Exercise Physiology and Exercise Testing
Carl Foster
Matthew J. Faber
John P. Porcari
Basics of Exercise Physiology
Exercise is about skeletal muscle contraction. Exercise physiology is about how skeletal muscles contract, how the rest of the body arranges to deal with the disturbance in homeostasis provoked by skeletal muscle contraction, how these physiological mechanisms adapt to repetitive skeletal muscle contraction (exercise training), and (for the clinician) how these physiological mechanisms are deranged in pathological states. However, without the obligatory requirement for skeletal muscle, there is no exercise.
At the simplest level skeletal muscle contraction involves a linkage of the actin and myosin filaments that are part of the architecture of the basic unit of every muscle, the sarcomere. This is mediated by calcium released from the sarcoplasmic reticulum in response to the depolarization induced when acetylcholine is released at the motor end plate. This process leads to a cascade of reactions that results in the formation of an actin-adenosine triphosphate (ATP)-myosin complex, leading to a conformational change in the myosin unit, which in turn causes traction to be exerted on the actin filament. Because the architecture of the muscle is symmetrical, the process leads to the actin filament pulling on the rudiments of the connective tissue architecture of the sarcomere, resulting in shortening the muscle. Ultimately, this leads to the muscle pulling on a ligamentous or bony structure, resulting in movement. Thanks to the exquisite coordination of the motor nerve center, the timing and sequence in which muscle fibers are recruited allow for purposeful and effective motion. This is most elegantly evident to the sports physician who, typically, has the duty of providing care for individuals with very well developed neuromuscular systems, and the physiological support structure to allow muscle contraction to continue, often at a very high rate compared to nonathletic individuals.
The ability to provide for continuing muscular contraction ultimately depends on the availability of ATP within each sarcomere. This is provided for by three interlinked and overlapping energy systems that not only provide very rapid resynthesis of ATP for short bursts of activity but can also provide for low to moderate intensity activity to be continued almost indefinitely.
Energy Systems
There is a small storage reservoir of both ATP and a related compound, creatine phosphate (CP), in the cytoplasm of each sarcomere. These substances, collectively referred to as phosphagens, can provide for very rapid resynthesis of ATP on the myosin head, allowing for brief but very high-intensity bursts of muscular activity. However, the total quantity of phosphagens is very low. If other sources of ATP were not available, the duration of phosphagen-powered exercise would be only in the order of seconds. The second energy system is related to the anaerobic degradation of muscle glycogen to pyruvate. This not only yields pyruvate that can be oxidized (in the third [aerobic] energy system) but also directly provides ATP that becomes part of the cytoplasmic phosphagen pool. Because pyruvate can be produced more rapidly than it can be oxidized, and because pyruvate accumulation would stop the ATP production coming from this second energy system, pyruvate may be reversibly converted to lactate, which may then be transported out of the cell and used by other tissues. This provides not only for continued glycogen to pyruvate hydrolysis but (through the proton release correlated with lactate transport) also provides a negative feedback loop to limit the duration of
high-intensity exercise (e.g., the metabolic acidosis induced by lactate contributes to both increases in ventilation and to perceptual responses such as local muscle discomfort that ultimately cause the individual to reduce the intensity of exercise). The third energy system depends on the oxidation in the mitochondria of either pyruvate, acetyl CoA formed directly from glucose, or free fatty acids. This is a low power but very high capacity energy system that is dependent on the availability of molecular O2 within the cell.
high-intensity exercise (e.g., the metabolic acidosis induced by lactate contributes to both increases in ventilation and to perceptual responses such as local muscle discomfort that ultimately cause the individual to reduce the intensity of exercise). The third energy system depends on the oxidation in the mitochondria of either pyruvate, acetyl CoA formed directly from glucose, or free fatty acids. This is a low power but very high capacity energy system that is dependent on the availability of molecular O2 within the cell.
The Oxygen Connection
Although the phosphagen and the glycogen to pyruvate energy systems are quite important (particularly at the beginning of exercise or during very high-intensity exercise), the oxidative energy system is quantitatively the most important. And, because it depends on the availability of O2, which is plentiful in the room air but very scarce at the level of the sarcomere, much of the physiology of the body is organized to transport O2 to the tissues. This requires the combined actions of the cardiorespiratory system to extract O2 from the inhaled air and transport O2 in the arteries, to deliver CO2 (a prime waste product of oxidative metabolism) back to the lung, and then to selectively deliver O2 to the active muscles, with active reductions of blood flow to less active tissues. The characteristic changes in heart rate, stroke volume, end diastolic volume, and ejection fraction that are hallmarks of the cardiovascular response to exercise are all designed to facilitate increasing the cardiac output to a level consistent with providing O2 to the tissues. The cardiovascular response to exercise is well integrated by the Fick equation, which defines this response. Likewise, the characteristic changes in pulmonary tidal volume and respiratory frequency during exercise are designed to facilitate adequate alveolar O2 and CO2 concentrations so that delivery of blood to the lungs results in appropriate gas exchange. Lastly, coordinated vasodilation in the active tissues, often regulated by the metabolites of muscular contraction, is designed to allow selective delivery of blood to the active tissues. This allows for optimizing O2 delivery to the tissues with the minimal increase in cardiac output.
Adaptations to Chronic Exercise
The other aspect of exercise physiology that is immediately obvious, and of particular importance for the sports physician is the adaptability of both the musculoskeletal system and the cardiorespiratory support system to regularly performed exercise. This is elegantly obvious in the legend of Milo of Crotona. Milo was a young farm boy who lifted a newly foaled bullock on his shoulders and walked around the barnyard. On successive days, Milo continued lifting the growing bullock, until he was capable of lifting a fully grown bull, becoming the strongest man in the world (and an Olympic champion in the ancient Games) in the process. This feat was actually reproduced in the early days of television for a show called “You Asked For It.” Apparently, a farm boy lifted a growing cow for some months. The cow gained more than 200 lb although the boy gained only 3 lb. The adaptability of the exercise capacity is also elegantly demonstrated by the large number of normal individuals (and even cardiac patients) who can adapt well enough to exercise training to complete marathon length races without particular problems.
The adaptation to repetitive exercise can be understood in terms of several factors that can be recalled using the anagram P-ROIDS (a humorous word play on the fact that urine is the most common testing medium for anabolic steroid abuse). These principles are:
Progression
Reversibility
Overload
Individual differences
Specificity
Exercise Stress Testing
Physicians are often called on to perform evaluative procedures to determine the presence of pathological states that might influence participation in exercise and/or to provide guidance regarding increased levels of activity. At the outset it is critical to remember that exercise testing is simply an extension of the history and physical examination. Just as the physician might manipulate an injured limb to determine what motions lead to discomfort, and thereby evaluate the nature of the presenting complaint, a patient’s responses during incremental exercise may allow the physician to observe the patient when he/she is symptomatic. In a general sense, there are several broad indications for exercise stress testing including the following:
Evaluation of exertional discomfort. Including provocation of exertional angina pectoris, dyspnea or exertional bronchospasm, claudication, palpitations, or hemodynamic abnormalities.
Provocation of occult pathology. Particularly, occult cardiovascular disease in which the first presentation is often fatal, particularly in males. Provocation of pathophysiology before the presentation of sudden death or acute myocardial infarction provides the physician with many more options for treating the patient.
Defining prognosis in known pathological states. This is particularly critical when deciding amongst several therapeutic options. Effectively, this can guide the physician regarding which patients might benefit from more aggressive or invasive therapy, and which may effectively be treated with less invasive therapies.
Evaluation of therapy. Improved exercise tolerance and disability evaluation.
Exercise prescription. Guidance regarding the appropriate Frequency, Intensity, and the Time and Mode (FIT’M) of exercise to achieve therapeutic goals. Accepted guidelines recommend at least 30 minutes of moderate-intensity exercise, performed in one or more sessions on most days of the week. However, for improving physical fitness and performance capacity, a likely goal amongst the patients of sports physicians, more strenuous training (particularly higher-intensity training) is usually critical to success.
Graded Cardiac Stress Testing
The most familiar form of exercise testing for most physicians is the cardiac stress test. This procedure is typically conducted either to evaluate exertional discomfort that might be consistent with cardiovascular disease or to reveal the presence of occult cardiovascular disease. Most typically, exercise is performed on either a treadmill or cycle ergometer, although other forms of exercise can be used effectively. There are a variety of exercise protocols. The most commonly used is the Bruce treadmill protocol (3) (see Table 3.1). Although this protocol starts at a fairly high energy requirement (approximately 5 times the average resting metabolic rate or 5 metabolic equivalents [METs]) and has relatively large increments between stages, it is still a well recognized and convenient way to conduct this aspect of the physical examination. We have created a convenient modification of the Bruce protocol, that allows for a lower-intensity start and smoother transition between stages (see Table 3.2). Although lagging slightly behind the Bruce protocol, on a METs/minute basis, the modified protocol is much better tolerated than the standard Bruce protocol.
During the exercise test, the exercise capacity is typically estimated from established prediction equations (4
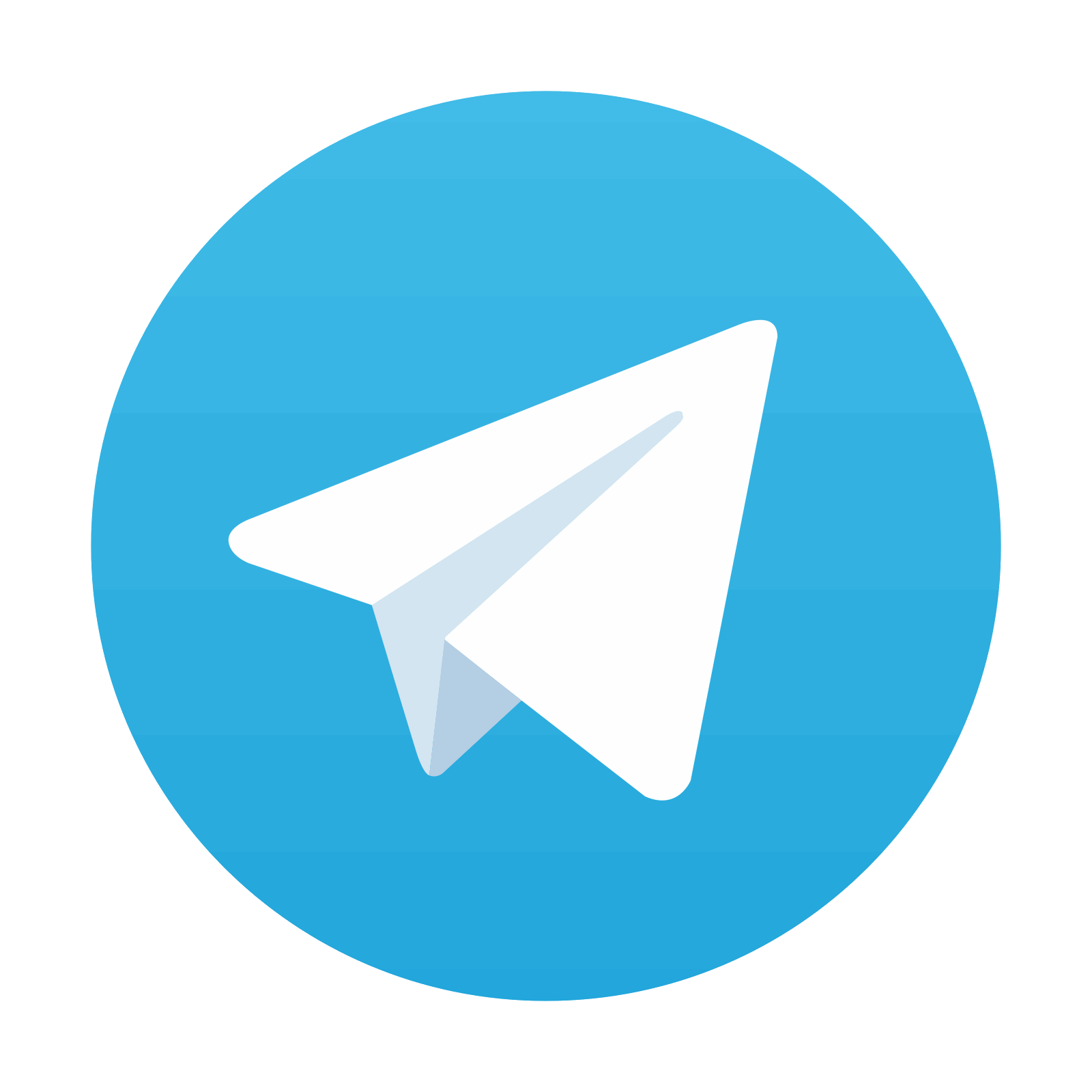
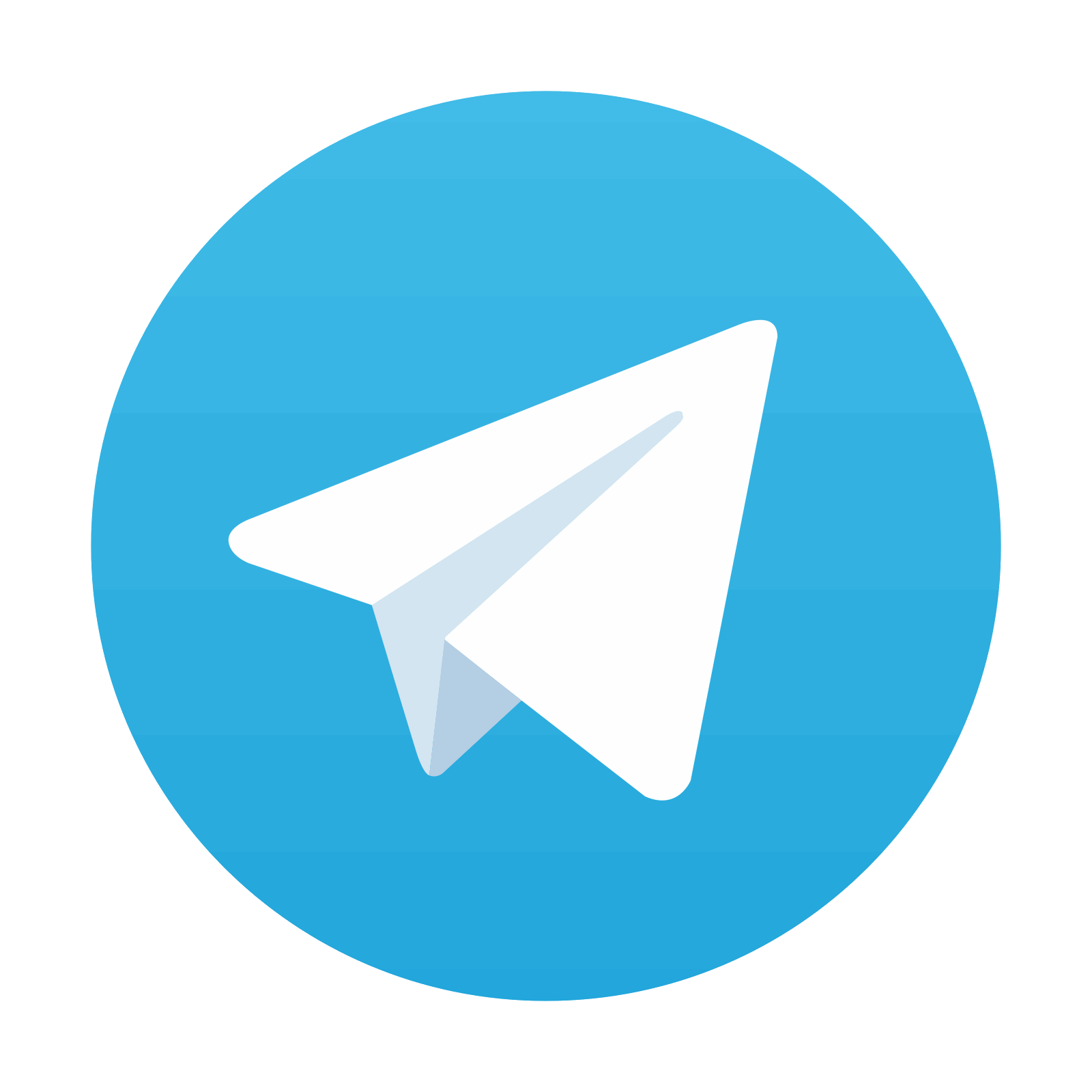
Stay updated, free articles. Join our Telegram channel

Full access? Get Clinical Tree
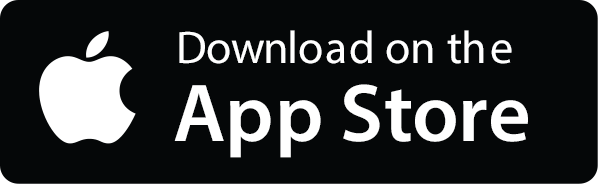
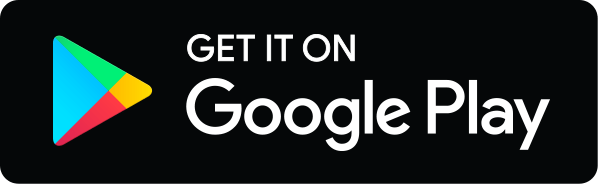
