A summary of the potential sources of heat stress, the resulting effects of that heat stress (i.e., heat strain) and the interindividual factors (green circles) and intraindividual factors both within (red circles) and beyond (blue circles) the individual’s control that may modify an individual’s response to a given level of heat stress. Heat stress represents the combined heat load from the environment, clothing, and heat generated from metabolic processes, while the resulting physiological, psychophysical, and performance effects characterize the heat strain response. Excessive and/or prolonged heat strain can progress to heat-related illness or death without appropriate monitoring
In addition to these interindividual factors, individual variation in thermoregulatory function during heat stress can arise from day-to-day and within-day variation changes both within (e.g., including acclimation and hydration state and caffeine, alcohol, and medication use, among others) and beyond a person’s control (e.g., heat exposure duration, acute illness, consecutive work shifts, shift duration, and illness, among others). For instance, it is well established that repeated heat exposure or exercise in hot environments can confer thermoregulatory adaptations [105], with these changes being recently shown to enhance whole-body evaporative heat loss as a function of the exercise-induced heat load, and thus, markedly reduce body heat storage during exercise in the heat [106, 107]. Conversely, during exercise-heat stress, fluid consumption that is insufficient to offset sweat losses can result in hypohydration, which can exacerbate body heat storage by attenuating sweat production, and thus, whole-body evaporative heat loss [108]. Further, workers in many industries (e.g., mining, electric utilities, others) often perform consecutive days of prolonged (~10–12 hours), strenuous work in the heat. These conditions cause considerable heat strain [84], which can cause carry-over effects that impair whole-body heat loss, and, thus, exacerbate body heat storage and the resulting changes in heat strain in older adults on the next work day [109], with those effects potentially worsening over two or more work days [110]. However, while we have markedly improved our understanding of these intraindividual factors over recent years, we know relatively little about the independent effects of other common factors during heat exposure or physical activity in the heat, including sleep deprivation and alcohol and medication use, among others. Given that many of those factors have been shown to modulate local heat loss responses of skin blood flow and sweating [111–113], examining the integrated effects of these changes on whole-body heat exchange and body heat storage represents an important area of future research.
Up until this point, emphasis has been directed to the inter- and intraindividual factors known to independently modulate whole-body heat exchange when examined in isolation. While that research has improved our understanding of the independent effects of those factors, we possess only limited knowledge of the interactive effects of multiple inter- and/or intraindividual factors. Such interactions may exert an additive effect on heat strain, while others may reduce the additional heat strain associated with a given factor. For instance, it is known that sedentary middle-aged adults display age-related reductions in whole-body evaporative heat loss relative to young adults, whereas aerobically trained middle-aged adults display a similar capacity to dissipate heat compared to their young counterparts [44]. As another example, recent work has demonstrated that sweat-induced hypohydration (~4% body mass loss) causes marked reductions in evaporative heat dissipation (via sweating), likely to conserve fluid to support blood pressure regulation [114], which exacerbate body heat storage during exercise-induced heat stress in in young, but not older adults [108]. Since both the general and working population are heterogeneous for multiple interindividual factors, improving our understanding of the interactive effects of these intraindividual factors is critical for explaining individual variation in heat exchange and the resulting changes in heat strain during heat exposure and physical activity in the heat.
Heat and Impact on Population Health
High ambient heat has been associated with adverse impacts on health which include a worsening of preexisting health conditions (e.g., cardiovascular, respiratory, and renal illnesses; diabetes; mental health issues). Due to an insufficient ability to thermoregulate, children are particularly vulnerable during extreme heat events. The degree to which people are negatively affected by an extreme heat event is to a large extent dependent on the frequency and duration of heat of the event. Moreover, the severity and extent of health effects associated with extreme heat events are unique to the human, societal, and environmental circumstances at the time and location where the heat event occurs. This complex set of factors can moderate or exacerbate health outcomes and vulnerability in the affected people and communities.
Having strong adaptive capacity contributes to heat-resilient people and communities—the ability to prepare and plan for, absorb, recover from, and more successfully adapt to adverse events. During extreme heat events, heat-related morbidity and mortality is especially elevated in people with low adaptive capacity and that have difficulty responding or relocating when necessary. As discussed in the following section, heat-health relationships are complex, and vulnerability to heat varies as a function of nonphysiological (e.g., geographical location, urban density, building design) and physiological (e.g., natural aging, state of health) factors.
Heat and Human Health
A recent report concluded that the threat to human life from excess heat now is inevitable [50]. Extreme heat causes some of the highest death tolls among all natural weather hazards [12, 13] dramatically increasing short-term mortality in vulnerable populations (i.e., children, the elderly, the physically challenged or the sick, deprived individuals, others) [14, 15]. Change of 0.7–3.6% in all-cause mortality has been reported for a 1 °C increase in average daily temperature during hot weather [115]. In addition to the above groups, people living at home alone as well as deprived individuals show increased mortality rates during heat waves [14, 116–124], which highlights the key roles of isolation and socioeconomic factors on the vulnerability to heat waves [119].
The dramatic effect of heat on human health was revealed during a major heat wave in Chicago in 1995. Record high temperatures of 41 °C resulted in 739 heat-related deaths over a 5-day period primarily in the elderly, especially those without air conditioning or those who were unable to open their windows due to fear as a result of the unprecedented increase in crime [125]. Additionally, thousands of people, including the young, were hospitalized with heat-related illnesses. In 2003, a large-scale catastrophic effect of extreme heat on population health occurred when between 30,000 and 70,000 premature deaths were recorded in Europe during a 2-month heat wave (average temperature ≥30 °C; peak temperatures >40 °C) [126]. As in the case of the Chicago heat wave, overheating in homes caused wide-scale fatalities in the 2003 European heat wave [126]. There were 55,000 excess deaths during a heat wave in Russia in 2010 [127].
The Temperature-Mortality Relationship

Schematic depicting the relationship between outdoor temperature and human health. Assessment of the thermal environment on temperature-attributable mortality serves as a valuable tool to assess the acute health effects of heat exposure that fluctuate over time. As exemplified by the white and orange tracing, the temperature-mortality relationship is not constant and can vary as function of geographical location, climate type, seasonal influences, as well as population groups (e.g., older adults, individuals with chronic disease, others) (adapted from 130)
Heat Health and Aging
As mentioned in the subsection above (Inter- and Intraindividual Determinants of Heat Strain), vulnerability to heat depends on climate factors such as the frequency of heat waves, but it is also dependent on individual risk factors (e.g., age, sex, health status, etc.; see Fig. 12.1) [139, 140]. The impacts of heat are disproportionately borne by older adults and individuals with type 2 diabetes [123, 141, 142], especially those with associated comorbidities such as obesity [143, 144] and/or cardiovascular disease [120, 123, 142]. The issue of aging and its implications on heat tolerance and thermal comfort has become an important concern especially with our aging population and climate change. A growing body of evidence indicates that the ability of humans to manage heat stress progressively decreases with age [38, 40]. Individuals over the age of 65 years are among those most at risk for adverse heat-related events [14], as evidenced by an increased risk for hospital/emergency department admissions [29, 138, 142] and death [118, 121, 123, 145] during extreme heat events compared with younger populations. These findings are attributed to social and community factors [119], as well as to marked reductions in the ability of older individuals to dissipate heat [39, 40, 44, 48]. Recent evidence even suggests that this impairment in thermoregulation can be detected as early as 40 years of age [38] and, by the age of 55 years, it leads to a substantially increased risk for hyperthermia when working or exercising in the heat [140]. The impact of this decrement in heat loss capacity also appears to be dependent upon the heat load such that the magnitude of difference in heat loss as a function of age increases progressively along with increasing levels of heat stress [39, 44].
Heat Health and Chronic Disease
Chronic health conditions commonly associated with aging such as obesity, diabetes, hypertension, and cardiovascular disease have also been shown to independently diminish one’s capacity to regulate core temperature during heat stress. Obesity was a comorbidity in the 2003 European heat wave [146], which supports previous findings showing that fatal heat strokes occur at a rate of 3.5 times higher in those who are obese or overweight than in those of normal weight [147]. Furthermore, those who have diabetes suffer disproportionately during extreme heat events compared with the general population [14, 148]. Type 2 diabetes has been linked to impairments in sweating and skin perfusion [148]. As a consequence individuals with type 2 diabetes have a reduced capacity to dissipate heat and therefore a compromised ability to regulate core temperature during heat stress [49]. Moreover, the magnitude of those impairments is exacerbated by complications from diabetes (e.g., peripheral and autonomic neuropathies, poor glucose control, others) [148].
While there remains a paucity of information regarding the extent to which individuals with cardiovascular disease may have an impaired capacity to dissipate heat, some links have been found between increased mortality during heat waves and the presence of cardiovascular diseases [14, 125, 149]. For example, mortality in those who had cardiovascular diseases was 30% higher during the 2003 European heat wave than during other “normal” heat days [149]. Cardiovascular disease was also prominent among health conditions ascribed to the excess mortality recorded during the 1995 Chicago heat wave [125]. Additionally, hypertension was a common comorbidity factor in those who died from heat effects during the Chicago 1995 heat wave [150].
Heat Health and Children
Children are regarded as a population group that is especially vulnerable to the effects of heat and extreme heat events. Children are usually defined as humans under 18 years of age [151]. There are several physiologic differences between children and adults (e.g., higher surface area-to-mass ratio, lower sweat rate, smaller blood volume, others) that could explain a higher vulnerability to heat [152]. Surprisingly, only a few studies have examined the association of heat and mortality among young children [153]. Using different age ranges, some studies have found a relationship [153–155], while others have observed no association [156, 157]. According to the most conservative estimates, children under 14 have a 1.5-fold greater risk of experiencing a heat-related fatality as compared to young healthy adults [158].
Despite the growing evidence that heat-related morbidity and mortality is elevated in children, relatively little is known about the physiologic responses of children exposed to heat stress conditions. Inbar and colleagues were among the first to suggest that children’s thermoregulation differed markedly from that of adults. This was evidenced by their observation that children demonstrate a reduced tolerance to heat [159]. A number of other studies showed similar reductions in heat tolerance in children [160, 161] albeit responses have been variable [161–163]. It was also demonstrated that the process of maturation, and not only physical growth, may modulate thermoregulatory function in children [164–166], although the mechanism(s) underpinning this response has yet to be elucidated. This is likely associated with multiple factors related to morphologic, metabolic, and cardiovascular differences [167].
Heat Health: Social and Community Determinants
Epidemiological studies examining trends in heat-related mortality have noted in several instances that there is an unequal distribution of morbidity and mortality among the various socioeconomic groups [118, 141, 168–170]. Studies show a greater risk of mortality was associated with lower levels of education [168, 171] and lower income (includes living in poverty and poor housing) [121, 130, 170]. A direct inverse correlation has been found between income and education and the rates of obesity, hypertension, cardiovascular disease, and type 2 diabetes [172–178]. Those with higher income and education are also more likely to be physically active [179–181], adding an element of protection against heat injury and illness. In addition, those in lower socioeconomic strata are often at a disadvantage in terms of self-care due to the costs of monitoring and treatment that may not be covered by health insurance. As a result, the socioeconomically disadvantaged often have lower treatment compliance rates and higher rates of long-term disease-related complications [182–184]. This, in turn, would make them even more susceptible to heat-related injury and illness when exposed to hot weather.
Lack of social contact is considered an important risk factor for heat-related mortality. It is estimated that people, especially the most vulnerable, spend the majority (80–90%) of their time indoors [185, 186]. Living alone has generally been shown to be one of main risk factors for mortality [121, 123, 125, 187], although some studies observed no greater risk which may be linked to differences in geographical location [188, 189]. Involvement in group activities, maintaining friendships, or even having a pet in the household have been shown to provide some measure of protection [121, 123]. While living alone should not be equated with social isolation, changes in the social and built environments may have led to an increase in the number of people living alone, who are also socially isolated. It is noteworthy that there has been an increase in recent years in the number of individuals, especially seniors, who live alone [190]. There has also been a trend toward the decreasing availability of safe public spaces and supported housing arrangements, thereby decreasing the availability of social contact for many vulnerable individuals (e.g., senior citizens, individuals with mental illness) [125].
The direct relationship between heat-related mortality and race/ethnicity is less obvious, possibly due to the complex interplay of many factors that may influence vulnerability [191]. While some report a higher risk of heat-related morbidity and mortality in individuals of nonwhite origin [141, 168, 169], and in particular African Americans compared with Caucasians [142, 191, 192], others have reported no differences [193, 194].
Heat Health and Geographical Location
Many studies have documented the importance of geographic location in heat-health relationships. Populations adapt to local climate conditions physiologically and through cultural and behavioral practices [195]. As noted earlier, minimum mortality thresholds increase in warmer climates. This is well illustrated by McMichael et al. and colleagues [195] who evaluated 12 cities across the globe, spanning tropical (12°N) to mid-latitude regions (46°N). They observed that minimum mortality thresholds varied from 16 °C to 31 °C, generally increasing in warmer climates. Irrespective of geographical locations, global average surface temperatures are predicted to rise, and heat waves are expected to increase in intensity, frequency, and duration; dense urban environments will be most impacted because of the urban heat island effect [129, 196–198].
Heat islands can form over any built-up area, but especially occur over large cities where surfaces absorb large quantities of solar radiation during the day and radiate it during the night, sustaining higher temperatures than surrounding rural areas and intensifying the effects of heat waves [12, 199]. The magnitude other urban heat island effect varies through the day, by season, and geographically. While green spaces are associated with decreased heat-related morbidity and mortality in urban areas [200], these spaces are increasingly limited in large metropolitan cities. The hottest areas tend to have high density of buildings or roadways, little greenspace, intense generation of anthropogenic heat such as by factories, and low air circulation caused by a very dense cluster of building and narrow streets [201]. While urban density is a major factor contributing to the urban heat island effect, studies indicate that surface temperatures in lower-density land use caused by urban sprawl in large cities [202] are also on the rise. Urban sprawl has been shown to increase the probability and intensity of heat waves [203] increasing the likelihood of high indoor temperatures that can pose a danger to a person’s health and well-being.
Even within the same city, heat exposure may differ among neighborhoods based on land-use patterns and land cover [204]. In this context, individuals are not uniformly exposed to similar levels of heat stress during extreme heat events. A report by Hondula and co-workers showed significant fine-scale spatial variability in heat-related mortality within Philadelphia such that heat-related mortality across different sectors of the city varied by as much as 50% [197]. Several studies have incorporate multiple variables such as those associated with environmental (e.g. land-use type or greenspace), sociodemographic (e.g., education, income, age, ethnicity), living conditions (e.g., access to air conditioning), and health (e.g., diabetes) determinants to develop heat vulnerability indices [205] or heat maps [206] that are useful for identifying geographic patterns of sensitivity to heat. These measures have been shown to be effective indicators of geographic vulnerability to heat-related mortality and morbidity within cities and can assist health officials develop action plans for extreme heat events including resource allocation needed to support increased hospital admissions, ambulance transport needs, public cooling centers, and others [206, 207].
As outlined above, there are a number of factors that can affect temperature and therefore human health during an extreme heat event. However, the challenge remains in quantifying a person’s level of heat exposure which can be highly variable between individuals. This is in part due to the combined influence of differences in activity patterns [208] and the built environment [201, 209]. Even within dwellings, there can be considerable heterogeneity in thermal exposures depending on building characteristics. For example, upper stories, a southern orientation, and an elevated glazing-to-wall ratio are associated with a greater indoor air temperature [210]. Of note, the thermal characteristics and qualities of a home accounts for a greater variation in indoor temperature than the effect of location within the urban environment itself during an extreme heat event [211]. Other factors such as the number of occupants in a home will also have an influence as overcrowding is associated with greater internal heat gains [212]. Because there are differences in how groups of people may respond behaviorally to the same living environment a person’s level of exposure to heat can vary. While the use of protective measures (e.g., use of operable windows to enhance air exchange, mechanical ventilation, use of air conditioning, etc.) can mitigate potentially dangerous increases in thermal strain, the appropriate behavioral strategies to implement these measures must occur (e.g., turn on air conditioner, open window, close window blinds, etc.). In the case of vulnerable population such as the elderly, the sick, and others, in the absence of assistance, the activation of protective measures may not be possible.
Occupational Heat Stress in a Changing Climate
Impact of Climate Change and Heat on Occupational Health
At present, nearly 30% of the world’s population is frequently exposed to climate conditions that exceed human thermoregulatory capacity [50]. Even if aggressive mitigation measures are adopted by countries across the globe, half of the planet’s population will still be exposed to such conditions by 2100 [50], leading to ever-increasing levels of occupational heat stress [30]. Occupational heat stress typically originates in industries from harsh environmental conditions (e.g., increased air temperature and humidity, radiant heat sources, limited air flow), the requirement to wear insulated and/or impermeable protective clothing, and the high metabolic heat load from performing physically demanding tasks, especially when wearing heavy equipment [85, 213]. While the definition/threshold of occupational heat stress can vary based on these factors, in a recent systematic review and meta-analysis of the relevant literature, we found that occupational heat stress is defined in the vast majority of studies as air temperature beyond 27 °C or 33 °C for moderate-/high- or low-intensity work, respectively, or WBGT beyond 22.0 °C or 25 °C for moderate-/high- or low-intensity work, respectively [30]. Working under occupational heat stress conditions leads to progressive rises in core temperature, cardiovascular strain, and fluid depletion [214], which can lead to heat stroke or death [215]. In fact, the core temperature of individuals working under occupational heat stress is increased by 0.7 °C beyond normal, and their urine-specific gravity (a marker of hydration status) is increased by 14.5% compared to the start of the work shift 30. Moreover, 15% of individuals who typically work under occupational heat stress experience kidney disease or acute kidney injury [30]. It is not surprising, therefore, that recent large-scale epidemiological studies on heat-related illness and mortality show that occupational heat stress is responsible for 13–36 deaths per year in the United States alone [216, 217].
Physiological Strain During Work in the Heat
The term occupational heat strain is used to describe the abovementioned physiological and psychophysical effects of occupational heat stress on the worker and his/her performance. In this sense, the occupational heat strain refers to the physiological consequences of occupational heat stress, and a number of studies report that these consequences directly threaten workers’ health, with associated negative impacts on productivity, poverty, and socioeconomic inequality [10, 23, 30]. Indeed, nearly one million “work-life years” (similar notion as DALYs) will be lost by 2030 due to occupational heat stroke fatalities, and 70 million “work-life years” will be lost due to reduced labor productivity [218, 219].
Core body temperature higher than 38 °C, according to international occupational health and safety standards [220–222]
At least one occupational heat strain symptom, as defined by international health and safety guidelines [220, 222–225]
- (a)
- (b)
- (c)
Urine-specific gravity ≥1·020 (indicating dehydration) [224]
- (d)
Heat-associated self-reported nausea or vomiting (indicating heat stroke) [223]
- (e)
Painful muscular spasms (indicating heat cramps) [223]
- (f)
Confusion, dizziness, or fainting (indicating heat syncope, heat exhaustion, or heat stroke) [223]
- (g)
Hot dry skin (indicating heat stroke) [223]
- (h)
Self-reported heat strain (indicating heat exhaustion) [223]
- (a)
Cholesterol concentration higher than 6·7 mmol/L or low-density lipoprotein concentration higher than 3·4 mmol/L (indicating heat-induced dyslipidemia) [228, 229]
Indices of Occupational Heat Stress and Strain
In the past century, more than 160 environmental heat stress indices have been developed [230] and, as outlined earlier, the most widely used are WBGT and UTCI. The vast majority of these indices consider meteorological factors (e.g., temperature, humidity, wind, solar radiation) and elements of the physical environment that can be measured with instruments to estimate the psychophysical impact on the human body (i.e., the heat strain). Despite their wide usage, the efficacy of these indices for the management of heat strain imposed upon individuals during work or leisure activities is limited and has received some criticism [231–236]. This is illustrated by the fact that heat injuries have been documented in a wide range of air temperatures (26–49 °C) and water-vapor pressures (0.8–3.5 kPa, corresponding to 10–100% relative humidity) [52, 237, 238].
- (i)
Age ≥53.0/55.8 years
- (ii)
Body mass index ≥29.5/25.7 kg·m−2
- (iii)
Body fat ≥28.8/34.9%
- (iv)
Body surface area ≤2.0/1.7 m2
- (v)
Peak oxygen uptake ≤48.3/41.4 mlO2·fat free kg−1·min−1
Worker Productivity Under Occupational Heat Strain
In parallel to physiological changes, occupational heat stress generates discomfort and fatigue [214], which can lead to labor losses by compromising worker productivity [23, 240]. Indeed, recent meta-analytic data shows that 30% of individuals who work under heat stress report productivity losses [30]. The same systematic review and meta-analysis reports an average 2.6% productivity decline for every degree increase beyond 24 °C WBGT [30]. In occupational settings, problems are already felt by millions of workers [30], especially those above 53/56 years old (males/females) who are especially susceptible to heat illness [140]. The available data suggest that in future decades, individuals working even in temperate climates may be at risk for heat illness on nearly 40 days/year [241]. In line with this, the extent of the damaging potential of heat on human productivity is evident in estimations for 2030, according to which the heat-related labor productivity losses may be 70 times more damaging to healthy, productive, and disability-free life years, than those resulting by occupational heat stroke fatalities [219].
Overview of Occupational Heat-Related Illnesses
Occupational heat-related illnesses – a fully preventable range of conditions – generate deleterious health and productivity outcomes, and it is vital that they are recognized globally as a public health problem [30, 242]. It is necessary to international attention and take action on this problem, particularly in light of climate change and the anticipated rise in environmental heat stress. Developing a surveillance system to monitor prevalence of occupational heat strain throughout the world could be a first step. In parallel, workers and employers should be educated about the health and performance effects of occupational heat strain, while effective screening and mitigation protocols should be incorporated within health and safety legislation.
Heat Health Planning and Protection for General Population
The Current State of Planning and Prevention
A direct relationship between acute mortality and exposure to high temperature has been recorded in almost all populations where it has been studied [243]. As discussed earlier, a well-known analysis of published temperature-mortality relationships in populations living in temperate and cold climates has shown that this association is U- or V-shaped, with exponential mortality risk increase at both high and low temperatures [244]. These results suggest that populations are adapted to their local climates [245] and that mortality risk is increased beyond a location-specific optimum temperature range. Work by the World Health Organization [246] has shown that the factors (economic development, infrastructure, health systems, etc.) that affect the ability of developed countries to prepare for and respond to climate-related hazards such as heat waves vary widely. Additionally, the resilience and adaptive capacity of populations and health systems is different among and within countries. More importantly, the same work [246] highlighted that the interest or capacity of government agencies to collect and circulate information on heat wave response should not be assumed, despite the widely publicized and unanimously agreed health effects of climate-related hazards on health and productivity. The willingness and adaptive capacity reflect each country’s specific conditions and priorities, while factors such as location, socioeconomic development, and recorded mortality do not appear to play important roles. Instead, the single most important factor that appears to determine action or inaction across developed countries pertains to the existence (or absence) of a culture for protecting health from climate change [246].
Community Adaptation Programming and Health Advisory Systems for Heat Events

Overview of the geographical representation of the 21 countries where early warning systems for heat waves have been tested according to the World Meteorological Association 224. Color codes representing the different World Health Organization regions: Americas (red), Europe (blue), and Western Pacific (green) (no studies have reported on early warning systems tested in Africa, Eastern Mediterranean, or Southeast Asia)
In light of the above discussion on occupational heat stress and strain, it is crucial to note that the existing early warning systems are designed for the general population whose needs and exposure to heat are vastly different from those of workers. For instance, the typical adaptation strategy in existing systems is to advise individuals to stay indoors throughout the day or to remain in “cooling shelters” at public buildings. However, such strategies are not an option for industrial actors given their need to maintain productivity regardless of the prevailing environmental conditions. Moreover, existing systems have a forecasting capacity limited to a few days which is insufficient for industrial actors who need to plan their activities weeks (or months) in advance to ensure a high level of productivity. Therefore, a different approach is needed to address the health needs of the European workforce.
Heat Protection and Management Strategies for Workers
As noted in the subsection above (Occupational Heat Stress in a Changing Climate), heat stress is a deadly, yet common, occupational hazard, reported to be responsible for 37 fatal and 2830 nonfatal occupational injuries and illnesses in the United States in 2015 [248]. Given the increasing frequency and intensity of heat waves due to global warming, heat-related deaths in workers have been estimated to increase by as much as 37% for each ~0.55 °C (1 °F) rise in average daily summer temperature [249], with workers facing a four- to sevenfold greater risk of experiencing a heat-related illness during a heat wave [250]. Excessive heat strain can also cause psychophysical strain (e.g., discomfort, fatigue), which can exacerbate the risk of injury and cause reductions in worker productivity [23, 251–253], costing up to US$6.2 billion in heat-related labor losses [254]. However, while heat stress management guidelines have been recommended by the National Institute for Occupational Safety and Health (NIOSH) [255] and other safety institutions for some time, heat stress continues to compromise worker health and productivity. The following subsections describe the current approach to occupational heat stress management and future directions to better protect and maximize the productivity of individuals working in our warming global climate.
Occupational Heat Stress Management: Common Practices and Methodologies
To reduce the risks associated with heat stress, NIOSH recommends upper heat stress limits defined by the environment (assessed with WBGT) and metabolic rate, expressed as a time-weighted average [255]. Two sliding-scale limits are then specified, the Recommended Alert Limits (RALs) and the Recommended Exposure Limits (RELs) , which are analogous to the American Conference of Governmental Industrial Hygienists (ACGIH) Action Limit Values and Threshold Limit Values (TLV®) [256], respectively. The RALs are directed at protecting unacclimatized workers (i.e., individuals who do not possess thermoregulatory adaptations associated with repeated heat exposure), while the less conservative RELs are directed at protecting acclimatized workers. Both upper limits are designed so that healthy workers, who are adequately hydrated, unmedicated, and wearing only a single clothing layer (~1 Clo) should be able to tolerate an 8-hour exposure to such heat stress without incurring adverse health effects for a 5-day workweek. Since additional insulated or impermeable clothing can restrict heat exchange [257], the WBGT associated with the RAL and REL is lowered to consider these effects (e.g., two-layered clothing requires that the WBGT for each limit is lowered by 2 °C). In instances where these thresholds are exceeded, engineering (e.g., ventilation, shade) and administrative controls (e.g., work-to-rest allocations), hygiene practices (e.g., personal cooling devices, fluid consumption schedule), and physiological monitoring are prescribed.
While current occupational heat exposure limits such as the RALs and RELs may provide a relatively simple means to manage heat stress, recent laboratory-based evaluations of the ACGIH TLV® (analogous to the RELs) indicate that unsafe rises in body core temperature (≥38.0 °C) would occur during work shifts ≥4 h in young [234] and especially in older workers [236] when work is performed within these limits. Several instances of peak core temperatures exceeding the ACGIH guidelines for acclimated workers (≥38.5 °C) as well as two instances of more severe heat strain (≥39.5 °C) have also been observed during field-based evaluations of heat strain in electric utilities workers [83]. It has therefore been suggested that current guidelines may provide only moderate protection to worker health [258]. This is because the heat strain one may experience in response to a given level of heat stress can differ markedly among individuals due to various interindividual factors and intraindividual factors both within and beyond a worker’s control (see section “Inter- and Intraindividual Determinants of Heat Strain” for additional details). As such, the assessment of heat stress alone can result in the over- or under-protection of workers from heat-related illness, with the former leading to a reduction in productivity in more heat-tolerant workers (false positive) and the latter compromising safety in less heat-tolerant workers who may develop heat-related illness even in mild conditions (false negative).
To provide more individualized protection, it has been suggested that future emphasis be directed toward the development of guidelines that consider interindividual factors that commonly differ among workers (e.g., sex, age, chronic disease), as well as critical intraindividual factors that can be identified indirectly with basic worker information (e.g., consecutive work days, shift duration) or pre-work assessments (e.g., urine-specific gravity to assess hydration state) [99]. This may involve making adjustments to existing RELs to consider how such factors may impair or improve heat dissipation and the resulting increase in heat strain in response to a given heat stress. These individualized guidelines would therefore serve as a risk stratification tool to identify individuals who may be more susceptible to heat-related illness, even in mild heat stress conditions, while identifying those individuals who are better equipped to continue work in higher heat stress conditions. In instances where these individualized guidelines are exceeded, targeted protective strategies could be employed to alleviate heat strain.
Although individualized heat stress guidelines may better protect workers health than a generalized exposure limit, it is challenging and perhaps impossible to comprehensively consider all individual factors and their interactive effects on heat strain. For this reason, a more comprehensive solution to managing occupational heat stress may involve monitoring one or more physiological indices of heat strain (e.g., noninvasive or indirect measures of body core temperature and cardiovascular strain, among others), as opposed to the level of heat stress [255, 258]. This approach therefore prevents the over- or under-protection of workers from heat-related illness (i.e., false positives and false negatives) by ensuring that productivity is maximized in more heat-tolerant employees when working in high heat stress conditions and that heat-related illness is prevented in less heat-tolerant workers when working in temperate conditions. These physiological data can also be used to provide alert thresholds to management to ensure that safety limits are not exceeded and to provide physiological feedback to workers to ensure that they control their work rate to optimize productivity while preventing excessive heat strain [259]. Given the added protection offered by physiological monitoring and the recent increases in the development of wearable monitors providing a noninvasive and portable means of quantifying physiological strain, heat strain monitoring may represent the future of occupational heat stress management, especially given our diverse working population.
An occupational heat stress management program that incorporates physiological monitoring may provide a more comprehensive solution to protect worker health, although the implementation of such technology is not without its challenges [258]. Indeed, while some monitoring systems are certified for use in hazardous areas and possess water- and dust-resistance, future research is needed to evaluate the utility of these systems in harsh industrial environments for wearable monitoring to be successful. Further, materials that are nonflammable or flame resistant, noncombustible, hypoallergenic, as well as resistant to arc flash and electromagnetic fields must be used in the construction of a wearable heat strain monitor to satisfy existing safety requirements in many industries. While some of the systems available satisfy such requirements, others would require modification prior to use. Moreover, despite their benefits, the costs associated with purchasing wearable monitors for all employees may be prohibitive for some companies. These costs, however, could be reduced by restricting monitoring to “high-risk” work and/or weather conditions and/or by monitoring employees who, due to their age, health status, or physically demanding occupation, may be more vulnerable to heat-related illness, a process that could be aided by the individualized guidelines noted above [99]. Finally, it is possible that physiological monitoring per se may modify worker behavior (reactivity). This may be characterized by an increase in work intensity and a reluctance to cease work even when experiencing excessive heat strain or a reduction in work effort to avoid displaying greater fatigue than their co-workers. Unfortunately, the former may exacerbate heat strain, while the latter may reduce productivity. Although such behavior modifications may be minimized if physiological monitoring was routine or if management intervened, future research is needed to identify if and to what extent physiological monitoring may modify worker behavior.
Summary
Climate change projections show that there will be continuing increases in the occurrence and severity of some extreme heat events over the next decades placing. As a consequence, there is immediate need to use best scientific practices and other evidence-based methods to protect the public and workers against the potentially harmful effects of exposure to extreme heat. This includes formulating culturally sensitive solutions, policies, and guidelines to protect health, safety, and performance as well as prevent disease exacerbated by heat across different spheres – healthcare, civil protective agencies, military, industry, sports, and others – against the harmful effects of heat.

Full access? Get Clinical Tree
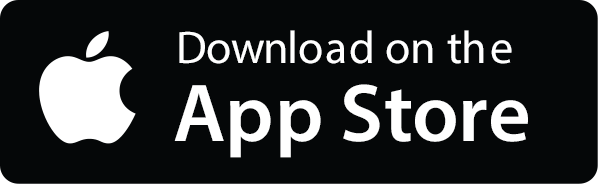
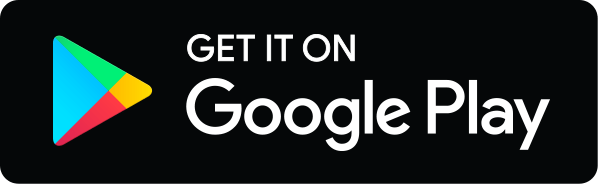