This article reviews the use of electrodiagnostic testing in disorders of neuromuscular transmission and discusses the differences between various presynaptic and postsynaptic disorders. Attention is paid to quality control issues that influence the sensitivity of repetitive nerve stimulation and single fiber electromyography. Electrodiagnostic testing, when used as an extension of the clinician’s history and physical examination, will provide appropriate direction in establishing the diagnosis.
Key points
- •
Conventional needle electrode electromyographic (EMG) examinations are necessary in patients suspected of having myasthenia gravis (MG) to exclude diseases that may mimic or coexist with MG such as peripheral neuropathy and inflammatory or ocular myopathies.
- •
The lack of attention to temperature requirements in repetitive nerve stimulation testing is the most common error made, rendering the study unhelpful. This is most important when considering presynaptic disorders of neuromuscular transmission.
- •
There is no one muscle that will be more abnormal in every patient with MG in single-fiber EMG. The muscle(s) to be tested must be selected based on the distribution of weakness in the individual patient.
- •
Single-fiber EMG provides the most useful information particularly when repetitive nerve stimulation studies are normal and is most always abnormal when there is careful selection of involved muscles including those of the paraspinal region.
- •
Normal jitter in a clinically weak muscle indicates the weakness is not caused by an abnormality of neuromuscular transmission.
Introduction
The roles of electrodiagnosis in disorders of neuromuscular transmission are to confirm or reject one’s clinical impression regarding the presence or absence of a disorder of neuromuscular transmission (NMT), to determine whether the disorder is either presynaptic or postsynaptic, to exclude other coexisting neuromuscular disorders, and to monitor the disease course in response to its natural history or to treatment. Careful clinical and electrodiagnostic evaluation and sometimes genetic, morphologic, and in vitro microphysiologic studies are necessary to achieve an accurate diagnosis. The electrodiagnostic approach is similar to that of any neuromuscular disorder.
The basis of the clinical electrodiagnostic abnormalities in patients with disorders of NMT (eg, myasthenia gravis [MG], Lambert-Eaton syndrome [LES]) is the failure of the muscle fiber to depolarize sufficiently for the end-plate potential (EPP) to reach action potential (AP) threshold ( Fig. 1 ). The resulting impulse blocking accounts for the decremental responses seen on repetitive nerve stimulation (RNS) studies and the impulse blocking seen with single-fiber electromyography (SFEMG). In addition, the time variability of when the EPP reaches AP threshold accounts for the neuromuscular jitter seen in the latter technique. This article will review those electrodiagnostic techniques that are commonly used today and will highlight their specificity, sensitivity, and pitfalls.
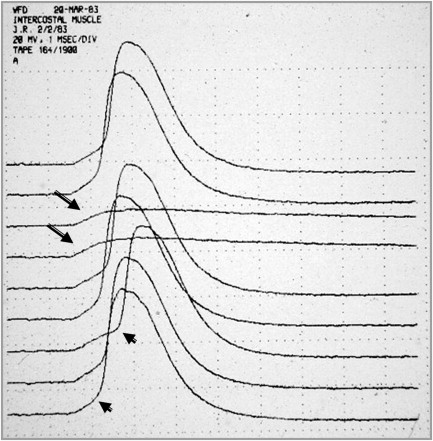
Introduction
The roles of electrodiagnosis in disorders of neuromuscular transmission are to confirm or reject one’s clinical impression regarding the presence or absence of a disorder of neuromuscular transmission (NMT), to determine whether the disorder is either presynaptic or postsynaptic, to exclude other coexisting neuromuscular disorders, and to monitor the disease course in response to its natural history or to treatment. Careful clinical and electrodiagnostic evaluation and sometimes genetic, morphologic, and in vitro microphysiologic studies are necessary to achieve an accurate diagnosis. The electrodiagnostic approach is similar to that of any neuromuscular disorder.
The basis of the clinical electrodiagnostic abnormalities in patients with disorders of NMT (eg, myasthenia gravis [MG], Lambert-Eaton syndrome [LES]) is the failure of the muscle fiber to depolarize sufficiently for the end-plate potential (EPP) to reach action potential (AP) threshold ( Fig. 1 ). The resulting impulse blocking accounts for the decremental responses seen on repetitive nerve stimulation (RNS) studies and the impulse blocking seen with single-fiber electromyography (SFEMG). In addition, the time variability of when the EPP reaches AP threshold accounts for the neuromuscular jitter seen in the latter technique. This article will review those electrodiagnostic techniques that are commonly used today and will highlight their specificity, sensitivity, and pitfalls.
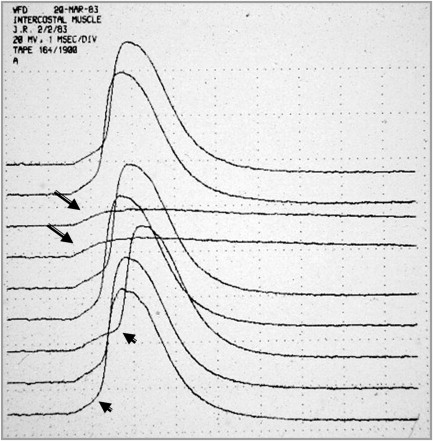
Nerve conduction studies
Standard neurographic studies should be performed on all patients suspected of having disease of the neuromuscular junction (NMJ). Conduction velocities, distal latencies, and late responses are typically normal in these patients. Compound muscle AP (CMAP) amplitude or negative peak area to a single stimulus will be preserved in postsynaptic disorders (unless the disease is very severe) and typically will be reduced in presynaptic disorders (eg, LES and botulism).
In some congenital myasthenic syndromes, such as the slow channel syndrome and congenital end-plate cholinesterase deficiency, and after exposure to drugs or toxins that inhibit acetylcholinesterase, a single nerve stimulation will evoke more than 1 CMAP response. In this situation, the EPP is abnormally prolonged and remains higher than the AP threshold through the refractory period of the AP. In this situation, each consecutive response is smaller than the previous one and disappears once the EPP amplitude decreases to less than the AP threshold.
Needle EMG
Conventional needle electrode EMG examinations are performed in patients suspected of having MG and other disorders of synaptic transmission to exclude diseases that may mimic or coexist with MG (and LES), such as peripheral neuropathy and inflammatory or ocular myopathies. In the absence of other neuromuscular disorders, the EMG examination will demonstrate, in both presynaptic and postsynaptic disorders of NMT, motor unit action potentials (MUAPs) that vary in configuration with consecutive discharges ( Fig. 2 ). This pattern results from intermittent failure of synaptic transmission (impulse blocking) of some of the muscle fibers that comprise the MUAP and is most easily recognized as a variation in amplitude of an isolated MUAP if a slow oscilloscope sweep speed is used. This abnormality may be partially or completely reversed in patients with MG by the administration of edrophonium. This finding may be confused with the abnormality seen in reinnervation in which motor unit instability occurs as a result of immature NMJs. However, patients with reinnervation will have large, prolonged MUAPs. In LES and, to a lesser extent, in botulism, one may see a progressive increase in the EMG envelope amplitude during sustained contraction, as a result of the facilitated release of acetylcholine (ACh) from the nerve terminal.
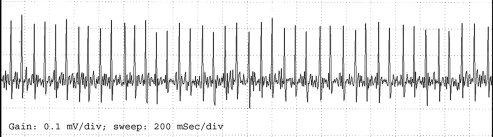
In rare situations, usually in patients with acute and severe MG, one may find fibrillation potentials, especially in the paraspinal muscles. They may also be seen in patients with severe LES, botulism, and congenital myasthenic syndromes caused by plectin deficiency. Their presence, however, should suggest to the electromyographer that there may be an associated disfigurative process. The interference pattern in patients with MG is typically full, although with sustained contraction one may see a reduction in the envelope amplitude as the muscle fatigues and impulse blocking occurs.
Needle EMG is most important in suspected cases of MG associated with antibodies to muscle-specific protein kinase (MuSK), in which MUAP changes (both myopathic and neuropathic) will be recorded in clinically affected muscles, particularly those with atrophy. Importantly, patients presenting with isolated neck extensor disease or respiratory failure may have normal electrodiagnostic studies in the limbs. It is therefore important that clinically affected muscles be examined to avoid missing the pertinent abnormalities of this disease.
RNS studies
Repetitive motor nerve stimulation has application to a wide variety of clinical disorders that affect the NMJ and is the most frequently used electrodiagnostic test of NMT. Abnormal results from RNS studies are not diagnostic of specific clinical disorders, and abnormalities may be detected in patients with multiple sclerosis, motor neuron disease, peripheral neuropathy, radiculopathy, or primary muscle membrane disease, in addition to patients with primary disorders of the NMJ such as MG, LES, arthropod envenomation, botulism, congenital myasthenic syndromes, and impaired NMT caused by certain commonly used medications (eg, antibiotics) and toxins (eg, organophosphates).
General Principles
The technique of RNS is similar to that used in conventional neurographic studies, differing only in the application of stimuli trains or paired stimuli, the use of conditioning exercise, and the careful immobilization of the limb to reduce movement artifact ( Table 1 ). The methodology of RNS studies consists of stimulating the peripheral nerve with a supramaximal stimulus (25%–50% greater than the maximum stimulation intensity necessary to activate all the nerve fibers) and recording the CMAP response with an active surface electrode (E1) over the belly of the muscle and a referential electrode (E2) over the tendon of the same muscle. The negative peak amplitude and area of the CMAP response are reflections of the numbers of muscle fibers activated by the nerve stimulus; hence, it is a marker of synaptic efficacy. It is important that measurements of amplitude and area are concordant; a discrepancy implies a technical problem. Negative peak area should not be used to assess facilitation as some authors have suggested, because there are many technical factors (eg, variable phase cancellation, repolarization, muscle fiber fatigue, and filter settings) that will impede quality recordings.
Defect Location | Stimulation Train | Stimulation Frequency | Findings | Exercise Duration | Expected Findings |
---|---|---|---|---|---|
Presynaptic | 5–10 stimuli | 2–5 Hz | >10% decrement, reduced CMAP amplitude | 10 sec | PAF >100% immediately after exercise |
Stimulation for 5–10 sec | 20–50 Hz | >100% increase in intratrain CMAP amplitude | … | … | |
Postsynaptic | 5–10 stimuli | 2–5 Hz | >10% decrement, normal CMAP amplitude | 30–60 sec | PAE at 3–4 min after exercise |
10 sec | Repair of decrement immediately after exercise |
Supramaximal stimulation must be used because failure to do so will result in a false-positive study because of pseudodecrement. Decrement is defined as the percent change comparing the negative peak amplitude or area between the fifth (or fourth or lowest potential) and the first potential. It can be calculated from the formula :
Facilitatory responses are calculated by the formula:
where n is the potential number to which the first potential is compared.
The criteria for abnormality will vary to some degree among laboratories. Most electromyographers will accept a decrement greater than 10% or a facilitatory response greater than 100% of the initial CMAP response, although the latter is now being reconsidered.
Muscle Selection
Ideally, one would like to test muscles that are involved clinically, although this is sometimes difficult. Larger muscles are, as a group, more difficult to immobilize and therefore are subject to movement artifact from displacement of either the stimulating or recording electrodes. Smaller muscles, such as those in the hand, are easy to immobilize but often are not involved clinically and most often will not show an abnormality unless the muscle is quite weak. The heterogeneous literature of RNS testing precludes drawing specific estimates of sensitivity and specificity and the literature must be interpreted with caution. However, generalizations can be made that will assist the electromyographer in developing a diagnostic strategy.
The abductor digiti quinti muscle is easily examined and is easily immobilized. Studies in this muscle are well tolerated by patients. The abductor pollicis brevis muscle is more difficult to immobilize because of the large moment of movement caused by thenar contraction. Stimulation of deep nerves requires high stimulus intensity, which may be uncomfortable to the patient and not tolerated well. One can reduce the stimulus-induced discomfort by using monopolar needle stimulation electrodes (eg, musculocutaneous nerve stimulation with biceps brachii recording). These techniques often produce movement artifact when large muscles are examined. Stimulation of the brachial plexus will often elicit contraction of several muscles, inducing movement artifact into the recording. Selective stimulation of the accessory nerve with recording over the upper trapezius muscle is often well tolerated and easy to perform, requires low stimulus intensity, and is an excellent choice for a proximal muscle. This study may be performed with the patient either sitting or lying. Facial muscles (nasalis, orbicularis oculi) studies may tend to be uncomfortable for the patient but offer the advantage of a higher diagnostic yield for bulbar and perhaps ocular disease. Most investigators begin their evaluation using a distal hand muscle, even though a greater proportion of patients with MG will have abnormalities in proximal muscles, because it allows the patient to experience the procedure with relative comfort. It would be quite unusual to have an abnormality in a distal limb muscle with a normal study in a more proximal location.
There is a paucity of evidence-based literature regarding the sensitivity and specificity of RNS testing in the diagnosis of MG. There are insufficient data to correlate abnormalities of specific nerve–muscle combinations with the clinical severity of disease or the number of nerve–muscle combinations necessary to examine to achieve the highest diagnostic accuracy. In general, the specificity of RNS is very high (∼95%) in both ocular MG and generalized MG, whereas the sensitivity of RNS in ocular MG is less than 30% and approaches 80% in generalized MG when clinically affected muscles are examined.
Immobilization
It is critically important to immobilize not only the muscle being examined but also the electrodes used to stimulate the nerve. Muscle and electrode movement artifacts are most often distinguishable by the abrupt change in waveform configuration during a train of stimuli. Failure to immobilize the tested muscle may also result in a pseudodecremental response as a result of submaximal stimulation of the nerve. Slower rates of stimulation are less likely to cause movement artifact because the muscle will return to its relaxation state before the next contraction. Faster rates of stimulation are more likely to produce movement artifact, primarily because the discomfort causes the patient to contract the muscle. Tetanic stimulation, in addition to the induced discomfort, may alter waveform configuration because of a change in the volume conduction through the muscle produced by change in its shape. Commercially made jigs to restrain movement may be quite useful, but in most instance proper attention to limb position and fixation of the limb by the examiner will suffice for the stimulation rates used in most laboratories. Often, the electromyographer only needs to gently hold the hand in place when recording from hand muscles or, when recording from the trapezius muscle, have the patient place their fingers under their hip when supine or to touch the bottom of the chair when sitting.
Temperature
Increased temperature is known to aggravate the strength of patients with MG, and control of intramuscular temperature is important in performing electrodiagnostic tests of NMT. All patients undergoing RNS studies should have their extremities warmed to at least 32°C in the leg and to 34°C in the arm. Shoulder and facial muscles do not need warming in most situations. This may be accomplished by bathing the limb to be studied in a warm bath or using a heating lamp or a thermostatically controlled radiant warmer. Failure to warm the limb properly may result in a masking of the decremental response in patients with disorders of NMT because temperature changes of only a few degrees Celsius can reverse mild decrements ( Fig. 3 ). Presynaptic disorders may be misdiagnosed because the CMAP amplitude will be large and a facilitatory response masked if the muscle is cool ( Fig. 4 ). The mechanism by which this occurs is not completely understood. Some authors postulate that it results from an increase in EPP amplitude and prolongation of EPP duration presumably as a result of alteration of the ionic channel conductance. Others suggest that cooling potentiates NMT by enhancing transmitter packaging and presentation of neurotransmitter to the release site of the nerve terminal, by reducing the hydrolysis of ACh and by increasing ACh receptor sensitivity to ACh.
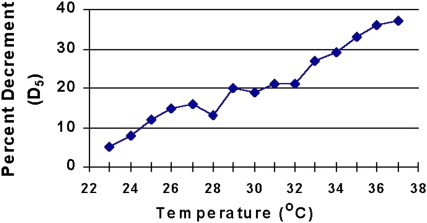
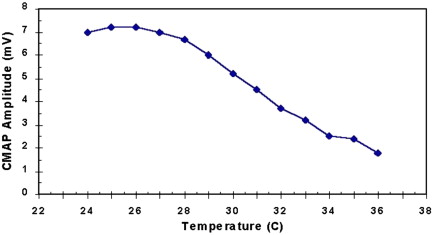
Stimulation Rates
Typically, stimulation rates of 1, 2, 3, or 5 Hz are used. Desmedt found that stimulation rates between 3 Hz and 5 Hz are most likely to produce a decremental response in MG because of the depression in quantal release of ACh with a train of stimuli. We and others have not found a difference between 2-Hz and 3-Hz stimulation. Others think that decremental responses may be missed with stimulation rates less than 7 Hz. Stimulation rates in excess of 10 Hz frequently produce artifact and should be avoided except when high-frequency, short-duration stimulation is used to demonstrate the presence of a presynaptic disorder of NMT, such as LES. Excessively fast rates of stimulation or coexisting voluntary muscle contraction may produce an increase in CMAP amplitude with a reduction in the duration of the potential duration but no change in the negative peak area, a phenomenon termed pseudofacilitation. This results from an increase in the synchronization of the propagation velocity of the muscle fibers. In normal individuals, there is no change in the CMAP amplitude with slow rates of stimulation and one may see a minimal increase (pseudofacilitation) of the CMAP amplitude at stimulus rates of 5 Hz or greater. At faster stimulus rates (40–50/sec), there is a mild facilitation of the CMAP amplitude within the first 10 stimuli and then a constant response ( Fig. 5 ). Paired supramaximal stimulations with varying interstimulus intervals have been used in the past to assess NMT. This technique is laborious and does not offer any advantages over RNS.
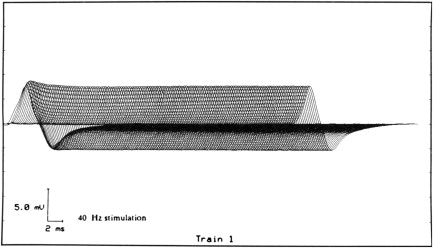
Activation Techniques and Provocative Measures
The diagnostic yield of RNS may be increased through several activation techniques or other provocative measures. This is necessary because not all muscles will show the same degree of abnormality. However, these techniques are liable to artifact because many of them require limb movement, rapid rates of stimulation, or noxious procedures.
The most commonly used muscle activation procedure involves having the patient contract the muscle maximally for 10 to 60 seconds or by delivering a high-frequency train of stimuli. Such efforts produce an accumulation of calcium in the nerve terminal, which mobilizes the release of ACh. In a presynaptic disorder, this produces a marked increase in the CMAP amplitude. This phenomenon is termed postactivation facilitation or postexercise facilitation. Following maximum voluntary contraction or tetanic stimulation, there is a depression of end-plate excitability. This response is most often seen 2 to 4 minutes after exercise and is referred to as postactivation exhaustion or postexercise exhaustion. The electrophysiologic accompaniment of this phenomenon is a worsening of the decremental response compared with the preexercise values. In some individuals, particularly those with mild disease, an abnormal decrement may be seen only during the during the exhaustion stage.
Ischemia will enhance the neuromuscular block of presynaptic and postsynaptic disease. Harvey and Masland were the first to describe the effects of ischemia on NMT. The “double-step” RNS test involves prolonged stimulation of a muscle in the hand before and after ischemia of the limb and has been proposed to increase the sensitivity of RNS. In patients with MG, this test was found to be only slightly more sensitive than RNS of the trapezius muscle alone and only 60% as sensitive as SFEMG of a hand muscle. The mechanism that accounts for the worsening decrement is not completely understood. Investigators postulate that ischemia depletes acetyl-coenzyme A, resulting in a failure of ACh synthesis, a depression of vesicle packaging, or vesicular recycling. This technique is not in common use today given the increasing use of SFEMG.
Curare and similar neuromuscular blocking agents will impair synaptic transmission at the NMJ by producing a competitive, nondepolarizing neuromuscular block. It is our belief that the use of curare should not be used in the diagnosis of MG given the sensitivity of SFEMG. The reader is cautioned that if curare is to be administered to patients with suspected neuromuscular disease, it should be done in a setting where there is appropriate critical care and respiratory support.
Quality Control in RNS Studies
As with other electrophysiologic techniques, the issue of quality control is paramount. The electromyographer must be able to review the actual waveform obtained in each train of stimuli to ensure that each is similar to the other. Electrode movement, submaximal stimulation, and muscle contraction will produce unique changes in waveform configuration that may be mistaken for either a decremental or incremental response ( Fig. 6 ). The review of only a stick diagram will not give clues as to any alteration in the waveform, and the sole use of these diagrams must not be relied on for accurate assessment of the study. Acceptable decrements, or increments, are characterized by similar or gradual changes in CMAP amplitude or area with the greatest change occurring between the first and second potentials ( Fig. 7 ). Decrements should be reproducible on repeated testing after appropriate rest periods. The lack of attention to temperature requirements in RNS testing is the most common error made rendering the study unhelpful in this author’s experience. This is most important when considering presynaptic disorders of NMT.
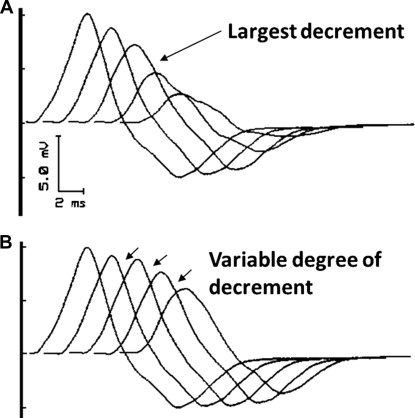
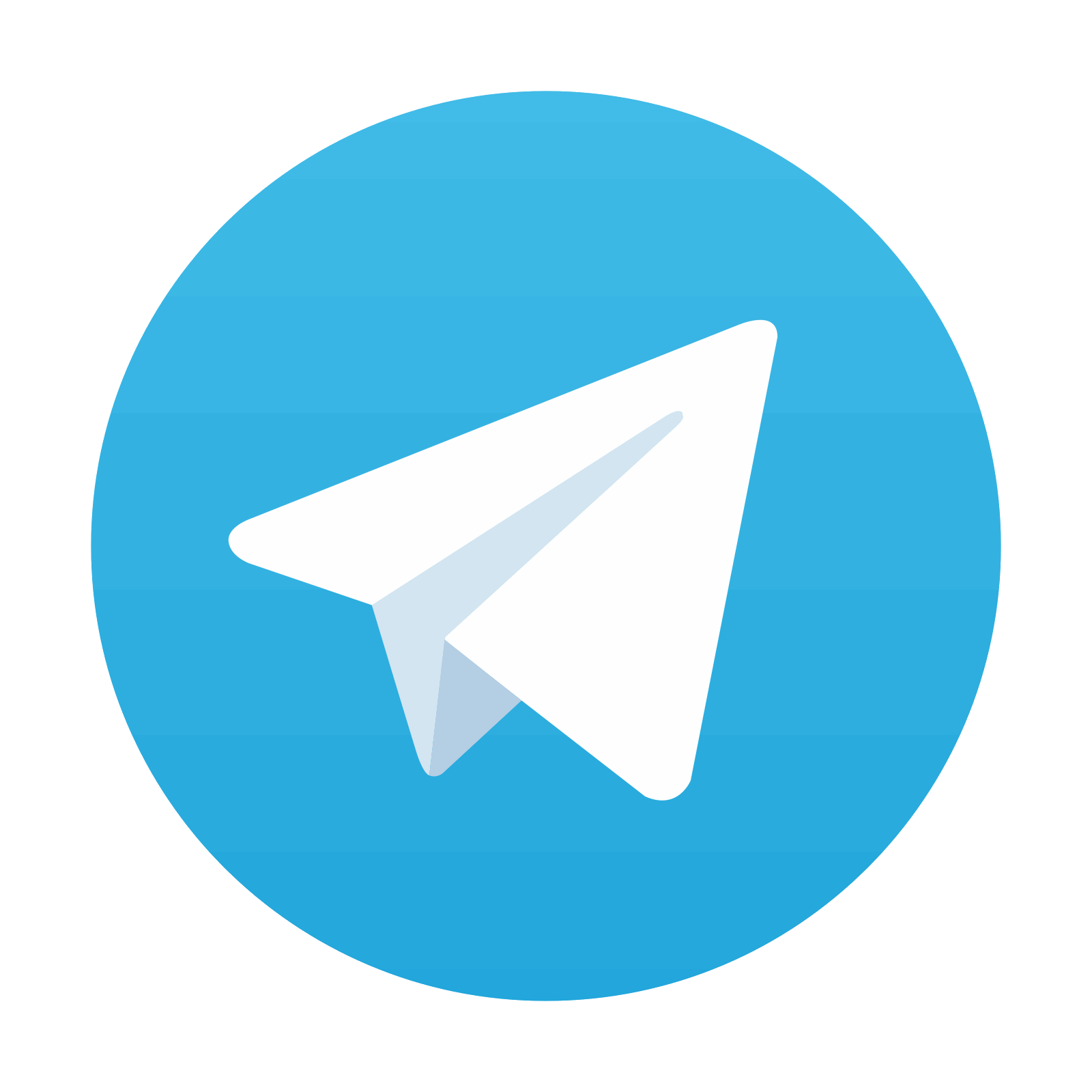
Stay updated, free articles. Join our Telegram channel

Full access? Get Clinical Tree
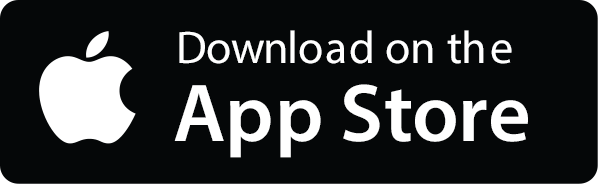
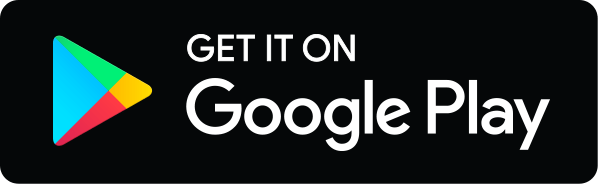
