in which the athlete exercises to estimate heat acclimation status, previous history of EHI, medications and supplements, medical conditions, body composition, and demographic characteristics. Pre-participation examination forms including these questions have been created and successfully implemented (see Pre-participation Examination section). This screening may also include genetic screening for conditions known to elevate EHI occurrence such as sickle cell trait and malignant hyperthermia. A number of other non-modifiable risk factors exist (environmental conditions, motivation and risk tolerance, team culture, and programmed exercise demands) that may not apply to a pre-participation examination but must be considered when assessing overall EHI risk.
Programmed Clothing and Exercise Demands
There are many times in sport and physical activity during which the external demands of the activity limit individual freedom to modify activity or clothing/equipment parameters. For example, lacrosse, American football, and field hockey goalies are required to wear protective equipment such as helmets and shoulder pads. Likewise, hazardous material workers and firefighters wear fully encapsulated personal protective equipment, while law enforcement officers and military members wear extensive protective body armor to prevent physical harm. This equipment impairs heat dissipation by creating a microclimate of hot, moist air between the skin and equipment limiting evaporative cooling. Metabolically generated heat is also increased due to the added equipment mass. In these circumstances, uncompensable heat stress is more likely resulting in rapidly increasing thermal strain and EHI risk due to greater heat production and lower heat dissipation [12–14].
Work intensity is usually self-regulated during recreational activities such as running and cycling; however, this may not be true in competitive or more structured sports and physical activity. In athletics, coaches set the physical activity parameters such as exercise intensity, frequency, duration, and rest and hydration breaks. During structural fire suppression, firefighters complete standard 15–30-minute shifts before recovering. During military marches, all soldiers must complete activities in a given period to ensure a successful mission. These strict limitations leave little ability for individualization of activity parameters to meet differing thermoregulatory needs when completed in hot conditions.
The standardization of activity across all individuals completing the same tasks leads to equal absolute workload across individuals but unequal relative workloads and heat production. Less fit individuals must exercise at a relatively greater intensity, leading to unmatched workload to fitness. This discrepancy leads to greater core temperatures in unfit individuals compared to fit individuals completing the same absolute work and can be attributed to greater metabolic heat production in less fit individuals [15], possibly increasing EHI risk in these individuals. Similarly, individual hydration needs are typically not addressed by individualizing hydration breaks, leading to variable levels of dehydration, a known risk factor of EHI [8]. Rest breaks provide an opportunity to dissipate heat and consume water. Without proper work-to-rest cycles, the risk of dehydration during exercise and continual body temperature rise (uncompensable heat stress) with concurrent performance decrements exist [16].
The frequency and duration of activity in the heat represent other programmed exercise variables which influence EHI risk. During August preseason football practices, 37% of EHI occurred after 2 h of practice [3], and when practice continued beyond 3 h in length, individuals were nine times more likely to experience EHI [5]. Multiple exercise sessions on the same day and consecutive days of exercise in the heat exacerbate heat strain during the subsequent exercise session [17, 18]. While physiological mechanisms of this phenomenon warrant further investigation, epidemiological evidence indicates greater rates of EHI on the second day of exercise in the heat compared to the first day in unacclimatized football athletes [19].
Environmental Conditions
Air temperature, relative humidity, solar radiation, and wind each impact an individual’s ability to thermoregulate. Environmental conditions exacerbate heat strain by either diminishing the effectiveness of heat loss mechanisms (e.g., reduced evaporation due to high humidity, reduced convection due to low wind speed) or directly promoting heat gain which occurs when ambient temperature surpasses skin temperature. To better predict when an individual is at greater risk of developing EHI, the wet-bulb globe temperature (WBGT) can be measured, which incorporates air temperature, relative humidity, solar radiation, and wind into one numerical value. A retrospective study determined that a high WBGT (>75 °F) the day prior to an EHI was more predictive of the EHI in Marine Corps recruits than the WBGT on the day of the incident [20]. In collegiate football athletes, the greatest number of heat illnesses occurred on the second day of August preseason practice, not on the first day [19]. Fatal exertional heat stroke cases in American football athletes competing in cooler parts of the United States most commonly occurred when the WBGT was higher than average for that region [21]. Both epidemiological [19–21] and lab studies [17, 18] indicate that environmental conditions are a risk factor for EHI and that there is a residual effect of the environment among consecutive days of exercise in the heat.
To offset the impact of environmental stress on thermoregulation, behavioral responses such as reducing exercise intensity or duration, removing clothing/equipment, and moving indoors or to a shaded area are effective heat stress mitigation strategies [11]. Unfortunately, certain populations such as occupational workers, military personnel in forward deployment, and athletes during competition or practice may not be able to respond in these manners increasing EHI risk.
The autonomic heat loss mechanisms of skin blood flow (convective) and sweat evaporation are effective at transferring heat to the environment provided there is a favorable gradient between skin and air temperature and water vapor pressure (i.e., ambient temperature is lower than skin temperature). Convective heat loss becomes negligible when ambient temperature surpasses skin temperature, and under these conditions sweat evaporation is the primary means of heat loss [22]. When the amount of moisture in the air increases, more sweat must be present on the skin to increase water vapor pressure around the skin enabling sweat evaporation. If fluid intake is not matched with sweat rate, this higher sweat production requirement results in fluid and electrolyte loss leading to dehydration-induced cardiovascular strain [23], impairments in thermoregulation [24, 25], and challenges blood pressure regulation [23, 26]. In particularly humid conditions, maximal sweating rates may be insufficient to overcome the water vapor pressure gradient such that evaporative cooling requirements are not met yielding uncompensable heat stress. Consequently, body temperature continually rises, and EHI risk is extremely high. Interestingly, even at 100% relative humidity, the body has some evaporative potential as long as air temperature remains below that of the skin and there is airflow across the skin.
Air movement created naturally, artificially (e.g., fan), or by activity (e.g., running, cycling) enhances the ability to cool via convection and evaporation. In the absence of adequate sweat production, air movement increases heat storage in extreme heat in older individuals with lower sweat evaporation [27, 28].
Age
There is a wealth of knowledge on the impact of age on heat balance during exercise in the heat. Middle aged and older men have reduced sweat rates compared to younger men exercising in the heat [29]. Older adults also exhibit reduced skin blood flow possibly stemming from lower stroke volume and cardiac output compared to young individuals [30, 31]. Unsurprisingly during exercise in the heat, older men and women have greater heat storage than their younger counterparts [31–33]. The decrement in evaporative heat loss exists even when age-matched for aerobic fitness and physical characteristics [33]. In older men, this reduced evaporative heat loss is even greater in untrained individuals, but this reduction can be minimized by improving aerobic fitness [34]. Combined, these studies indicate that older individuals gain heat at a faster rate than younger individuals, likely due to reduced evaporative ability. Considering that evaporation accounts for the majority of heat loss during exercise in a hot environment, older individuals are likely more susceptible to EHI when completing equal work to their younger peers.
Contrary to previous beliefs, children are not at a greater EHI risk than adults due to physical characteristics. When hydration is properly maintained, and accounting for differences in body size and exercise intensity, thermoregulatory ability [35, 36], cardiovascular capacity, exercise economy [37], and tolerance between adults and children are similar [38]. The greater body surface area to mass ratio of children is expected to be advantageous to heat loss during exercise except when environmental conditions are extremely hot. Aside from poor hydration practices, the incidence of EHI in youth sport [6] is thought to be related to other modifiable risk factors. These include improper programmed exercise and clothing/equipment requirements, unmatched physical activity to fitness, undue exercise duration, use of exercise as punishment, and insufficient recovery from activity (improper work-to-rest cycles or rest between exercise sessions [two-a-day practices]) during activity in the heat [38].
Sex
Differences in temperature regulation between men and women do not stem from inherent differences between sexes but instead are attributed to differences in aerobic fitness (modifiable) and physical characteristics including body mass and surface area (non-modifiable) [39]. The impact of anthropometrics and aerobic fitness on the predisposition of developing EHI will be discussed later in this chapter. However, because females typically have lower absolute VO2max than men, when exercising at a similar absolute work intensity (e.g., 7.5 mph), females will have a greater metabolic heat production, leading to a greater core temperature increase. In situations such as military drills or occupational tasks when men and women must complete work at the same absolute work intensity, this could possibly predispose women to EHI.
Most published research on EHS patients involves men which may be due to a variety of factors. First, many cases of EHI occur in the military, causing this population to be greatly studied, most of whom are men (males represent 79–86% of enlistees across the army, navy, and air force, and 92% in the marines). Secondly, men more often than women elect activities that result in EHI (e.g., American football, military, occupational work). Lastly, in situations with high EHI potential, men may more likely push themselves to greater levels of hyperthermia due to greater work intensities, although this is yet to be fully explored. When studied more systematically through epidemiological research, accounting for all sports, US high school male athletes have a greater rate of developing EHI than female athletes [3]. However, when football-related EHI were excluded and only those sports in which both girls and boys participate were included, EHI rates were similar between sexes.
Anthropometrics
Differences in body size and composition affect thermoregulation by way of affecting the physical process of heat exchange. This is accomplished by differences in body fat, body surface area to mass ratio, and body weight [3, 40–42]. Body surface area determines the heat exchange area with the environment for both dry (convective and radiative) and wet (sweat evaporation) heat loss and thus contributes to overall heat storage. A high body surface area to mass ratio is usually beneficial except when environmental conditions are extreme and impede heat loss.
As body weight increases, so does the metabolic demand involved in weight-bearing locomotion. In other words, greater metabolic heat is generated by heavier athletes completing the same absolute workload as lighter athletes. Body mass and body composition also determine heat storage capacity as the specific heat of fat mass (2.97 kJ·kg−1 °C−1) is 20% less than the specific heat of fat free mass (3.66 kJ·kg−1 °C−1) due to water content differences. This means that fat-free mass can store more heat than fat mass. This is relevant with passive heat exposures, or when heat loss is limited and body temperature increase is determined by storage capacity. Also, at fixed metabolic heat production rates, those with equal mass but higher body fat percentage will gain heat faster possibly because of lower mean specific heat capacity or impaired sweat gland function [41]. It is also documented that larger individuals produce lower self-generated airflow [43], decreasing the potential for heat loss. While body fat usually adds to body mass increasing metabolically generated heat, cutaneous fat is well perfused and in weight-matched subject’s whole body and local sweating responses as well as skin surface, heat dissipation is not affected by body composition [41]. In summary, anthropometric differences in heat production, heat storage, and self-generated airflow may explain why larger individuals (males/females, BMI ≥ 29.5/25.7 kg/m2, body fat ≥28.8%/34.9%, body surface area ≥2.0/1.7 m2) are at greater EHI risk [3, 6, 40, 42, 44].
Motivation, Culture, and Risk Tolerance
The willingness to succumb to intense discomfort in anticipation of an achievement or reward is an acknowledged EHI risk factor. For example, the psychological and physiological feedback of exercise (e.g., pain, discomfort, sensations of hotness, or dehydration) may be overshadowed by intrinsic or extrinsic motivators or some combination of both. Regardless of the motivator(s), the desire to complete a task or obtain an achievement or award drives metabolic heat production which may exceed heat loss capacity and increase heat storage, especially when environmental conditions are warm to hot.
It may be initially difficult to identify overzealousness as motivators among individuals vary widely. For example, a strong internal motivation to “make the team” or secure a starting position may be present in transfer or underclassmen athletes, whereas top-performing athletes may push themselves to achieve new personal bests. Others may be highly influenced by external motivators such as peers, rivals, coaches, or an audience. In the military, never married cadets had a greater incidence of severe EHI compared to married counterparts [42]. While the reason(s) for this relationship is unknown, it may be due to the differences in risk tolerance between married and single cadets.
In recent years, a number of American EHS deaths have occurred resulting in litigious investigations. A culture of not reporting EHI signs or symptoms, inaction upon knowledge of EHI symptomology, and/or using physical activity as punishment have been commonalities among many lawsuits representing risk factors associated with EHI and EHS. If modification of these risk factors is to occur, administration, coach, and athlete EHI education, communication expectations, and cultural awareness are necessary.
Peer, Coach, and Organization Pressure
Individuals may feel perceived pressure to continue exercising to keep up with their peers or opponents, particularly when they are competing to make the team, secure a starting position, finish in a certain time, or win a race. This may lead to unmatched exercise intensity to fitness levels and/or a reluctance to report symptoms of EHI to their coaches or superiors. These issues further exacerbate the risk of developing EHI. Similarly, youth athletes may perceive pressure to impress parents or coaches and not “let them down,” a phenomenon reported in youth hockey, wrestling, and soccer [45–47]. This thought process leads to the athletes continuing to exercise at a relatively greater intensity than peers with a higher fitness level or for a longer duration, leading to greater thermal strain and risk of EHI.
Medications
Selected medical and recreational drugs that may alter exertional heat illness risk
Drug class | Proposed mechanism |
---|---|
Anticholinergics | Impaired sweat gland function |
Antidepressants | Increased heat production |
Antihistamine | Impaired sweat gland function |
Beta-blockers and calcium channel blockers | Impaired CO increases |
Diuretics (antihypertensive) | Depletion of fluid and electrolytes |
Phenothiazines | Impaired sweat gland function Altered hypothalamic function |
NSAIDs | Increased gut and liver toxicity |
Sympathomimetics | Increased heat production Impaired sweat gland function Increased heart rate and blood pressure |
Tricyclic antidepressants | Increased heat production |
Recreational drugs | |
Alcohol | Diuretic Peripheral vasodilation |
Ephedrine | Increased heat production Impaired sweating |
Ecstasy (MDMA) | Increased heart rate and blood pressure Increased muscle tension Increased sweating or chills |
Cocaine | Decreased sweating threshold Decreased skin blood flow threshold Attenuated perception of thermal sensation |
Medical Conditions
Skin disorders | Sweat gland disorders | Cardiovascular diseases | Metabolic disorders | Thermoregulatory disorders |
---|---|---|---|---|
Sunburn Ectodermal dysplasia Psoriasis Miliaria rubra Substantial scarring Therapeutic radiation | Cystic fibrosis Chronic anhidrosis Substantial scarring Diabetes I and II | Heart failure Cardiomyopathy Myocarditis Hypertension | Hyperthyroidism | Malignant hyperthermia Insomnia Febrile events (fever, streptococcal pharyngitis, mononucleosis, etc.) Previous EHI |
Febrile Events
Current or unresolved illness (cold, fever, infection, etc.) may predispose an individual to EHI, especially EHS, and can be rationalized from three perspectives. First, pyrokines such as IL-1 and TNF increase the hypothalamic core temperature set point to fight infection [52]. The elevated resting body temperature means there is less capacity to increase core temperature before reaching dangerously high internal temperatures (>104 °F) [8]. Second, the risk of dehydration is higher due to excessive fluid loss (diarrhea) or lack of fluid replacement resulting in dehydration or hypohydration-induced impairments in cardiovascular and thermoregulatory function (see Hydration). Third, an individual may reside in an immunocompromised state limiting their ability to rapidly resolve the systemic inflammatory response characteristic of EHS [7]. A prodromal viral illness leading to altered gene expression and unchecked inflammatory pathways has also been purported [53]. Exercise in the heat should be avoided until an individual is fully recovered from febrile events.
Previous History of Heat Illness
In a recent epidemiological study of military cadets examining 427,922 person-years, the risk of heat-related cramping, syncope, or exhaustion was fourfold higher in cadets with a previous EHI [42]. Alarmingly, the risk of EHS was almost doubled when cadets reported a previous EHI [42]. The occurrence of an EHI may underscore the inability of certain biological systems (thermoregulation, fluid-electrolyte, cardiovascular, inflammation) to manage the additive stressors of exercise and heat. On the other hand, after EHS, cellular damage may precipitate impaired biological responses to exercise in the heat eliciting a heat-intolerant individual which may be transient or persistent [54].
Whether heat intolerance is a within-lifetime maladaptation or genetically founded (e.g., malignant hyperthermia, TLR4 polymorphisms) is debated [55]. Associational but not causal links between malignant hyperthermia and EHS are published [56, 57]. A small percentage of the population is thought to be heat intolerant, but through heat acclimation, tolerance to exercise-heat stress is markedly improved [58]. Thus, while previous EHI may indicate genetic predisposition or inefficient biological functioning in response to exercise-heat stress and increase EHI risk [55], adaptation to exercise-heat stress (heat acclimation) improves thermoregulation, fluid-electrolyte, cardiovascular, and cellular (heat shock protein) function leading to a more heat-tolerant individual [59].
Sickle Cell Trait
Sickle cell trait afflicts 8% of African-Americans and increases exertional collapse risk, a primary cause of which is thought to be heat-related injury and death. The strength of this association varies across studies with some reporting no association [42], while others show a strong relationship in military (33% increased EHI risk) [60] and athletic populations [61]. The exact link between sickle cell trait and EHI risk is unclear. It is likely that environmental conditions are a mediating variable in this relationship as 87% of sickle cell trait deaths occurred when ambient temperature exceeded 80 ° F [61]. Environmental heat stress can exacerbate sweat-induced dehydration increasing the potential for sickle cell vaso-occlusion and exertional collapse.
Spinal Cord Injury
As wheelchair sports continue to grow in popularity, understanding heat exchange and EHI potential in the paraplegic and quadriplegic athlete is crucial. As a consequence of spinal cord injury, heat production (muscle mass) and loss (blood redistribution and sweating capacity) are reduced below the level of the spinal cord lesion. However, heat balance remains a function of heat gain vs. loss as in able-bodied athletes. In both cool (15–25 °C) and warm-to-hot (25–40 °C) conditions, paraplegic athletes demonstrate similar core body temperature responses as able- bodied athletes [62]. However, it is consistently observed that lower body heat storage was greater in wheelchair athletes, possibly due to reduced lower body and, therefore, whole-body sweat rates. Quadriplegic athletes consistently show exacerbated heat gain due to the severe reduction or absence of sweat evaporation to dissipate environmental and metabolic heat stress during continuous exercise. Taken as a whole, wheelchair athletes present with potentially greater EHI risk due to elevated heat storage and reduced sweat rates below the level of the lesion.
Skin and Sweat Gland Disorders
Healthy skin contains a vast network of capillary beds and eccrine sweat glands. Damage to the skin from direct trauma or scarring could result in the inability to create sweat (anhidrosis) or adequate perfusion to dissipate heat through evaporation and convection. Chronic inflammation, genetic, neurologic, medicinal, and idiopathic etiologies underscore most disorders of the skin and sweating. Trauma may be mild and transitory such as a grade 1 sunburn, but even a mild sunburn has been shown to diminish local sweat production [63]. On the other hand, severe burns result in scarring and fibrotic tissue absent of sweat glands. The risk of EHI increases with the amount of scarring as sweat evaporation is negligible. Importantly, well-healed skin grafts can effectively increase blood flow and sweat production during exercise-heat stress [64]. Inflammatory skin disorders such as large rashes, psoriasis, and eczema disrupt skin vasodilation and potentially heat exchange.
A common complication of diabetes is peripheral neuropathy which could impair neural control of vasomotor and sudomotor function. Early research has shown that in type 1 diabetics, local cutaneous vasodilation [65] and sweating [66] were impaired at rest without neuropathy. However, recent work demonstrated only at moderate-to-intense cycling in the heat was whole-body sweat rate reduced in type 1 diabetics [67] and whole-body heat loss lower in type 2 diabetics [68]. In both studies greater exercising body temperature, thermal strain, and EHI risk were noted, largely due to reductions in evaporative heat loss.
Cystic fibrosis is an autosomal recessive trait disease that alters sweat gland transmembrane ion trafficking resulting in difficulty regulating sweat sodium losses. The risk of cramping and hyponatremia is elevated during exercise in the heat as greater sweat evaporation is necessary to regulate body temperature, at the expense of excessive sodium loss [69]. Mutations in genes coding calcium channels such as ryanodine receptor 1 responsible for calcium trafficking during skeletal muscle contraction underscore the life-threatening phenomena known as malignant hyperthermia [70]. This condition occurs in operating rooms when exposed to certain anesthetic agents resulting in unregulated calcium cycling causing muscle rigidity, rhabdomyolysis, and extreme hyperthermia. Death occurs without rapid dantrolene administration. While malignant hyperthermia and EHS are both deadly and arise as a result of excessive metabolic heat production, evidence to suggest a causal link between the two conditions is nonexistent.
Modifiable Risk Factors
Lack of Heat Acclimation
Heat acclimation, or the lack thereof, is arguably one of the most influential predisposing factors for EHI. Growing evidence from lab, field, and climatology studies demonstrate that EHI risk is greatest during unseasonably hot weather [71] and the initial 1–3 days of exercise-heat exposure [2, 17, 19] when heat acclimation adaptations have yet to be established. The first day of work in the heat appears to exacerbate next-day fatigue, thermal sensation, and body temperature increases [17, 18], possibly leading to greater EHI risk. Heat acclimation diminishes EHI risk due to enhancements in fluid-electrolyte balance (conserved sweat electrolytes, higher sweat rate and sensitivity), cardiovascular stability (expanded plasma volume, lower exercising heart rate), increased skin blood flow, and lower resting and exercising core body temperature, summating in a more heat-tolerant individual [72–74]. Several practical reviews outline best practices for heat acclimation induction [72, 75] and maintenance [73] in athletes. The effectiveness of heat acclimation in lowering EHI occurrence was recently demonstrated in high school American football. In schools that implemented preseason heat acclimatization guidelines [76], 55% less EHI were observed [77].
Low Physical Fitness
Low physical fitness has been cited in EHI/EHS case reports [1] and epidemiological studies [42, 78] as a predisposing risk factor. Conversely, developing physical fitness and increasing VO2max are associated with reduced physiological and thermal strain during exercise-heat stress [79–82]. Trained athletes exercising at the same relative intensity as untrained individuals do so at a higher metabolic rate (i.e., absolute workload) generating more heat [83], even in cool environments. Thermoeffectors respond to dissipate the metabolically generated heat stress and over time result in key adaptations. Adaptions observed in well-trained individuals include lower resting and exercising HR and core temperature, expanded blood volume, greater sweat rate and sensitivity, and greater exercise-heat tolerance among others [59, 84], consummate with a “quasi-heat-acclimated state. ” However, to optimize thermal tolerance, performance, and safety in the hot conditions, heat acclimation is required. It should be noted that VO2max is partially genetically determined [85] and a moderate-to-high VO2max may occur in the absence of robust physical training. Therefore, it is believed that recent aerobic training and the environment of this training, rather than VO2max alone, are more informative when relating aerobic fitness, the quasi-heat-acclimated state, and EHI risk.
Hydration
Water is essential for life, serving as a cell volume regulator, transport and chemical reaction medium, and mediator of thermoregulatory and cardiovascular function. Regarding EHI risk, fluid balance is implicated as a major predisposing factor [7]. Progressively greater levels of hypohydration or dehydration result in proportionately faster increases in body temperature and heart rate [86, 87]. For every 1% body mass loss due to dehydration, body temperature and heart rate increase 0.16–0.4 °C and 3 bpm, respectively, faster than euhydrated counterparts in hot environments [88–90].
The greater rise in body temperature is a function of dehydration-induced impairments in dry (skin blood flow) and evaporative (sweat) heat loss mechanisms. Specifically, as the degree of dehydration increases, progressive decrements in sweat rate, sensitivity, and onset are observed during exercise in the heat [91, 92]. Similarly, skin blood flow volume and sensitivity are diminished when dehydrated [93]. Shunting blood from thermoeffectors serves to limit further (a) fluid losses from sweating and (b) reductions in central blood volume to sustain mean arterial pressure [23, 26], at the expense of heat loss productivity.
From a performance viewpoint, dehydration (>2% body mass loss) consistently yields impaired cognition, sporting skills, aerobic, strength, power, and repetitive high-intensity performance [94–97]. Importantly, the aforementioned thermoregulatory, cardiovascular, and performance impairments demonstrate a dose (dehydration)-dependent response suggesting interventions to minimize dehydration are crucial not only to prevent dangerously high body temperature and EHI but also to maximize performance in hot environments.
Alcohol, Sleep, and Supplements
Alcohol, sleep, and supplements are factors that influence EHI risk through modulation of heat loss, hydration status, and metabolic rate, for which we know very little. Among the available evidence, it appears that alcohol influences autonomic thermoregulation (impaired vasomotor reflexes, core temperature set point) and perceptions of hotness during heat exposure [98, 99]. Beverages containing ≥4% alcohol by volume elicit diuretic effects by inhibiting vasopressin activity in active populations and should be discouraged for fluid replacement [100, 101]. Diuretics (alcohol, hypertensive medications, weight loss supplement, etc.) pose severe consequences to individuals engaging in exercise in the heat due to the potential of dehydration-induced impairments in thermoregulatory and cardiovascular functioning (see Hydration section).
Whether altered sleep (deprivation or interrupted) leads to impaired thermoregulation and increased EHI risk is unclear. In two case series, 5/6 fatal EHS [1] and 7/10 EHS [54] patients experienced some degree of sleep disturbance the nights preceding the event. Beyond case series, lab studies show conflicting evidence regarding the effects of sleep on thermoregulation. Some sleep deprivation studies (27–60 h awake) show lower sweat sensitivity [102] resulting in higher exercising core temperature [103]. Decrements in sweat rate and peripheral vasodilation have also been reported following sleep deprivation, albeit this observation is not consistently seen across the literature [103–105]. Moreover, there is evidence indicating disturbed sleep results in lower pre-exercise body temperatures and does not affect exercise-induced body temperature responses [106–108].
Markers of systemic inflammation [109] and the stress hormone cortisol [110] are greater after sleep disturbance and long sleep duration, but not short sleep duration. This sleep-induced pro-inflammatory state may compromise an individual’s ability to quickly resolve the systemic inflammatory response characteristic of EHS [7]. Altered sleep increases submaximal heart rate up to 5%, demonstrating a reduction in cardiovascular efficiency and capacity to adjust to exercise-heat stress [107, 111]. The interrelationships among sleep modification, thermoregulation, and overall health warrant future research given the frequency of interrupted, restricted, and deprived sleep in today’s society. Whether altered sleep is conclusively linked to EHI risk is debatable. However, considering altered sleep appears to affect some more than others, for those wishing to optimize safety during physical activity in the heat, attention to adequate sleep quality and quantity is warranted.
The use of recreational drugs and some supplements before or during exercise in the heat are cause for caution due to the lack of FDA oversight and questionable business practices of some manufacturers. While many individuals consume supplements for purported ergogenic effects, some supplements could influence thermoregulatory effectors and fluid balance elevating EHI risk (see Table 3.1). When consumed within reported ergogenic ranges, the popular supplements creatine and caffeine do not impair fluid balance or thermoregulation during exercise [112, 113]. It has been purported that creatine may actually improve thermoregulation by increasing the water content of fat-free mass and therefore heat storage capacity [113]. In military enlistees, tobacco, opioid, and NSAID use increased relative risk of mild and severe heat-related injury [42]. Cocaine was shown to diminish the onset of skin blood flow and sweating leading to elevations in core body temperature while also attenuating the perception of thermal discomfort [114]. Heavy use of over-the-counter medications, recreational drugs, and certain supplements should be cautioned and individualized when exercising in the heat. Medicinal substances should be taken with oversight from trained healthcare providers to ensure safety.
Heat Illness Mitigation Strategies
There is great inter- and intraindividual variation in EHI risk factors that modify an individual’s physiological and psychological tolerance to heat stress. Implementing prevention strategies addressing every foreseeable risk factor is very challenging in team-based sports but may be warranted on an individual basis in those with the highest EHI risk. Importantly, EHS risk may be mitigated by using evidenced-based approaches that can be modified at the team and individual level to address specific athlete concerns. These interventions include heat acclimation, increasing fitness level, ensuring proper hydration, cooling before and during exercise, as well as modifying the physical activity or exercise setting.
Heat Acclimation
Acute exposure to hot and/or humid ambient conditions results in the development of thermal strain (i.e., elevated skin, muscle, and core temperatures) during prolonged exercise, which impairs performance and increases the risk of EHI. However, when repeatedly exposed to conditions that elicit profuse sweating and elevate whole-body temperature, adaptations develop that reduce the deleterious effects of heat stress. Heat acclimation and acclimatization, which refer to periods of heat exposure undertaken in artificial (i.e., laboratory) or natural (i.e., outdoor) settings, respectively, lead to improved submaximal exercise performance, increased VO2max, and improved thermal comfort in the heat, as well as reduced susceptibility to EHI. These benefits are achieved through enhanced sweating and skin blood flow responses, plasma volume expansion, better fluid balance (i.e., hydration) and cardiovascular stability, eliciting acquired thermal tolerance [72, 73, 115].

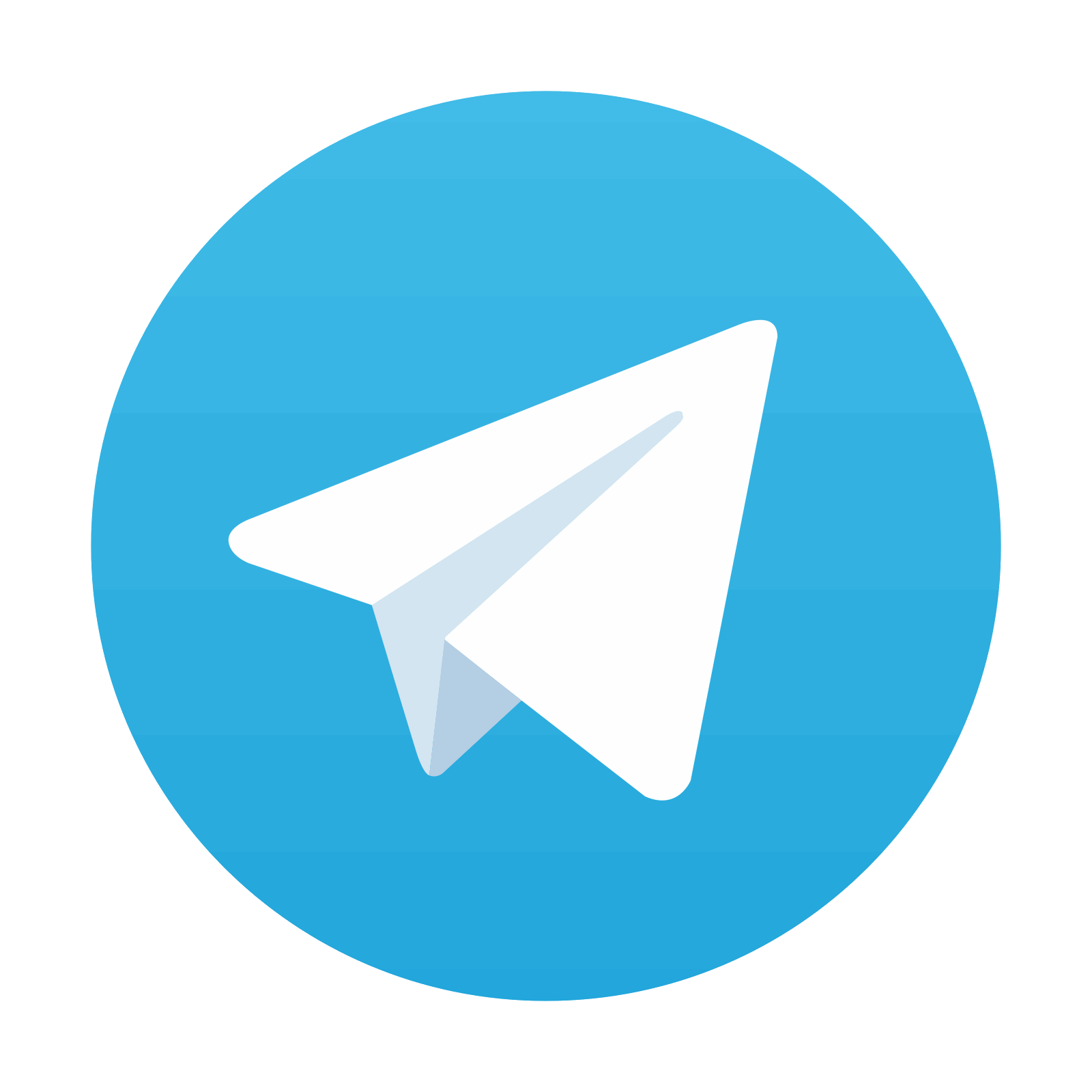
Stay updated, free articles. Join our Telegram channel

Full access? Get Clinical Tree
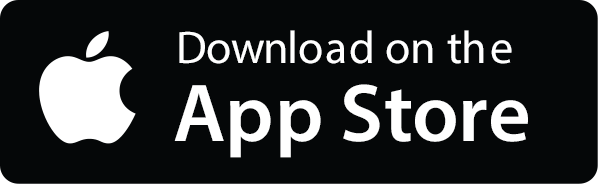
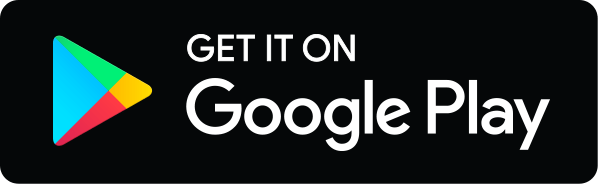