Electrodiagnosis, which includes nerve conduction and needle electromyographic studies, is an essential element in the evaluation of peripheral neuropathies. A systematic approach to the electrodiagnostic evaluation aids in clarifying the distribution and extent of involvement, type of nerve damage, and time course. When these data are combined with clinical information, a full characterization of the neuropathy is possible leading to a sound differential diagnosis and selection of rational tests to order. This article will discuss the relevant anatomy and physiology of peripheral nerves and the principles of nerve conduction and needle electromyographic studies, including how they help distinguish between prototypic examples of primary axonal and demyelinating neuropathies, how to plan electrodiagnostic studies, and how to interpret data from outside studies.
Key points
- •
A planned approach to electrodiagnostic testing provides the most information.
- •
Electrodiagnostic testing is highly sensitive for defining the pattern and degree of nerve involvement.
- •
Electrodiagnosis can provide information on the chronicity of a neuropathy.
- •
Electrodiagnostic testing can provide insight into the underlying pathophysiology, defining the neuropathy as either primary axonal or primary demyelinating.
Introduction
In the clinical setting of a suspected peripheral neuropathy, the history and neurologic examination provide information about general features but cannot define the nature of the pathologic changes and the clinical features may underrepresent the distribution of nerve involvement or time course. Electrodiagnostic tests allow for a more detailed characterization of a neuropathy. Electrodiagnostic tests include nerve conduction and needle electromyographic (EMG) studies. Reliable interpretation of the data assumes recognition of potential technical issues and overall must be within the context of the clinical information. A systematic electrodiagnostic approach is based on an understanding of basic nerve anatomy and physiology and how pathologic changes affect electrodiagnostic data, and this foundation will be reviewed. Electrodiagnostic findings in prototypic axonal and demyelinating neuropathies are presented. These principles are put together as a diagnostic strategy to aid in planning informative tests and interpreting outside studies.
Introduction
In the clinical setting of a suspected peripheral neuropathy, the history and neurologic examination provide information about general features but cannot define the nature of the pathologic changes and the clinical features may underrepresent the distribution of nerve involvement or time course. Electrodiagnostic tests allow for a more detailed characterization of a neuropathy. Electrodiagnostic tests include nerve conduction and needle electromyographic (EMG) studies. Reliable interpretation of the data assumes recognition of potential technical issues and overall must be within the context of the clinical information. A systematic electrodiagnostic approach is based on an understanding of basic nerve anatomy and physiology and how pathologic changes affect electrodiagnostic data, and this foundation will be reviewed. Electrodiagnostic findings in prototypic axonal and demyelinating neuropathies are presented. These principles are put together as a diagnostic strategy to aid in planning informative tests and interpreting outside studies.
Peripheral nerve anatomy
A nerve consists of individual nerve fibers of different types bundled together and can be divided along several lines: somatic and autonomic fibers, motor and sensory fibers, large and small fibers. Each fiber consists of an axon insulated by segments of myelin, which is thick and tightly wrapped for large myelinated fibers and thin and loosely wrapped for small unmyelinated fibers. The functional and electrodiagnostic implications of different nerve fiber diameters and their degree of myelination is varied in nerve fiber conduction velocities. Myelinated fibers have faster velocities as a result of saltatory conduction (30–60 m/s), whereas unmyelinated fibers conduct relatively slowly (<1 m/s). Routine nerve conduction studies assess exclusively larger myelinated nerve fibers, as the contributions from smaller myelinated and unmyelinated fibers to the recorded signal are by comparison minimal. Special tests can assess these fibers but are not commonly performed and rarely help with characterization of common neuropathies.
Named (large) peripheral nerves contain all fiber types (large and small fiber somatic, autonomic, and motor and sensory nerves) before branching in the end organs, where somatic motor and sensory nerves separate and are accessible for study. Sensory nerves contain 3000 to 6000 fibers. Motor nerves frequently innervate a group of muscles, such as the thenar or hypothenar groups, and 100 to 300 motor nerve fibers innervate muscle(s) in the groups. However, each motor axon branches into 500 to 800 terminal branches within a muscle and each branch innervates a muscle fiber.
Another anatomic feature of motor nerves is the motor unit, which is the axon and its terminal branches. Terminal branches and the muscle fibers they innervate are distributed over a circular area within a muscle with a diameter of 5 to 10 mm. With more than 100 motor units innervating a muscle, the territories of approximately 20 motor units overlap at any given site in the muscle.
Principles of nerve conduction physiology
During nerve conduction studies, the entire nerve is electrically activated. Sensory and motor responses are recorded separately by the position of electrodes over sensory nerves or over muscles. The sensory response is recorded as the sensory nerve action potential (SNAP) and the motor response as the compound muscle action potential (CMAP). The SNAP represents the sum of single nerve fiber action potentials. The SNAP waveform shape is determined by the arrival times of nerve fiber action potentials, the duration of the action potentials, and the degree of phase cancellation. Nerve fiber action potential waveforms are biphasic (negative-positive) with a duration of approximately 1 millisecond. Sensory nerves conduct over a range of approximately 25 m/s. The arrival of slower conducting nerve action potentials positions their negative peaks at the time of the positive portions of the faster conducting action potentials, resulting in significant phase cancellation and a lower SNAP amplitude than might be expected from the simple algebraic addition of the nerve fiber action potentials ( Fig. 1 ). With greater conduction distances, the effect of phase cancellation is marked and the SNAP amplitude decreases greatly with longer conduction distances. The shape of the CMAP is affected by the same elements. However, motor nerves activate muscle fibers whose action potentials are approximately 6 milliseconds in duration. Further, motor nerves conduct over a smaller range of conduction velocities, approximately 15 m/s, and the slower arriving muscle fiber potentials are minimally affected by phase cancellation (see Fig. 1 ). Accordingly, the CMAP amplitude is higher and decreases little with longer conduction distances.
Another difference between sensory nerve fibers and muscle fibers is their diameters: sensory nerve fibers that contribute to the SNAP have diameters of 8 to 12 μm, whereas muscle fibers that contribute to the CMAP have diameters of 30 to 70 μm. The amplitudes of individual action potentials are proportional to the diameter of the fiber; SNAP amplitude (normal range) is 6 to 90 μV, whereas CMAP amplitude (normal range) is 2000 to 15,000 μV, or 2 to 15 mV.
Nerve stimulation is accomplished by passing current between anode and cathode electrodes (which are 3–4 cm apart) on the skin over the nerve. Current flows from the anode to the cathode with hyperpolarized beneath the anode and depolarization under the cathode (the site of impulse initiation). With sufficient current, all axons are activated to produce a maximal SNAP or CMAP response. The cathode must be placed closest to the recording site, for if the anode is placed closest, 2 situations occur. Because the distance between the electrodes is 3 to 4 cm, the intended measured distance between recording and stimulating electrodes will be approximately 3 to 4 cm greater, leading to artificially longer distal latencies or slowed conduction velocities. The other is the phenomenon of anodal block that can cause partial conduction block along the nerve caused by hyperpolarization of some axons under the anode, leading to artificially lower SNAP and CMAP amplitudes.
Nerve conduction studies yield 2 types of metrics: the amplitude of the evoked response and a set of timing measures ( Fig. 2 ). SNAP and CMAP amplitudes are roughly proportional to the number of axons conducting between the stimulating and recording electrodes. With progressive axonal loss, both responses lose amplitude but the CMAP amplitude drops less than the SNAP amplitude because of the effects of collateral reinnervation of motor nerves (to be discussed later). Timing measures generally reflect activity of the fastest myelinated fibers and include distal latency (conduction over set distances), F-wave latency (conduction over twice the whole length of motor nerves), and conduction velocity (over segments of nerve). The duration of the CMAP waveform (negative peak duration) provides an estimate of the spectrum of more slowly conducting myelinated fibers.
Nerve fiber conduction velocity is slowed at lower temperatures in a linear manner. However, the effects of temperature are more apparent with sensory than with motor nerves. With lower nerve temperatures, sensory nerve action potentials are longer in duration, resulting in less phase cancellation and larger SNAP amplitudes. Although muscle fiber action potentials also lengthen in duration, the effect on CMAP amplitude is negligible. For both, the lower nerve temperature slows conduction, approximately 2 m/s/°C. Correction factors can be applied when limb temperature is low, but the factors are approximate and diagnostic interpretation uncertainties can be avoided by warming limbs to approximately 31°C.
Principles of needle EMG
Needle EMG records electrical activity from muscle fibers to assess the integrity and architecture or arrangement within the muscle. The electrical motor unit recorded by the EMG needle is called the motor unit action potential (MUAP) and represents only a portion of the anatomic motor unit, because the electrical uptake area of the electrode is less than 1 to 2 mm in diameter. Thus, the MUAP includes only about 7 to 15 fibers of an anatomic motor unit, for both concentric and monopolar electrodes. The MUAP waveform varies with slight needle movement based on the intimate proximity of the needle tip to 1 to 3 muscle fibers of the anatomic motor unit that contribute most to the waveform amplitude and shape.
The EMG study is performed in 2 stages. The first stage is assessment for the presence of abnormal spontaneous muscle fiber activity when the muscle is at rest, and the second is assessment of MUAPs during voluntary activation of the muscle. The presence of abnormal spontaneous activity is assessed at high EMG screen display sensitivity or gain, 20 to 50 μV per division. The presence of positive waves and fibrillation potentials indicates denervation and, in the context of a peripheral neuropathy, denervation from axonal loss (neurogenic denervation). Note that the waveform differences between positive waves and fibrillation potentials depend on the relationship between the electrode tip and the spontaneously active muscle fiber and thus are of equal clinical significance. The presence of very low amplitude abnormal spontaneous activity, less than approximately 50 μV, suggests very long-standing and slowly progressive denervation.
There are about overlapping 20 motor units in the electrode’s uptake area, and during voluntary muscle activation the number of active motor units increases from zero at rest toward the 20 with increasing effort. Of note, most routine EMG studies are performed at low levels of activation so that individual MUAPs can be observed. Accordingly, during low levels of activation, the electrode records from 3 to 5 MUAPs close to the needle. At low levels of muscle activation, the recruitment pattern of MUAPs can be assessed for MUAP discharge frequencies. The EMG screen display sensitivity or gain is set at 200 μV per division and sweep speed at 10 milliseconds per division. If the screen has 10 horizontal divisions, the beam sweep time will be 10/s: thus, it is rare for a single MUAP to consistently discharge at 20 Hz (twice/sweep), and observation of several MUAPs discharging at rates greater than 20 Hz support motor unit loss. MUAP waveforms can be inspected for increased amplitude by determining if they exceed 2000 to 3000 μV, an approximate upper limit of normal for distal muscles. MUAP complexity in terms of polyphasia (>4 phases) or polyturn (>5 turns) can be assessed by a trigger and delay line, which permits individual MUAPs to be electronically captured and spread out. It is to be noted that up to 10% of motor units can be polyphasic and excessive emphasis should not be placed on occasional complex motor units.
The goal of these observations is to estimate pathophysiologic changes. In the context of a neuropathy, abnormal spontaneous activity supports denervation of muscles. The remaining motor nerve fibers sprout collateral branches to reinnervate orphaned muscle fibers and the anatomic motor increases in muscle fiber density but not in area. Reinnervated muscle fibers no longer discharge spontaneously, but abnormal spontaneous activity usually persists for 2 reasons. There is subsequent ongoing denervation from the neuropathy and the degree of collateral reinnervation is limited, all of which leads to permanently orphaned (denervated) muscle fibers. With reinnervation, the increased density of the anatomic motor unit is reflected in larger and more complex MUAPs. In neuropathies that are progressing relatively rapidly, there will be ongoing denervation, marked by abnormal spontaneous activity, mildly decreased recruitment, and complex MUAPs. In very slowly progressive neuropathies, there is sufficient time for maximal reinnervation; positive waves and fibrillation potentials from permanently orphaned muscle fibers will be of very low amplitude; and the motor units will be few in number and of very high amplitude, but not complex, because there has been sufficient time for polyphasia and polyturns to simplify. Thus, when MUAPs are observed whose amplitudes are 5 to 10 times normal (10,000–20,000 mV), consideration should be given for a hereditary neuropathy, the most slowly developing form of neuropathy. The extent of denervation (distal-proximal gradient) can be assessed by identifying the most proximal muscle with abnormal needle EMG findings.
Limits of normal
As with most biologic data, “normal limits” are derived from distributions of values from subjects who have no apparent disease. The pool of subjects should include a wide spectrum of ages and body sizes (height). How limits of normal are set is controversial, but most laboratories use upper and lower limits (ULN of distal latency and F-wave latency, LLN for amplitude and conduction velocity) set at 2 to 3 SDs, but data for the different nerve conduction metrics may not be normally distributed and other limits such as confidence intervals may be more appropriate. Although it is preferable for each laboratory to gather its own normal data, this is rarely done, and most limits are handed down. An obscure origin of a laboratory’s normal values seems unscientific, but values are generally similar. Some metrics are influenced by body habitus and, although difficult to take into account precisely, should be part of the general considerations. Thin fingers in women can result in very high amplitude digital SNAP amplitudes, and vice versa for thick fingers. Conduction velocities can be reduced and F-wave latencies in particular can be long in very tall individuals, and vice versa in very short patients. Thus, ULN and LLN should be viewed as references and not absolute values and should be interpreted in the overall clinical context. Nerve conduction metrics in the elderly are especially challenging, and although good sural responses can be obtained in the very elderly, absent responses may not be abnormal.
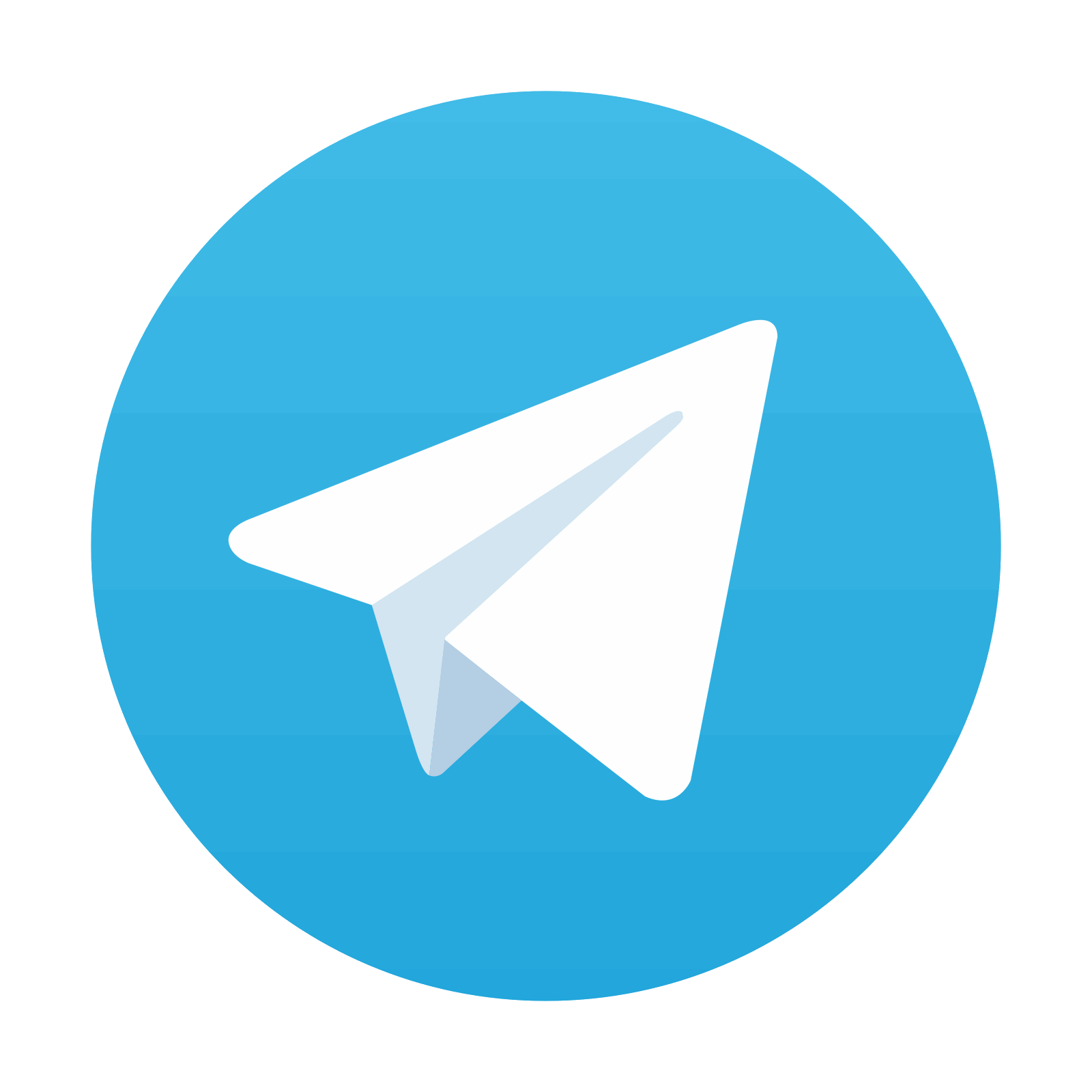
Stay updated, free articles. Join our Telegram channel

Full access? Get Clinical Tree
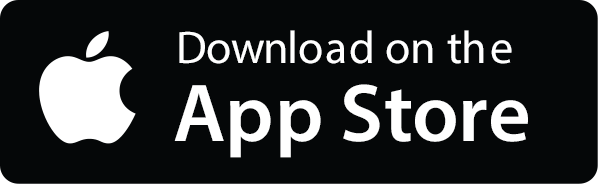
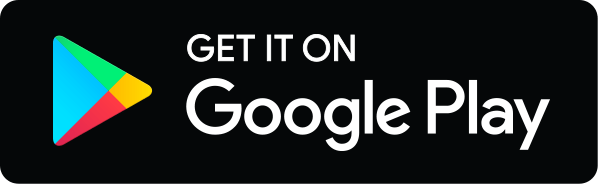