© Springer International Publishing Switzerland 2017
Francesco Feletti (ed.)Extreme Sports Medicine10.1007/978-3-319-28265-7_33. Endocrine Responses to Extreme Sports
(1)
Division of Sports Medicine, University School of Physical Education, ul. Paderewskiego 35, Wroclaw, 51-612, Poland
(2)
Department of Endocrinology, Diabetology and Isotope Treatment, Wroclaw Medical University, Wroclaw, Poland
Keywords
Physical activityIronmenExerciseCatecholaminesCortisolAndrogensEstradiolThyroxineGrowth hormoneTestosteronePsychological stressCoping response3.1 Effects of Regular Exercise on Endocrine Functions
Directly or indirectly, physical activity influences functions of all endocrine glands. However, effects of exercise vary greatly depending on, e.g., type, duration, and intensity of exercise, genetic variants, race, gender, age, body composition, nutritional status, diet, day/season variations, training history, or performance level of an athlete.
Short-time exercise of any kind is associated with significant changes in the hypothalamo-pituitary-adrenal (HPA) axis. Catecholamines (secreted by the adrenal medulla) and cortisol play the key roles here. Concentrations of catecholamines (adrenalin and noradrenalin) may rise severalfold immediately after the beginning of an exercise. They return to basal levels (or lower) 5–10 min after the end of an exercise. Changes of catecholamine levels differ depending on the intensity and the duration of exercise. It is suggested that the reactivity of the adrenal medulla is diminished with aging [1–3].
The secretion of cortisol from the adrenal cortex is stimulated by the pituitary hormone – adrenocorticotropin (ACTH). There is no consensus on the intensity of exercise that is able to activate ACTH secretion. The threshold may be between 30 and 80 % VO2max. Cortisol concentration rises due to endurance rather than power training. Long-distance running or canoeing have higher potency to induce increases in salivary/plasma cortisol than short-time or interval exercise. Similarly, anaerobic exercise changes cortisol stronger than aerobic exercise. The response of the HPA axis to exercise is less pronounced in well-trained individuals [4–6].
Regular training does not influence basal concentrations of ACTH or cortisol. However some data from epidemiological studies indicate that physical activity may be associated with hair cortisol concentration [7].
Generally, overtraining is characterized by reduced ACTH and cortisol responses to stimulation. In overreaching and overtraining syndromes, specific patterns of ACTH and cortisol reactions are observed [8, 9].
Among many beneficial effects of regular exercise, one must underline its effects on glucose metabolism. 10–15 min of exercise exceeding 40 % VO2max is able to reduce insulin secretion by 40 %. Physical training enhances insulin sensitivity by increasing expression of insulin receptors in muscles and stimulating glucose utilization. Longer exercise (e.g., 120 min at the intensity of 30–50 % VO2max) is associated with increased secretion of glucagon and increased hepatic gluconeogenesis. Although the influence of exercise on glucose transport to muscle cells lasts up to 120–240 min, insulin sensitivity remains increased for about 48-h postexercise. Expression and activity of GLUT4 (glucose transporter 4) in skeletal muscle is enhanced by endurance training (especially regular) [10–12].
Physical exercise is the strongest physiological stimulus for secretion of growth hormone (GH). Exercise that exceeds 30 % VO2max may lead to tenfold increases in plasma GH concentration. The rise of GH is usually observed within 10–15 min from the start, and it may reach its peak 25–30 min from the beginning of an exercise. GH concentration may stay elevated for more than an hour afterward. IGF-1 follows a similar pattern showing a rise after 10 min of exercise and reaching its peak before 20–40 min from the start of a workout. The greatest response is noted for intensities above the lactate threshold. Exercise of moderate intensity, short intervals between consecutive bouts, and activation of large muscles are associated with stronger response of GH/IGF-1 axis. On the other hand, low temperature, high-fat meal, or obesity may attenuate GH response to exercise.
The reactivity of the GH/IGF-1 axis differs among races; gender differences are of minor importance here. Younger age is associated with greater GH response to exercise. Long-term training has no impact on basal concentrations of GH in both younger and older individuals. Some authors suggested that lower level of fitness correlated with less pronounced GH response; however, it was undermined by others [13–18].
Short-term training (days, weeks) of athletes and non-athletes, men and women, resulted in decreased concentrations of IGF-1. Some authors suggested that lower levels of IGF-1 may indicate overtraining. Training of a longer duration (months) either rises or does not change basal concentrations of IGF-1. However there were published results suggesting the presence of a positive correlation between VO2max and plasma IGF-1 [13, 18, 19].
Only exercise of intensity over 50 % VO2max induces noticeable fluctuations in the components of the pituitary-thyroid axis. The impact of a short-time exercise on thyroid hormones is usually modest and short lived. Reports on changes in concentrations of the thyroxin (T4), triiodothyronine (T3), and free fractions of T4 and T3 (FT4, FT3) brought ambiguous results [20–22].
Many authors observed reduced levels of gonadotropins after intense exercise; however, there were also reports in which gonadotropins did not change or increased. Similarly, some investigators observed 10–25 % rise in testosterone concentration after exercise, but others noted decreased testosterone concentration that remained lower for 13 h after, e.g., resistance exercise. Long-term influence of physical training on androgen concentration is insignificant [13, 23–25].
3.2 Endocrine Responses in Ultra-endurance Sports
The studies on the reactivity of the HPA axis to endurance exercise/training are unequivocal. It seems that HPA axis in Afro-Americans is more sensitive to training than in people of European origin.
For example, intensive, 4-day march (164 km) did not affect basal or postexercise concentration of ACTH in males. However in ultramarathon runners, supraphysiological concentrations of ACTH were observed. Results of some other studies also suggested that this type of training may increase the level of ACTH. In men, repeated 50-km skiing during 2 days (Finlandia Ski Race) increased cortisol concentration 2.2- and 2.6-fold after the races. Covering a distance of 42 km in kayak changed cortisol concentration more than if the distance was 19 km [26–29].
Triathlon can be treated as a flagship of ultra-endurance sports. It combines swimming, cycling, and running in one event. Among five types of triathlon, the Iron distance is the most demanding one. Participants have to complete 3.9-km swim, 180.2-km bike ride, and 42.2-km run. American authors reported significant changes in steroid hormone concentrations in 57 athletes (38 men), taking part in the Hawaii Ironman Triathlon. They have found that this type of exercise induces a clear increase of estradiol (58 %) and a similar decrease of testosterone in men (58 %). In women insignificant increases of estradiol and testosterone were noted [30]. Observations similar to the above were also made in marathon and ultramarathon male runners. On the other hand, there were no significant changes of the hypothalamo-pituitary-gonadal axis in men marching for 4 days (185 km) with the load of 10 kg [27].
According to some authors, marathon runners may have decreased FT3 and increased rT3 (reverse T3) with no change in T3 levels. According to others, marathon running is not associated with any significant changes in the pituitary-thyroid axis [31].
In men who run on skies for 2 days (100 km), LH and testosterone decreased, and there was no change of FSH. Similar observation was made in participants of a several week-long cycling race or soldiers taking part in military training camps. The comparison of the effects of endurance training in professional cyclists, elite triathlonists, recreational marathon runners, and controls leading a sedentary lifestyle showed only modest differences among studied groups. The only significant one was higher concentration of testosterone in cyclists in the preseason period [29]. Japanese authors studied semen and endocrine parameters in members of the Masherbrum expedition in 1999. Three subjects stayed above 5100 m for more than 3 weeks and above 6700 m for 4–5 days. Specimens were collected before the departure and 1, 3, and 24 months after the return from the expedition. There were no changes of semen volume during the study period. Within the first 3 months, a decreased sperm count and decreased testosterone concentration were noted. There were no persistent alterations of the studied parameters after 2 years from the expedition [32].
Ultra-endurance exercise may turn especially dangerous for women. A characteristic threat is the female athlete triad. The syndrome means the presence of disordered eating, menstrual disturbances/infertility, and osteopenia/osteoporosis. It is estimated that 15–62 % of young sportswomen suffer from some kind of eating disorders. The diagnosis is frequently made in disciplines such as gymnastics, ice-skating or running (anorexia athletica is the extreme form of the problem). The percentage of the body fat mass in female athletes is usually 50 % of that found in non-active women. If the fat mass drops below 22 %, it may lead to menstrual irregularities or even amenorrhea. The risk of menstrual disturbances is ten times higher in sportswomen than in general population. They are found in up to 48–66 % of sportswomen. The female triad syndrome may have year-long health consequences. Regular, intense exercise changes pulsatile secretion of GnRH and reduces frequency and amplitude of LH bursts. It is often associated with lower estradiol, increased sex hormone-binding globulin (SHBG) and luteal phase defects. Not fully explained are disturbances of melatonin, thyreotropin, and prolactin. Amenorrhea in young girls has detrimental effects on bone structure and function in adulthood. Decreased concentration of estradiol reduces the peak bone mass which is achieved before the age of 30. BMD of female athletes with amenorrhea is 22–29 % lower than in age-matched regularly menstruating controls. The risk of bone fractures remains elevated in the fourth and fifth decades of life [33, 34].
3.3 Endocrine Responses to Extreme Psychological Stress
Threat and perceived loss of control are capable to elicit psychological stress. Elite sport is associated with psychological stress, though one cannot exclude such a burden in recreational competition either.
Stress activates two main body systems. The immediate response is conveyed by the sympathetic adrenal system. It involves release of adrenalin and noradrenalin. The visible signs of sympathetic stimulation are, e.g, increased heart rate, constriction of peripheral blood vessels, increased blood pressure, sweating, trembling, and dilation of pupils.
The hypothalamo-pituitary-adrenal (HPA) axis plays the key role in the slower hormonal response to stress stimuli. It starts with the secretion of corticotropin-releasing hormone (CRH) from the periventricular nuclei of the hypothalamus. CRH in turn increases secretion of adrenocorticotropin (ACTH). The release of ACTH from the pituitary stimulates secretion of the hormones of the adrenal cortex and medulla. Another hormone that modulates stress reactions is vasopressin (antidiuretic hormone, ADH). It is synthesized in the hypothalamus, and then it is stored in the posterior part of the pituitary. ADH together with CRH increases secretion of ACTH.
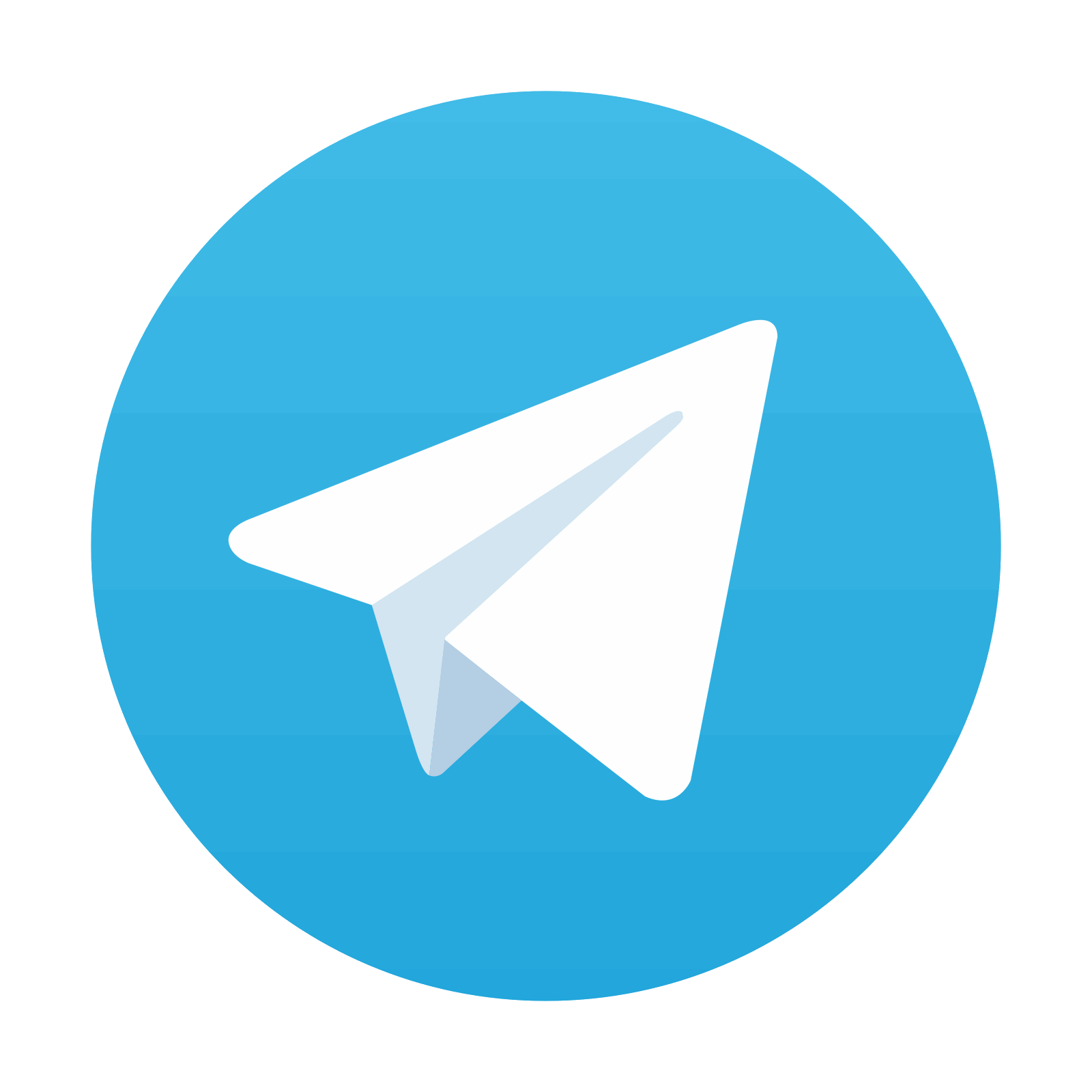
Stay updated, free articles. Join our Telegram channel

Full access? Get Clinical Tree
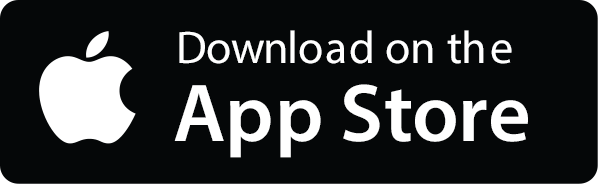
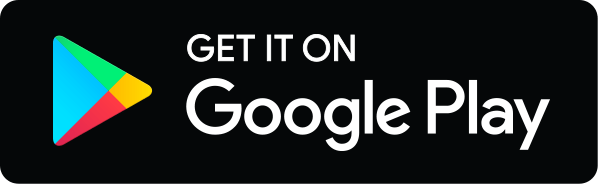