Elbow Injuries
Ronald M. Selby MD
Marc R. Safran MD
Stephen J. O’Brien MD, PLLC

The elbow joint is multifaceted and works primarily in conjunction with the shoulder to position the hand in space. It also serves as a fulcrum for the forearm lever and transmits load between the forearm and the upper arm.
Inner elbow pain may be the result of injury to the ulnar collateral ligament (UCL). Injury to the UCL may be acute, chronically disrupted, or chronically attenuated.
Treatment of UCL injury consists of a very structured approach that includes relative rest, anti-inflammatory measures, and physical therapy. With this conservative regimen, approximately 50% of throwing athletes are able to return to throwing.
The elbow of the skeletally immature athlete is susceptible to two main problems—apophyseal injury and osteochondritis dissecans (OCD). The physis is the weakest link in the skeletally immature musculoskeletal system, resulting in apophysitis and avulsion injuries, rather than ligament injuries as seen in adults. Lateral elbow pain may reflect OCD, which is particularly common in young throwing athletes and gymnasts.
Fracture or avulsion of the medial epicondylar physis can be a stress fracture or overt fracture due to repetitive valgus forces, falling, or repetitive violent forearm flexor muscle contraction in the skeletally immature athlete.
Abnormal stresses of the triceps attachment on the olecranon apophysis can result in an injury syndrome similar to Osgood-Schlatter disease of the knee.
The ulnar nerve is the most commonly injured nerve around the elbow.
Acute tendon ruptures about the elbow often require surgical reattachment. They may be treated with protection, rest, ice, compression, and elevation, along with medications (PRICEM), as long as it does not interfere with, or delay, surgical reattachment.
Pain at the triceps insertion at the proximal olecranon is common in athletes who lift inordinate amounts of weight or participate in explosive field events such as shot put. However it is also seen in javelin throwing and baseball pitching.
Lateral epicondylosis (tennis elbow) is arguably the most common source of elbow pain in the general population. Lateral epicondylosis, which also affects nontennis players, is a chronic, noninflammatory condition of the extensor muscles, primarily the extensor carpi radialis brevis (ECRB), due to overuse.
Athletes participating in overhead sports such as tennis, volleyball, swimming, weightlifting, gymnastics, and especially throwing activities, subject their elbows to repetitive stresses most marked in specific phases of the activity. These athletes are vulnerable to injuries and present with a myriad of problems including tendonitis, bursitis, nerve injuries and instability. Repetitive overhead stress to the elbow can and does cause cumulative injuries to soft tissue static stabilizers, with recurrent micro injuries to the primary stabilizers including the UCLs and capsule.
The athlete involved in throwing (baseball, javelin, water polo, football quarterback) and overhead sports (such as volleyball and tennis) is most often exposed to chronic repetitive valgus stress at the elbow.
Although pitching a baseball places the elbow at some risk, application of the proper pitching mechanics can assist in minimizing potential risk for injury while maximizing performance.
The elbow is the second-most commonly dislocated major joint in patients of all ages. In children under the age of
10 years, the elbow is the most commonly dislocated major joint.
Medial epicondylar fractures may be the result of an acute injury or repetitive overuse. Skeletally immature overhead throwing athletes may develop an injury to the medial epicondylar growth plate, because the apophysis is the weakest link in the growing musculoskeletal unit.

The elbow joint is formed by an articulation at the distal end of the humerus with the radius and the ulna and by an articulation of the radius with the ulna. Thus, the elbow joint is a composite of three articulations composed of a hinge (ginglymus) joint and a pivot (trochoid) joint (Figs 23-1 and 23-2). Flexion and extension of the forearm in the frontal plane take place at the humeroulnar articulation, while pronation and supination occur at the articulation of the radius with the ulna (the radio-ulnar joint) (Fig 23-3). The distal humerus is triangular in shape and composed of two condyles covered with a continuous layer of hyaline cartilage supporting the joint surfaces of the spool-shaped trochlea which articulates with the reciprocally saddle-shaped trochlear notch of the proximal ulna and the rounded capitellum (Fig 23-3), which articulates with the slightly concave superior end of the radial head (Fig 23-4). The capitellum is limited to the anterior and inferior surface of the distal humerus, while the trochlea is present anteriorly, inferiorly, and posteriorly. Stability of the elbow joint is largely a function of the bony congruity of the humerus, ulna, and radius, with contributions from some ligaments and muscles. In the distal humerus, the coronoid fossa anteriorly and olecranon fossa posteriorly are divided by a thin layer of bone. The proximal ulna is composed of two processes, the olecranon and the coronoid with the trochlear notch between them. The proximal radius is composed of the radial head that articulates with the capitellum, the radial neck, and a tuberosity onto which inserts the biceps tendon. The humerus is a tubular bone that articulates with the shoulder proximally and the elbow distally. Superior to the trochlea, the medial epicondyle provides the attachment for the flexor-pronator group of forearm muscles and the medial
collateral ligament. Superior to the capitellum, the lateral epicondyle is not as prominent as the medial epicondyle, which serves as the site for forearm extensor and supinator muscles attachment.
collateral ligament. Superior to the capitellum, the lateral epicondyle is not as prominent as the medial epicondyle, which serves as the site for forearm extensor and supinator muscles attachment.
![]() Fig 23-1. Anterior osseous anatomy. (Reprinted with permission from Agur AMR, Dally AF II. Grant’s Atlas of Anatomy. 11th ed. Philadelphia: Lippincott Williams & Wilkins; 2005:498. ) |
![]() Fig 23-2. Posterior osseous anatomy. (Reprinted with permission from Agur AMR, Dally AF II. Grant’s Atlas of Anatomy. 11th ed. Philadelphia: Lippincott Williams & Wilkins; 2005:499. ) |
![]() Fig 23-3. Elbow osseous articulating anatomy. (Reprinted with permission from Agur AMR, Dally AF II. Grant’s Atlas of Anatomy. 11th ed. Philadelphia: Lippincott Williams & Wilkins; 2005:524. ) |
Just superior to the capitellum, the radial fossa is found on the lateral humerus. This fossa accommodates the radial head in forearm flexion. The coronoid fossa is located medial to the radial fossa in the midline of the anterior distal humerus and similarly accommodates the coronoid process in forearm flexion. The olecranon fossa up is found posteriorly, midline in the distal humerus, and provides space for the olecranon process during extension. These fossae are bordered by the robust lateral supracondylar column and a smaller medial supracondylar column.
The ulna is asymmetrical with a cylindrical cross section at the wrist changing from a triangular cross section in its midshaft to its thickened proximal section (Fig. 23-4). The greater sigmoid notch of the ulna comprises a 190-degree arc and articulates with the trochlea of the humerus. The sigmoid notch is composed of the coronoid process at its distal extent with an articular and cortical surface where the brachialis muscle and the oblique cord insert and the olecranon at its proximal extent where the triceps muscle inserts. The medial portion of the coronoid serves as the origin for the medial part of the pronator teres, flexor pollicis longus, flexor digitorum profoundus, and flexor digitorum superficialis. The anterior portion of the medial collateral ligament of the elbow inserts onto the medial aspect of the coronoid process. The lateral surface of the coronoid process is composed of a radial notch, which serves as a site for articulation with the radial head. The radial head is stabilized by the annular ligament that surrounds it and attaches to the anterior and posterior margins of the notch. From the inferior portion of the notch, a depression extends distally, which serves as the origin of the supinator muscle. The medial collateral ligament inserts onto a tuberosity at the proximal extent of this depression.
The radius has a cylindrical cross section proximally and tapers to an elliptical cross-section distally. The radial head, held securely by the annular ligament, has a proximal concavity for articulating with the capitellum. The anterior face of the radial tuberosity is covered by a bursa that protects the biceps tendon during pronation. The biceps brachii tendon attaches to the posteromedial surface of the radial tuberosity and thereby plays a role in flexion as well as supination of the forearm. The interosseous membrane is found between the medial crest of the radius and the lateral crest of the ulna.
The humeroulnar, radiohumeral, and proximal radio-ulnar joints are all enclosed within one synovial and fibrous capsule (Fig 23-5). Elbow flexion is constrained by the trochlea articulating with the trochlear notch of the ulna. Articular cartilage covers an arch of 300 to 330 degrees on its anterior, distal, and posterior surfaces and is responsible for absorbing compressive forces (1,2). This allows for a tongue and groove, captive interface with the proximal ulna. The trochlear is not symmetrical and allows for approximately 5 degrees of internal rotation in early flexion and approximately 5 degrees of external rotation in terminal flexion. The medial lip is larger and protrudes more distally than the lateral. The two lips are partitioned by a helical groove that traverses in an anterolateral to posteromedial fashion. The capitellum of the distal humerus is spherical and covered with a thick articular cartilage. The posteromedial capitellum is marked by a prominent tubercle separating it from the lateral lip of the trochlea, where the radial head articulates through the entire range of flexion. The orientation of the articular surface of the distal humerus is rotated anteriorly about 30 degrees with respect to the longitudinal axis of the humerus. The center of the arc formed by the trochlea and capitellum is on a line that is coplanar to the anterior distal cortex. Therefore, 5 to 7 degrees of internal rotation is observed at the distal humeral articular surface with respect to the midline to the humeral epicondyle. In a frontal plane there is about 6 degrees of valgus tilt of the condyles with respect to the longitudinal axis of the humerus. This articular surface accounts for more that 50% of elbow stability in extension and more than 70% of elbow stability when the elbow is flexed 90 degrees.
![]() Fig 23-5. Bursae and synovium of the elbow. (Reprinted with permission from Agur AMR, Dally AF II. Grant’s Atlas of Anatomy. 11th ed. Philadelphia: Lippincott Williams & Wilkins; 2005:524. ) |
Articular cartilage covers the radial depression anteriorly within the radial head. This concavity has an arc of about 40 degrees (2). Additionally, 240 degrees of the outside circumference of the radial head articulates with the ulna. The lesser trochlea notch forms an arc of approximately 60 to 80 degrees delegating the remaining 160 to 180 degrees to pronation and supination (3). The head is largely covered with articular cartilage with the exception of the anterolateral one-third of the circumference of the head. This part of the radial head is devoid of subchondral bone and does not have the same structural integrity as covered areas. The head and neck of the radius form a 15-degree varus angle with the distal portion of the radius relative to the midline distally.
The trochlear notch is divided medially and laterally by a ridge that forms four quadrants. The sigmoid notch is situated approximately 30 degrees posterior to the longitudinal axis of the ulna, which corresponds to the 30-degree inferior rotation of the distal humerus. This allows for stability. Anteriorly, 1 to 6 degree rotation of the shaft lateral to the articulating surfaces contributes to the carrying angle (1). Laterally, the notch forms a 190-degree elliptical arc (3).
The congruous bony anatomy affords stability to the elbow joint. Medial (ulnar) and lateral collateral ligament complexes (LCLCs) comprise the ligaments of the elbow and provide stability in varying degrees of flexion to varus, valgus, and rotational forces. The ulnar collateral ligament (UCL)
complex is composed of the anterior oblique, posterior oblique, and transverse ligaments (Fig 23-6). This is the primary stabilizer of the elbow to valgus stress. Within the UCL complex the anterior bundle is the most discrete component composed of anterior and posterior bands. These bands tighten in reciprocal fashion as flexion and extension of the elbow occurs. The posterior bundle is a thickening of the posterior capsule and defined well only with the elbow in about 90 degrees of flexion. The transverse ligament appears to contribute little to elbow stability because its fibers span the ulnar border of the trochlea notch from the coronoid process to the olecranon. The UCL originates from the anteroinferior surface of the medial epicondyle. The anterior oblique ligament (AOL) is the primary stabilizer of the elbow and resists valgus stress between 20 and 120 degrees of flexion (4,5,6). The AOL is composed of two bands (Fig 23-7). The anterior and posterior oblique ligaments have distinct biomechanical roles. The anterior band is the primary restraint to valgus stresses at 30, 60, and 90 degrees of flexion and a co-primary restraint at 120 degrees of flexion (7). The posterior band functions as a secondary restraint to valgus stress with the elbow held at 120 degrees of flexion (7).
complex is composed of the anterior oblique, posterior oblique, and transverse ligaments (Fig 23-6). This is the primary stabilizer of the elbow to valgus stress. Within the UCL complex the anterior bundle is the most discrete component composed of anterior and posterior bands. These bands tighten in reciprocal fashion as flexion and extension of the elbow occurs. The posterior bundle is a thickening of the posterior capsule and defined well only with the elbow in about 90 degrees of flexion. The transverse ligament appears to contribute little to elbow stability because its fibers span the ulnar border of the trochlea notch from the coronoid process to the olecranon. The UCL originates from the anteroinferior surface of the medial epicondyle. The anterior oblique ligament (AOL) is the primary stabilizer of the elbow and resists valgus stress between 20 and 120 degrees of flexion (4,5,6). The AOL is composed of two bands (Fig 23-7). The anterior and posterior oblique ligaments have distinct biomechanical roles. The anterior band is the primary restraint to valgus stresses at 30, 60, and 90 degrees of flexion and a co-primary restraint at 120 degrees of flexion (7). The posterior band functions as a secondary restraint to valgus stress with the elbow held at 120 degrees of flexion (7).
![]() Fig 23-7. Ligaments of the medial elbow. (Reprinted with permission from Agur AMR, Dally AF II. Grant’s Atlas of Anatomy. 11th ed. Philadelphia: Lippincott Williams & Wilkins; 2005:526. ) |
![]() Fig 23-8. The lateral collateral ligament complex. (Reprinted with permission from Agur AMR, Dally AF II. Grant’s Atlas of Anatomy. 11th ed. Philadelphia: Lippincott Williams & Wilkins; 2005:527. ) |
The LCLC encompasses the lateral collateral, lateral ulnar collateral, and annular ligaments (Fig 23-8). The lateral complex consists more of a blend of poorly demarcated fan-shaped ligamentous fibers compared to the discrete bands of the medial collateral ligament complex. The function of the lateral collateral ligament is to provide an external rotation and varus stability as the elbow flexes. The LCLC effect gradually increases as the elbow flexes toward 110 degrees. The lateral collateral ligament origin is from the entire inferior surface of the lateral epicondyle. This is near the axis of rotation and subsequently remains taut throughout flexion and extension. The fibers of the lateral collateral ligaments and the lateral UCLs cannot be separated proximally. The lateral UCL is the posterior portion of the lateral collateral ligament. The annular ligament attaches to the anterior and posterior margins of the lesser sigmoid notch. It becomes taut during supination at its anterior insertion and becomes taut at the extremes of pronation at its posterior insertion. This ligament is funnel shaped and assists in stabilizing the proximal radius throughout the range of pronation and supination.
The blood supply to the elbow is composed of the vessels that comprise the collateral circulation about the elbow. This is from the superior and inferior ulnar collateral arteries on the medial side superiorly and the two ulnar recurrent arteries inferiorly (Fig 23-9). Laterally, the radial recurrent and middle collateral arteries of the profunda brachii supply the joint superiorly and the radial and interosseous recurrent arteries inferiorly.
Nervous innervation to the elbow joint arises typically from all four nerves that cross the joint (Fig 23-10). In general, the distribution of each nerve to the joint is overlapped by the distribution of branches from other nerves (9).

Elbow function is an inherent component of the normal use of the arm in daily activities and athletic endeavors. The elbow joint is multifaceted and works primarily in conjunction with the shoulder to position the hand in space. It also serves as a fulcrum for the forearm lever and transmits load between the forearm and the upper arm. The structural makeup of the bony articulations, ligaments, and capsule contribute to the elbow’s renowned stability. As the anatomic link between the humerus and forearm, the elbow is consistently subjected to intense compressive, tensile, and shear forces (Fig 23-11). Effects of the culmination of these forces can be seen in repetitive activities; this effect is magnified in the overhead athlete. Stiffness or instability of the elbow can severely compromise the function of the entire upper extremity. An understanding of the biomechanical function and kinematics of the elbow is paramount for treating physicians.
Flexion and extension about a transverse axis is permitted by the humeral trochlea serving as a pulley for the trochlear notch of the ulna. The radial head glides along the articular surface of the capitellum during flexion and extension without influencing the path of motion. Elbow flexion is limited by contact of the biceps brachii muscle and other soft tissue structures. Forearm extension is limited by the olecranon process of the ulna when it contacts the olecranon fossa of the humerus. Posteriorly the margins of the trochlea extend obliquely superiorly and laterally so that when the forearm is extended it deviates laterally rather than in line with the upper arm and thereby forms the carrying angle of the elbow.
The normal range of flexion and extension of the elbow is 135 degrees (10). Rotation as well as varus and valgus forces of the elbow are limited by the inherent bony stability as well as a significant restraint to varus and valgus forces from the collateral ligaments. The radio-ulnar joint plays a role in pronation and supination. The axis of rotation runs through the center of the radial head and styloid process of the ulna. During supination and pronation, the ulna remains fixed while the radius rotates internally during pronation or externally in supination. Supination is limited largely by ligaments between the ulna and carpal bones. Pronation is limited by soft tissues on the anterior radius and ulna. The normal range is about 80 degrees of supination and 80 degrees of pronation.
The elbow is in essence a slightly loose hinge with small amounts of varus, valgus, and rotational laxity throughout its range of motion. The center of the arc formed by the trochlea and capitellum is on a line that is coplanar to the anterior distal cortex. Therefore, 5 to 7 degrees of internal rotation is observed at the distal humeral articular surface with respect to the midline to the humeral epicondyle. In a frontal plane
there is about 6 degrees of the valgus tilt of the condyles with respect to the longitudinal axis of the humerus. This articular surface accounts for more that 50% of elbow stability in extension and more than 70% of elbow stability when the elbow is flexed 90 degrees.
there is about 6 degrees of the valgus tilt of the condyles with respect to the longitudinal axis of the humerus. This articular surface accounts for more that 50% of elbow stability in extension and more than 70% of elbow stability when the elbow is flexed 90 degrees.
The range of motion of the elbow is composed of extension to about zero to 5 degrees, flexion to approximately 145 degrees, supination to 85 degrees, and pronation to about 75 degrees (Fig 23-12). The normal valgus angle at the elbow (in full extension this is known as the carrying angle) is about 5 degrees in males and 10 to 15 degrees in females. Bony and soft tissue constraints contribute to the noted stability of the elbow joint. The bony structure resists valgus and varus forces and shows increased stability in extension. Static stability is also enhanced by the medial and lateral collateral ligaments and the joint capsule. This is especially noted in flexion. Dynamic stabilization is also aided by several muscles that cross the elbow joint. The primary constraint to valgus forces of the elbow is the anterior bundle of the UCL. During overhead motion, tensile forces are applied across the medial aspect of the elbow, compressive forces across the lateral aspect of the elbow, and valgus extension forces in the posterior aspect of the elbow.
The biomechanics of pitching involves coordination of the entire body with sequential activation of different body parts to culminate in maximum velocity at ball release. The motion of the arm in throwing, and this is repeatedly magnified in pitching, is rapid and violent. Elbow injuries in pitching are fairly common. The peak angular velocity during throwing has been measured to be 6,180 degrees per second for shoulder internal rotation and 4,595 degrees per second for elbow extension (11). Peak elbow acceleration has ranged from 225,000 to 500,000 degrees per second. Stresses at the elbow occur during acceleration and follow-through phases, which last approximately 15 milliseconds. During this time period the arm undergoes organized deceleration, which requires normally functioning elbow musculature to prevent the articular and ligamentous stabilizers from having to absorb all the force. The pitching motion is divided into five phases to more easily enable study of the biomechanics of the elbow during pitching. During the windup and stride phases, the elbow is not subjected to significant forces. In the arm-cocking phase, contraction of the wrist flexor pronator group generates varus torque to counter the valgus extension loading of the UCL, which is not strong enough to resist the torque by itself (12). The anconeus and triceps are also active during this phase to decrease the stress on the UCL by compressing the joint and conferring dynamic stability. During acceleration, triceps activity increases and biceps activity decreases. When the shoulder arm reaches maximum internal rotation,
the deceleration phase begins with increased contraction in the biceps, triceps, reflectors, and wrist extensors to counter wrist flexion. The trunk and legs help to dissipate forces during the follow-through phase. (See Throwing Mechanics and the Elbow section.)
the deceleration phase begins with increased contraction in the biceps, triceps, reflectors, and wrist extensors to counter wrist flexion. The trunk and legs help to dissipate forces during the follow-through phase. (See Throwing Mechanics and the Elbow section.)

Ligament injuries of the elbow have been of particular interest to the sports physician with increasing amounts of research and literature being written in recent years. Due to better understanding and awareness, the diagnosis of ligament injuries is becoming more common. Particularly for the UCL, it is now understood that subtle degrees of laxity are the hallmark of injury to this ligament, thus careful examination, in addition to newer, advanced imaging techniques, help in the ability to diagnose this problem. On the lateral side, enhanced understanding of the lateral ligament complex, its role in injury and subsequent disability was brought to the forefront by O’Driscoll in the early 1990s and is now recognized as a major factor in the ligamentous stability of the elbow.

Inner elbow pain may be the result of injury to the UCL. Injury to the UCL may be acute, chronically disrupted, or chronically attenuated. Waris (13) was the first to recognize and describe UCL tears, particularly isolated anterior oblique ligament disruption, in javelin throwers half a century ago. Early publications suggested that UCL tears are a rare lesion; however, with better understanding, evaluation, and imaging of the UCL, it is clear that UCL injury is not uncommon. Individuals who sustain an acute UCL rupture, note sudden onset of medial elbow pain, with or without a popping sensation, that occurred with throwing or falling. Usually the individual notes they were unable to throw after the injury and may complain of symptoms of ulnar nerve irritation.
Individuals with chronic elbow valgus instability from either complete disruption or attenuation of the UCL give a history of having pain or soreness along the inner elbow with throwing. The number one cause of UCL injuries in the throwing athlete is overuse, or repetitive microtrauma (14). The athlete gives a history of repeated bouts of inner elbow pain during and after throwing that would respond to conservative treatment. However, with the final injury, the athlete notes that he or she is unable to throw greater than 75% of maximal capacity due to this pain (14). The athlete may note, however, that there was a single “giving way” episode or sudden severe inner elbow pain, which was distinct and isolated and likely represents the final insult. The throwing athlete most commonly complains of pain at the acceleration phase of throwing and with ball release or point of impact in hitting the ball being the second-most commonly reported timing of pain (15). Due to chronicity of the instability, the athlete may have ulnar nerve irritation due to stretching of the nerve with the increased valgus laxity or symptoms of medial epicondylitis (due to attempts at muscular stabilization of the medial elbow), or symptoms of loose bodies, such as catching or locking.
On physical examination, these individuals will have point tenderness 2 cm distal to the medial epicondyle, over the UCL itself, and pain and instability with valgus stress (Fig 23-13). The athlete may have pain with resisted wrist flexion, indicative of medial epicondylitis, which can co-exist with UCL injury. However, the absence of increased pain with resisted wrist flexion and the location of pain posterior to the common flexor muscle origin help differentiate this problem from flexor-pronator muscle rupture. In some cases, ecchymosis may appear at the medial joint line and
proximal forearm 2 to 3 days after an acute injury. With a torn UCL, the medial joint gaps opens with valgus stress and the ulnar nerve is stretched. Baseball pitchers and tennis players often have long-standing fixed-flexion and valgus deformities, which are static deformities that may predispose to traction neuritis (16,17).
proximal forearm 2 to 3 days after an acute injury. With a torn UCL, the medial joint gaps opens with valgus stress and the ulnar nerve is stretched. Baseball pitchers and tennis players often have long-standing fixed-flexion and valgus deformities, which are static deformities that may predispose to traction neuritis (16,17).
Examination to determine the functional integrity of the UCL is performed with the humerus stabilized and a valgus force applied to the elbow. This can be done classically with the elbow flexed 30 degrees, while holding the patient’s hand between the examiner’s elbow and body (Fig 23-13). The author finds this test to be a bit less useful than some of the following tests because (a) humeral rotation is poorly controlled, making examination difficult, if not confusing, and (b) the UCL is most autonomous in resisting valgus force at 70 to 90 degrees of elbow flexion (18,19). Thus, other tests utilized include the “milking maneuver,” which was described by Veltri et al. (20) produces a valgus stress to the joint in greater flexion (Fig 23-14). The elbow being tested is flexed beyond 90 degrees, and the opposite hand of the patient is placed under the elbow to grasp the thumb of the affected hand, thereby exerting a valgus stress on the UCL. The examiner palpates the UCL tenderness, joint line gapping, and quality of endpoint during this maneuver (20,21). This technique of examination was advocated based on the increased valgus rotation of the elbow at 90 degrees compared with 30 degrees when using the normal elbow as the control (22). Several authors prefer a modification of this technique, where the patient’s elbow is flexed 70 degrees with the humerus in maximal external rotation (23). The examiner pulls on the subject’s thumb and also palpates the medial joint line with the thumb of the examiner’s other hand (Fig 23-15). This allows for evaluation of pain with stress testing, as well as medial joint gapping and quality of endpoint at the degree of elbow flexion deemed most important in cadaveric testing (18). As with all the above tests, medial joint gapping is usually only a few millimeters with complete, isolated UCL injuries (24).
![]() Fig 23-14. Milking maneuver as described by Veltri et al. (20). The patient’s contralateral extremity helps lock the shoulder of the elbow being examined. While pulling down on the thumb of the elbow being examined, a valgus stress is imparted on the elbow and the examiner palpates the medial joint line of gapping. The asterisk denotes the medial joint line of the elbow being examined. |
Recently, another test has been described to identify UCL insufficiency, the Moving Valgus Stress Test described by O’Driscoll et al. (25) (Fig 23-16). In this examination, the athlete’s shoulder is placed in an abducted and externally rotated position. The elbow is then flexed and extended while imparting a valgus force to the elbow. For those athletes with UCL insufficiency, pain is felt at a specific and reproducible point within the arc of 80 to 120 degrees of elbow flexion. This examination reproduces the pain of throwing in the athlete, since the shearing force is applied to the attenuated UCL in a similar way as with throwing.
Though ligaments are not radiodense, radiographs still may help confirm the diagnosis or provide clues of UCL injury. A small avulsion fracture fragment may be identified radiographically, confirming the diagnosis. Secondary findings on plain films suggestive of chronic UCL insufficiency include ossification within the ligament (18%) (26), loose bodies in the posterior or lateral compartments, marginal osteophytes about the radiocapitellar or ulnohumeral articulations, or olecranon and condylar hypertrophy—this latter finding more common in throwing athletes or tennis players, and not necessarily in UCL-injured elbows. UCL injury may
be confirmed with the use of stress radiographs (Fig 23-17). In the acute setting, stress radiographs may be difficult due to guarding and may require anesthesia. A commercially available stress device enables evaluation of the UCL with consistent elbow flexion, rotation, and forces, resolving a problem seen with manual stress testing. This mechanical device has been reported to be 94% sensitive and 100% specific in diagnosing UCL tears, though these results have yet to be confirmed (27). Further, it should be noted that there is increased laxity of the dominant elbow to valgus stress (nearly 0.5 mm) in uninjured, asymptomatic professional baseball pitchers when compared with their nondominant elbow (28). This is distinctly different from the general population where there is a lack of difference (up to 0.5 mm) in laxity between the dominant and nondominant elbow (29,30). Thus, there may be a continuum of elbow laxity in overhead athletes, blurring the line between asymptomatic laxity and symptomatic instability by this relatively sensitive method. Because this difference is so small, injuries to the UCL may be underestimated and misdiagnosed initially (31). Conway (32) presented his initial findings of stress radiographic findings in professional baseball pitchers and found that 2 mm of relative increased laxity and 3 mm of absolute medial joint gapping were consistent with an incompetent UCL in his series.
be confirmed with the use of stress radiographs (Fig 23-17). In the acute setting, stress radiographs may be difficult due to guarding and may require anesthesia. A commercially available stress device enables evaluation of the UCL with consistent elbow flexion, rotation, and forces, resolving a problem seen with manual stress testing. This mechanical device has been reported to be 94% sensitive and 100% specific in diagnosing UCL tears, though these results have yet to be confirmed (27). Further, it should be noted that there is increased laxity of the dominant elbow to valgus stress (nearly 0.5 mm) in uninjured, asymptomatic professional baseball pitchers when compared with their nondominant elbow (28). This is distinctly different from the general population where there is a lack of difference (up to 0.5 mm) in laxity between the dominant and nondominant elbow (29,30). Thus, there may be a continuum of elbow laxity in overhead athletes, blurring the line between asymptomatic laxity and symptomatic instability by this relatively sensitive method. Because this difference is so small, injuries to the UCL may be underestimated and misdiagnosed initially (31). Conway (32) presented his initial findings of stress radiographic findings in professional baseball pitchers and found that 2 mm of relative increased laxity and 3 mm of absolute medial joint gapping were consistent with an incompetent UCL in his series.
![]() Fig 23-17. Stress radiograph showing valgus laxity indicative of rupture of the ulnar collateral ligament complex. |
The gravity stress test is of historical significance to determine instability of the elbow, but is not used much anymore (33,34). The gravity stress test is performed with the patient supine and the shoulder brought into maximal external rotation with the sagittal plane of the elbow parallel to the floor (Fig 23-18). There is no support under the forearm allowing the weight of the forearm, resisted only by the flexor-pronator muscle group and the UCL, to work with gravity to apply a valgus stress. A standard anteroposterior (AP) radiograph is taken, enabling assessment of medial joint gapping or displacement of a medial elbow fragment (following avulsion of the medial epicondyle). A standard arthrogram was also classically utilized, particularly in the acute injury; however, its value was lost in the chronic setting because dye leakage from the ruptured medial capsule is usually not seen in cases of chronic UCL insufficiency. On the other hand, computed tomographic (CT) arthrogram is quite sensitive and specific for acute and chronic injuries to the UCL, as reported in one study of 25 baseball players (35). The advantage of the CT arthrogram is in visualizing a partial or undersurface tear of the UCL (35,36). The role of CT arthrography must also still be confirmed and clarified. The role of magnetic resonance imaging (MRI) is more clear and can be of help in identifying torn UCL as well. MRI has been shown to be helpful in identifying UCL injury (35,37,38). The MRI visualizes the ligament directly, including acute and chronic injury, and can demonstrate secondary structures that may be injured, including the insertion of the UCL or radiocapitellar overload, by demonstrating bony edema and chondral thinning. Timmerman et al. (35) found the MRI was 57% sensitive and 100% specific. However, Thompson et al. (39) found the MRI to be positive in 79% of athletes undergoing UCL reconstruction, but “falsely” negative in 21%. The addition of intra-articular contrast to the MRI improves the ability to detect UCL injuries, while maintaining the benefit of visualization of other bony and soft tissue elbow structures. Azar et al. (40) reported that MRI arthrography was 97% sensitive in detecting UCL injury, including partial undersurface UCL tears.
Dynamic ultrasonography has recently been studied in asymptomatic major league professional baseball players to evaluation the UCL of the elbow. Dynamic ultrasound revealed that this modality provides a rapid means for evaluating the UCL. In these baseball players, dominant UCL had a thicker anterior band, and the UCL was more likely to have hypoechoic foci and/or calcifications. Further, dynamic ultrasound demonstrated increased medial elbow laxity with valgus stress (41,42).
Timmerman and Andrews described the arthroscopic valgus stress test of the elbow and have found this to be the most sensitive and specific way to diagnose UCL disruptions in their hands. With this test, the authors arthroscopically visualize the medial compartment of the elbow while applying a valgus stress at 70 degrees. Timmerman et al. (35) noted that if the medial compartment gaps open, UCL insufficiency exists. Field and Altchek (24) confirmed in vitro that valgus stability of the elbow is best assessed in 60 to 75 degrees of elbow flexion. They found that rupture of the anterior bundle resulted in only 1 to 2 mm of medial joint gapping when viewed arthroscopically, whereas complete release of the UCL resulted in only 4 to 10 mm of medial joint opening. Thus, history and physical examination remain the mainstay for diagnosis and imaging studies may help confirm injury.
Recently, Safran et al. (43) reported their findings with a custom, noninvasive, nonradiographic stress test device. The authors developed a testing device based on in vitro studies (19) demonstrating that valgus stress testing of the elbow in 50 or 70 degrees of flexion and neutral rotation was the best position to demonstrate laxity after injury to the UCL of the elbow (19). The measurement of elbow laxity was based on angular displacement of the forearm. Then, in a comparative study of both a commercially available stress radiographic device and the noninvasive elbow tester, two groups
of patients were evaluated (43). Seven patients had a history of isolated UCL injury treated conservatively by the senior author. These seven were able to “cope” with their UCL injury on one elbow and the other elbow was normal. The second group was 11 patients with no history of elbow injury or surgery. The noninvasive elbow testing device was able to demonstrate a side-to-side difference between the injured and noninjured elbow, whereas the radiographic stress device was unable to show a difference. Further, neither device demonstrated a side-to-side difference between the normal elbows of the controls, as one would expect.
of patients were evaluated (43). Seven patients had a history of isolated UCL injury treated conservatively by the senior author. These seven were able to “cope” with their UCL injury on one elbow and the other elbow was normal. The second group was 11 patients with no history of elbow injury or surgery. The noninvasive elbow testing device was able to demonstrate a side-to-side difference between the injured and noninjured elbow, whereas the radiographic stress device was unable to show a difference. Further, neither device demonstrated a side-to-side difference between the normal elbows of the controls, as one would expect.
Treatment of UCL injury consists of aggressive therapy with relative rest, anti-inflammatory measures, and physical therapy. With this conservative regimen, approximately 50% of throwing athletes are able to return to throwing (17,44). The most recent study of nonoperative treatment of 31 UCL injuries in throwing athletes demonstrated that 42% of throwing athletes were able to return to their previous level of activity at an average of 24 weeks (13 to 54 weeks) (44). Jobe prescribes two cycles of 3 months of rest from throwing and rehabilitation, and if pain occurs when throwing over 75% capacity, surgery is indicated; however, the success rate of this program in his patient population is not reported (15,45). Rettig’s program includes restriction from throwing for two or more months, upper-extremity strengthening, and bracing. When the athlete is pain free, a throwing program is initiated and advanced over a period of 1 to 2 months (44).
Surgical treatment for UCL tears has evolved over the past few decades. Primary repair of the UCL when acutely injured had been advocated for years (17,34,46). Most UCL avulsion injuries were treated by surgically reattaching the ligament to bone using drill holes, whereas midsubstance tears were repaired with sutures primarily (46). Conway et al. (15) published their data of 70 athletes with acute UCL disruptions noting that 87% of UCL injuries were midsubstance, 10% were avulsions from the ulna, and only 3% were humeral avulsions. Jobe’s (15,45,47) recommendation was to perform UCL reconstruction with a free tendon graft in the acute setting with the main exception being an individual undergoing surgery soon after a proximal UCL tear, with no ulnar nerve symptoms, and with the remainder of the ligament appearing normal. Currently, the most popular surgical recommendation is to perform UCL reconstruction for both acute and chronic injuries (21). Transposition of the ulnar nerve was recommended in all cases; however, there is a significant incidence of neurologic symptoms postoperatively (up to 21%), which has led to a more conservative approach (15). The techniques currently employed by most surgeons do not involve transposition of the ulnar nerve unless there is significant ulnar nerve involvement including motor weakness or significant sensory changes.
Current popular indications for UCL reconstruction are for (a) acute ruptures in overhead sports athletes, (b) significant chronic elbow instability; (c) after debridement for calcific tendinitis, if there is insufficient tissue to effect a primary repair in an overhead or throwing athlete; and (d) multiple episodes of recurring elbow pain (with subtle instability) with throwing after periods of conservative treatment.
Current results appear better than after initial reports of UCL reconstruction. Conway et al. (15) reported the results of 56 UCL reconstructions using a palmaris longus free tendon graft and found 80% good-to-excellent results at 2 to 5 years, with 68% of the athletes returning to their previous level of competition for after more than 1 year. In that series, the authors transposed the ulnar nerve in all cases and found that 21% had postoperative ulnar neurologic symptoms, 40% were transient (all but one patient returned to previous sports activity), but 60% required a second operative procedure for the nerve (with almost half returning to previous sports activity) (15). These results have been confirmed by others (31). Jobe noted that UCL reconstruction results were not as good when there had been a previous operative procedure performed on the same elbow. In the last decade, there have been revisions in surgical technique for UCL reconstruction to make the surgery easier, more reproducible, and to reduce the incidence and risk of complications including not transposing or addressing ulnar nerve and splitting the FCU muscle mass (48), elevating flexor-pronator tendon without detaching it (31), these latter two eliminating the need to detach and reattach the flexor pronator mass with the risk of weakness and delayed avulsion of the musculotendinous unit. Other variations including fixation and tunnel techniques such as the docking procedure (49) and interference screw fixation (50) to reduce the need for multiple drill holes to reduce the risk of blow out of the bony bridge and making it easier to tension the graft. There has been a report of fixation of the graft with suture anchors onto bone as compared with tunnels (51); however, this biomechanical study is lacking clinical follow up and other studies to confirm the efficacy. Schwab et al. (52) had recommended transfer of the anterior oblique ligament anteriorly and superiorly with its bony attachment when the UCL is present but attenuated. However, this treatment is generally not recommended because the attenuated ligament is weaker due to microscopic injury, its position is not functionally isometric compared to its natural position, and elbow extension tends to be lost when using this technique, all of which is likely not acceptable to most individuals when there is a more anatomic reconstruction technique available without those risks. Synthetic ligament research has continued over the years; however, no long-term clinical data exists showing efficacy of this approach.
Repetitive valgus stress and microtrauma to the elbow may result in chronic UCL injury that occasionally heals with calcification. These calcifications are generally removed during the UCL reconstruction. It is important to note, however, that these calcifications are within the UCL. Individuals with medial elbow pain and these calcifications must be carefully examined. Care must be taken to not mistake these intraligamentous calcifications for intra-articular loose bodies. These calcifications are extra-articular, and
removal of them without UCL reconstruction will result in an unstable elbow.
removal of them without UCL reconstruction will result in an unstable elbow.

Though there were case reports years prior, posterolateral rotatory instability (PLRI) was brought to the forefront in the early 1990s by O’Driscoll (53,54). This elbow injury pattern is a rotatory instability due to injury to the lateral UCL. The mechanism of injury is a combination of axial compression, valgus stress, and supination (or external rotation) that imparts a rotational force to the elbow resulting in a spectrum of soft tissue injury (53). The initial injury is to the ulnar portion of the LCLC and progresses to capsular disruption (anterior and posterior capsule) and eventually may involve the UCL complex if the injury is severe (53,54,55,56).
Understanding the pathomechanics is the key to understanding PLRI. PLRI of the elbow results from a rotatory
subluxation of the radius and ulna (which move together as a unit) relative to the distal humerus. Because the annular ligament is intact in this injury, the radio-ulnar joint maintains its normal relationship and thus the proximal radius and ulna move together in a “coupled” motion. Most give credit to Osborne and Cotterill (57) as the ones who probably were the first investigators to appreciate fully the importance of the lateral ligamentous complex in recurrent instability and describe the findings of PLRI. While much of the initial literature by O’Driscoll (53,54) and the laboratory at the Mayo Clinic reported the results of biomechanical studies demonstrating that the primary stabilizer to PLRI is the lateral ulnar collateral ligament (LUCL) (53,54,55,56,58), much of the recent literature suggests that the injury may not be to the LUCL alone. It was reported that the radial collateral ligament and capsule serve as secondary restraints. However, clinically it is known that elbow dislocations and injury to the LCLC occurs proximally. And it is after this injury that the PLRI most commonly manifests. Subsequent research by other investigators has suggested that isolated injury to the LUCL alone may not be enough to produce PLRI (18,59,60). Some suggest that the complex works in a “Y” configuration and that a proximal injury, near the epicondyle, as seen clinically, can and does produce PLRI (61).
subluxation of the radius and ulna (which move together as a unit) relative to the distal humerus. Because the annular ligament is intact in this injury, the radio-ulnar joint maintains its normal relationship and thus the proximal radius and ulna move together in a “coupled” motion. Most give credit to Osborne and Cotterill (57) as the ones who probably were the first investigators to appreciate fully the importance of the lateral ligamentous complex in recurrent instability and describe the findings of PLRI. While much of the initial literature by O’Driscoll (53,54) and the laboratory at the Mayo Clinic reported the results of biomechanical studies demonstrating that the primary stabilizer to PLRI is the lateral ulnar collateral ligament (LUCL) (53,54,55,56,58), much of the recent literature suggests that the injury may not be to the LUCL alone. It was reported that the radial collateral ligament and capsule serve as secondary restraints. However, clinically it is known that elbow dislocations and injury to the LCLC occurs proximally. And it is after this injury that the PLRI most commonly manifests. Subsequent research by other investigators has suggested that isolated injury to the LUCL alone may not be enough to produce PLRI (18,59,60). Some suggest that the complex works in a “Y” configuration and that a proximal injury, near the epicondyle, as seen clinically, can and does produce PLRI (61).
The diagnosis of PLRI is much more elusive than other ligament injuries, including the UCL, and requires a careful history and physical examination. The physician must be diligent and maintain a high degree of suspicion in patients with vague reports of elbow discomfort. It is frequently reported in patients with PLRI that they have painful clicking, snapping, clunking, locking, dislocating, or giving way. Position of the hand during maneuvers that elicit these symptoms is critical, because the symptoms are often produced when the patient extends his or her elbow, with the forearm supinated (53). An example would be using their hands to get out of a chair with their forearms maximally supinated.
Standard, preliminary physical examination of the elbow generally is unremarkable with regard to strength, range of motion, and tenderness. The diagnosis requires a provocative test of stability described by O’Driscoll et al. (53) and termed the lateral pivot-shift test of the elbow (Figs 23-19 and 23-20A). The pivot shift test is performed with the patient supine and the examiner standing above the patient’s head. The extremity being tested is brought above the patient’s head into forward elevation, and the shoulder is placed in full external rotation to stabilize the humerus for the test. The patient’s forearm is held in maximal supination, with one hand grasping the wrist distally. A valgus force is applied with the examiner’s other hand as the elbow is slowly flexed from a starting position of full extension. The combination of supination and valgus results in an axial joint compression, which produces a posterolateral rotatory subluxation of the combined radius and ulna relative to the humerus. In the unanesthetized patient, this maneuver reproduces the sensation of instability and often elicits apprehension or guarding. Usually, the subluxation is maximized as the elbow reaches 40 degrees flexion and further flexion results in a sudden clunk as a result of joint reduction. Dimpling of the skin proximal to the radial head (posterolateral elbow) can be seen with PLRI in degrees of extension before the reduction (Fig 23-20B). The clunk of reduction in PLRI is generally only felt by the examiner in a patient under general anesthesia or occasionally following an intra-articular injection of anesthetic. If a patient does display apprehension or guarding during the pivot-shift maneuver in the office, then we usually recommend confirmation of posterolateral instability with an examination under anesthesia (Fig 23-20A). Lateral stress radiographic studies or fluoroscopy during the pivot maneuver demonstrates posterolateral radial head dislocation with widening of the ulnohumeral joint (due to the subluxation of the ulna out of the trochlear groove) and may be helpful for confirmation and documentation of the pathomechanics.
Plain radiographs are usually unremarkable unless there is a bony avulsion off the lateral epicondyle. Stress radiographs can often show the posterolateral subluxation if the patient is relaxed and allows application of the forces necessary. Lateral stress radiographs show that the ulna is rotated off the distal humerus (slight gapping of the ulnohumeral joint with otherwise maintained relationship) while the radial head is rotated with the ulna so that the radial head rests posterior to the capitellum (Fig 23-21). The normal radio-ulnar relationship differentiates PLRI from radial head subluxation or dislocation in which the radial head is displaced but the ulnohumeral joint is entirely normal. While arthrography has not been shown to be of benefit in aiding the diagnosis, advances in MRI have allowed good visualization of the LCLC and can be helpful in confirming the diagnosis in some cases. However, since this is a dynamic problem, and the ligament may be stretched but intact, the sensitivity and specificity of MRIs for PLRI is less than one would like; however, studies are still forthcoming.
No nonoperative treatment regimens have been reported to be successful in the management of PLRI (62). For PLRI that markedly interferes with the patient’s daily function, one can consider activity modification and a hinged brace with forearm pronation and an extension block, although few patients are content with this option and most require surgical intervention.
Surgical treatment of PLRI involves reattaching the avulsed LCLC or reconstructing the LUCL with a free tendon graft to the lateral epicondyle, similar to reconstruction of the medial UCL. Because the annular ligament is intact in these patients, radio-ulnar dislocation does not occur, and surgery on this ligament is not indicated (62). If the condition is diagnosed acutely, primary repair of the lateral UCL may be performed, and this is usually an avulsion from the humerus and can be repaired back to the bone (63) (Fig 23-22). Modifications of the technique originally described by O’Driscoll include making the distal connecting tunnels perpendicular to the isometric point to the lateral epicondyle as well as using the interference screw technique. Results for these latter techniques are lacking, but in general, surgical reconstruction of the LUCL has been excellent (64). Osborne and Cotterill (57) reported excellent results in eight patients with transosseous repair and Nestor et al. (64) reported excellent results in three patients. Ligamentous reconstruction results have been mixed. O’Driscoll and Morrey reported a 90% success rate in restoring stability in the absence of arthritis or radial head fracture. 60% resulted in excellent results while 40% continued to have pain or some loss of motion.

The elbow of the skeletally immature athlete is susceptible to two main problems: apophyseal injury and osteochondritis dissecans (OCD). The physis is the weakest link in the skeletally immature musculoskeletal system, resulting in apophysitis and avulsion injuries, rather than ligament injuries as seen in adults. OCD is also common in young athletes, particularly throwing athletes. The term “Little Leaguer’s Elbow” has been applied to both medial- and lateral-sided injuries
(generally medial epicondylar apophyseal injury and OCD of the capitellum) and thus has very little usefulness and is avoided in this chapter.
(generally medial epicondylar apophyseal injury and OCD of the capitellum) and thus has very little usefulness and is avoided in this chapter.
Medial Epicondylar Physeal Injury
Fracture or avulsion of the medial epicondylar physis can be a stress fracture or overt fracture due to repeated valgus forces, falling, or repetitive violent forearm flexor muscle contraction in the skeletally immature athlete. Throwing results in a repetitive valgus stress and can result in accelerated growth of the medial epicondylar apophysis, which can be followed by separation and eventual fragmentation if the body does not have the opportunity to recover and heal from the repetitive forces (65,66) (Fig 23-23). Pediatric athletes with medial epicondylar apophysitis complain of pain with throwing while also noting their throwing accuracy, speed, and distance are reduced. On examination, the patient has point tenderness over the medial epicondyle. Frequently, these pediatric athletes have an elbow flexion contracture of more than 15 degrees based on muscular and capsular tightness. Radiographs demonstrate that the medial epicondylar apophysis can be widened and occasionally fragmented. This is as compared with the contralateral elbow, which should always be obtained when evaluating an injured elbow in the skeletally immature patient with elbow pain due to the complexity and various ages of closure of the multiple different physes about the elbow (Fig 23-24). Care should be taken, since this finding may be normal in throwing athletes (34). The treatment of the stress fracture or inflamed apophysis is rest from throwing for 4 to 6 weeks, along with the
application of ice (take care to protect the ulnar nerve from ice injury by placing a wet towel between the ice and the medial elbow). NSAIDs may also be used to reduce pain and the acute inflammation. After the symptoms subside, a rehabilitation program is instituted consisting of stretching to eliminate the flexion contracture, strengthening of the muscles that cross the elbow, and then transitioning to a gradual throwing program.
application of ice (take care to protect the ulnar nerve from ice injury by placing a wet towel between the ice and the medial elbow). NSAIDs may also be used to reduce pain and the acute inflammation. After the symptoms subside, a rehabilitation program is instituted consisting of stretching to eliminate the flexion contracture, strengthening of the muscles that cross the elbow, and then transitioning to a gradual throwing program.
![]() Fig 23-23. Medial epicondylar apophysitis and avulsion occur due to repeated stress from the ulnar collateral ligament (arrow) and flexor-pronator muscle forces. |
![]() Fig 23-24. Thirteen-year-old baseball pitcher with medial elbow pain. A: Left (normal) elbow. B: Right, throwing elbow showing widening of the medial epicondyle physis. |
Continued stress to the elbow with apophysitis may result in progression to a complete fracture or separation of the medial epicondyle. The avulsion fracture of the medial epicondyle, however, may occur without any preceding symptoms of medial elbow pain and is usually due to a single, more severe, valgus force or violent muscle contraction. Medial epicondylar apophyseal fracture can have two distinct patterns, as described by Woods (67) and Woods et al. (68). A Woods Type 1 fracture occurs in the younger child and is characterized radiographically by a large fragment, involving the entire epicondyle that is often displaced and rotated anteriorly and distally, and may even become entrapped in the joint. A Woods Type 2 fracture occurs in the adolescent and is radiographically notable for medial epicondylar fragmentation with a small fracture fragment. In this type of injury, the flexor-pronator muscle is essentially avulsed; however, the anterior oblique ligament of the UCL complex is usually intact. Treatment of this entity, however, depends on elbow stability (Fig 23-18).
The treatment of these injuries where the elbow is stable and epicondyle is nondisplaced is rest for 2 to 3 weeks, with return to throwing by 6 weeks after attaining full motion. Unstable elbows are treated by open reduction and internal fixation, using wires, pins, or screws. If, however, the medial epicondyle is displaced more than 1 cm, then surgery should be performed to anatomically reduce the fragment and internally fix it with pins or screw(s) (69,70) (Fig 23-25). It should be noted that displacement of 1 cm as an indication for surgery is somewhat arbitrary, and it is easy to see how malunion of this fracture could lead to functional medial ligamentous laxity, which would be expected to be symptomatic in a throwing athlete. Therefore, we recommend aggressive management, including open reduction and internal fixation, to obtain adequate fracture reduction. In nonthrowing pediatric athletes or for the nondominant elbow, some authors recommend surgery only if the fragment is displaced 2 cm or more.
![]() Fig 23-25. Medial epicondylar avulsion fracture. A: Displaced medial epicondyle fracture. B: Fracture fixed with internal fixation. |
Occasionally, elbow dislocation is complicated by avulsion of the medial epicondyle fragment, which may become entrapped within the elbow joint. Closed manipulation of the elbow by applying valgus stress and wrist extension can free the fragment from within the joint, enabling the child to avoid open surgery. As an adjunct, air can be injected into the lateral joint, which may help to move the incarcerated fragment out of the joint, helping to avoid open reduction (69,71). Minimally displaced medial epicondyle avulsion injuries and apophysitis (widening of the growth plate without fracture or displacement) can usually be managed without surgery. If surgery is required to manage this problem, UCL evaluation is also performed and the ligament is repaired as needed.
Olecranon Apophysitis
Abnormal stresses of the triceps attachment on the olecranon apophysis can result in an injury syndrome similar to Osgood-Schlatter disease of the knee. An intrinsic overload from repeated triceps pull can lead to inflammation and separation of the olecranon secondary ossification center (72). This injury may also be due to valgus extension overload and has been reported in a baseball pitcher (73,74). Generally, those afflicted are adolescents. Typical complaints are those of pain on resisted elbow extension and tenderness over the olecranon. Radiographs demonstrate widening of the olecranon apophysis, and should be confirmed by comparison with radiographs of the contralateral elbow.
When the apophysis is widened, but not separated or avulsed, initial treatment is rest from the offending activity, gentle range-of-motion and flexibility exercises, eventually progressing to strengthening exercises. Ice and NSAIDs are useful if acute inflammation is present. If the child is in severe pain, a short period of immobilization in a splint or brace may be beneficial. In the situation where there is separation of the secondary growth center or adolescents have persistent pain despite conservative management due to lack of fusion of the physis, internal fixation is recommended. Surgical intervention to promote fusion of the apophysis may require internal fixation with a screw in addition to bone grafting, due to the high incidence of fibrous union when bone grafting is not used (75,76). Left untreated, apophysitis of the olecranon may result in an incompletely fused olecranon apophysis, which may later fracture as a result of direct trauma when older (73,74,75,77,78). It is generally recommended that unfused olecranon physis fractures in adults be treated with open reduction, internal fixation, and primary bone grafting, though recent case series have been presented calling into question the need for bone grafting (75).
Osteochondritis Dissecans
OCD is a focal lesion of the lateral elbow that occurs most frequently in young throwers and gymnasts. Although OCD can occur in throwers and nonthrowers, in the dominant and nondominant elbows, and in the capitellum, radial head, or both, it tends to occur in the capitellum of the dominant arm in throwers and gymnasts 10 to 15 years of age (79,80,81,82,83,84,85). The exact etiology of OCD remains a mystery. A current leading hypothesis of the etiology of OCD is that it is a result of vascular insufficiency due to repetitive trauma or microfracture in a genetically predisposed adolescent. The capitellar blood supply is tenuous, with end-arterioles terminating at the subchondral plate (86). The current leading pathogenesis theory proposes that compression at the radiocapitellar joint produces arterial injury at the subchondral plate resulting in bone death (34). As noted in the section on throwing injuries, the radiocapitellar joint functions as a secondary restraint to the valgus stresses associated with throwing resulting in compression of this joint (Figs 23-26 and 23-27). OCD can be a disabling problem.
![]() Fig 23-27. Schematic representation of radiocapitellar compression associated with valgus force and ulnar collateral ligament laxity thought to result in OCD of the capitellum or radial head. |
There is a debate as to whether OCD is a distinctly different entity from Panner disease; however, it should be clear that these diagnoses have different prognoses and treatments. The difference between the two problems focuses on age and degree of involvement of the capitellar secondary ossification center. Panner disease occurs in the young child, usually 7 to 12 years of age, and is characterized by a focal, localized lesion of the subchondral bone and its overlying articular cartilage (anterior central capitellum) (86). In comparison, OCD occurs in the older child, 13 to 16 years of age, and is characterized as a focal lesion in the capitellum demarcated by a rarefied zone, with or without loose bodies (34).
The skeletally immature athlete often gives a history of insidious onset of lateral elbow pain and reduced throwing effectiveness and distance. The child may have a flexion contracture of more than 15 degrees on examination. A provocative test, which may reproduce symptoms and suggest the diagnosis, known as the “active radiocapitellar compression test,” consists of forearm pronation and supination with the elbow in full extension resulting in pain if OCD is present. Early in the process, radiographs may be normal, although with time, islands of subchondral bone demarcated by a rarified zone can be seen, and with even more time, loose bodies may be generated (Fig 23-28). MRI, computed tomography (CT), and ultrasound have all been found to be helpful in defining the extent of the lesion. The critical factor in determining treatment is the status of the overlying cartilage. The diagnosis is usually made by MRI, MR arthrogram, arthro-CT scan, ultrasound, or arthroscopy if plain films do not show free fragments to help detect early OCD lesions and to assess the integrity of the overlying articular cartilage (87). If the overlying articular cartilage is intact, treatment is relative rest and occasionally splinting, followed by range-of-motion and then strengthening exercises. Some have also advocated an unloading type of elbow brace. Symptoms generally subside with rest alone; however, throwing is contraindicated because the healing process may lag behind symptomology and tends to be slow. The athlete is restricted from throwing and other heavy activities until there is radiographic evidence of healing. Bennett and Tullos (34) have suggested that fragmentation is likely if pain and flexion contracture persist for more than 6 weeks. If the overlying articular cartilage is not intact at the time of surgery, some recommended excising the fragment regardless of size. They note the patient will have a functional elbow; however, the patient should be discouraged from throwing or performing heavy labor. The current recommended management for elbows where the articular cartilage is not intact is to reattach and internally fix large fragments with screws, wires, or biodegradable pins after drilling the bed to enhance vascularity, similar to the procedure described by Indelicato et al. (88). Smaller fragments, on the other hand, are usually just removed. The surgeon usually attempts to fix the larger fragments to help reduce articular surface incongruity and potentially degenerative changes, while smaller fragments, if removed, would have less impact on contact stresses.
If the large fragment has been chronically detached, the fragments may have continued to grow, and/or become incarcerated within the joint, resulting in multiple smaller fragments or in a fragment that is rounded and hypertrophied, and in either scenario, reattachment may not be possible. In these situations, the bony fragment(s) is removed and the crater is cleared of the fibrous tissue and roughened to attempt to stimulate fibrocartilaginous growth. Yamamoto has also recommended filling the defect, particularly if it involves the lateral columnar wall, with an autogenous osteochondral graft from the knee (88a). There have been several reports of excellent short-term results of arthroscopic debridement of loose articular cartilage flaps and loose bodies removal for OCD (89,99). However, studies have also shown that the late sequelae of OCD after removal of loose bodies include deformity of the capitellum and radial head, arthritis, loss of motion, pain, and often residual disability (91,92).
Radial Neck Physeal Fracture
Fracture of the radial neck at the physis may be the result of a fall on an outstretched hand or due to valgus stresses at the elbow with attenuation or insufficiency of the UCL resulting in increased compressive forces to the radiocapitellar joint. This injury tends to be acute due to a sudden force to the radiocapitellar joint in the skeletally immature athlete. These children complain of lateral elbow pain and reduced forearm rotation. On examination, these kids have tenderness over the radial head and concomitant loss of supination and pronation. Radiographs reveal a fat pad sign is present, and the fracture may be identified if displaced or with stress films. The treatment for nondisplaced fractures is rest for 4 to 6 weeks, until the fracture is healed. Displaced fractures
are treated with closed versus open reduction, which is based on the degree of angular displacement.
are treated with closed versus open reduction, which is based on the degree of angular displacement.

The ulnar nerve is the most commonly injured around the elbow. This is likely due to the tight path it follows, which changes its dimensions with elbow flexion and extension, its subcutaneous location, and the considerable excursion required of it to accommodate the full motion of not only the elbow but also the shoulder. The ulnar nerve may be injured by traction, direct trauma, friction, or compression. Compression, either alone or in combination with the other causes, can produce cubital tunnel syndrome. Interneuron pressure increases six times over resting levels when the elbow is flexed, wrist extended, and shoulder abducted. With full elbow flexion the confines of the cubital tunnel become restrictive and the retinaculum becomes taut and compresses the nerve (Fig 23-29). Beginning proximally to distally, the most common sites of ulnar nerve compression are the arcade of Struthers, medial intramuscular septum, medial epicondyle, cubital tunnel, and deep flexor pronator aponeurosis. Other causes of ulnar nerve compression that are less common include cubitus valgus and cubitus varus, which are sometimes associated with snapping triceps syndrome, a subluxing medial head of the triceps.
Injury to the ulnar nerve can result in significant long-term morbidity. The relatively high incidence of ulnar neuropathy is likely related to its posteromedial location in the elbow with its path through a confined space and the necessity for generous excursion to accommodate motion of the elbow and shoulder (Fig 23-30). The ulnar nerve is a terminal branch of the medial cord of the brachial plexus. Through the upper two-thirds of the arm it travels along the anterior aspect of the medial intramuscular septum near the median nerve and brachial artery. At the distal third of the arm, the ulnar nerve pierces the intramuscular septum and passes under the anteromedial margin of the triceps to the medial epicondyle. There it passes through the cubital tunnel where the retinaculum joining the medial epicondyle to the olecranon encloses the tunnel configuration (Fig 23-31). The floor of the tunnel is the joint capsule and posterior band of the ulnar collateral ligament. Cubital tunnel retinaculum can display considerable anatomic variation. This is also known as the arcuate ligament. It may be muscular, known as the anconeus epitrochlearis, or fibrous and tight or alternately absent. Distal to the cubital tunnel, the nerve passes between the ulnar and humeral heads of the pronator teres. There are no clinically significant branches from the ulnar nerve to the upper arm. A sensory branch to the elbow joint is the first noted branch and usually arises proximal to the medial epicondyle. Occasionally, a branch to the FCU arises proximal to the cubital tunnel and courses in intimate relationship with the ulnar nerve itself in the cubital tunnel. Distally, in the cubital tunnel, the ulnar nerve distributes two to three branches to the FCU. The medial brachial cutaneous and antebrachial cutaneous nerves both pass through the posteromedial arm and medial epicondyle.
Pronator syndrome involves the median nerve at the level of the elbow and is painful. As a cause of median nerve compressive neuropathy, it is a distant second to carpal tunnel syndrome. The median nerve originates from branches from the medial and lateral cords that pass through the upper arm next to the brachial artery. It contains contributions from the sixth through the eighth cervical roots and the first thoracic nerve root of the brachial plexus.
The median nerve crosses the elbow joint medial to the brachial artery. It passes distally under the lacertus fibrosis and then penetrates the pronator teres. Considerable anatomic variation can be noted as the nerve traverses the pronator teres. Entrapment of the median nerve around the elbow may occur at various anatomic sites.
A supracondylar process when present and ligament of Struthers may cause compression of the median nerve just proximal to the elbow and is a possible cause of pronator syndrome. The causes are compressive. The possible compressive structures beginning proximally include the ligament of Struthers, lacertus fibrosis, fibrous band of the pronator teres, and the fibrous band in the flexor digitorum superficialis. Pronator syndrome presents as a dull ache and pain in the proximal anterior forearm just distal to the antecubital fossa and may radiate distally to the volar wrist. Rarely will it radiate proximally. Dysesthesias in the radial three and one-half digits and weakness and clumsiness may also be noted. Pronator syndrome usually occurs in the fifth decade of life and is four times more common in women than men. The history may involve a direct blow to the forearm, repetitive trauma, or, more commonly, no known cause. Physical findings include a positive Tinel sign over the nerve at the elbow, reproduction of symptoms with resisted pronation (or less
commonly with supination), a positive median nerve compression test at the elbow that reproduces the symptoms, or tenderness over the proximal edge of the pronator teres. Initial conservative treatment of the pronator syndrome is rest, modified activities, nonsteroidal anti-inflammatory medication, and splintering. The majority will respond to conservative management. In recalcitrant cases surgery may be indicated.
commonly with supination), a positive median nerve compression test at the elbow that reproduces the symptoms, or tenderness over the proximal edge of the pronator teres. Initial conservative treatment of the pronator syndrome is rest, modified activities, nonsteroidal anti-inflammatory medication, and splintering. The majority will respond to conservative management. In recalcitrant cases surgery may be indicated.
With anterior interosseous syndrome, a deep unremitting pain in the proximal forearm usually precedes the symptoms that define the syndrome. The patient may complain of loss of dexterity or weakness of pinch. Clinically, loss of the function of the flexor pollicis longus and flexor digitorum profundus to the index and middle fingers with weakness of the pronator quadratus is noted. No tenderness over the proximal forearm is usually noted and sensibility is unaffected. The thumb and index finger assume a classic position during pinch in anterior interosseous syndrome. The index finger extends at the distal interphalangeal joint with compensatory increased flexion of the proximal interphalangeal joint. The thumb hyperextends at the interphalangeal joint and demonstrates increased flexion at the metacarpophalangeal joint. Conservative measures for treating anterior interosseous nerve syndrome include rest, activity modification, protective immobilization, holding the elbow in flexion, forearm in slight pronation and wrist in slight flexion, cryotherapy, nonsteroidal anti-inflammatory medication later followed by physical therapy and rehabilitation.

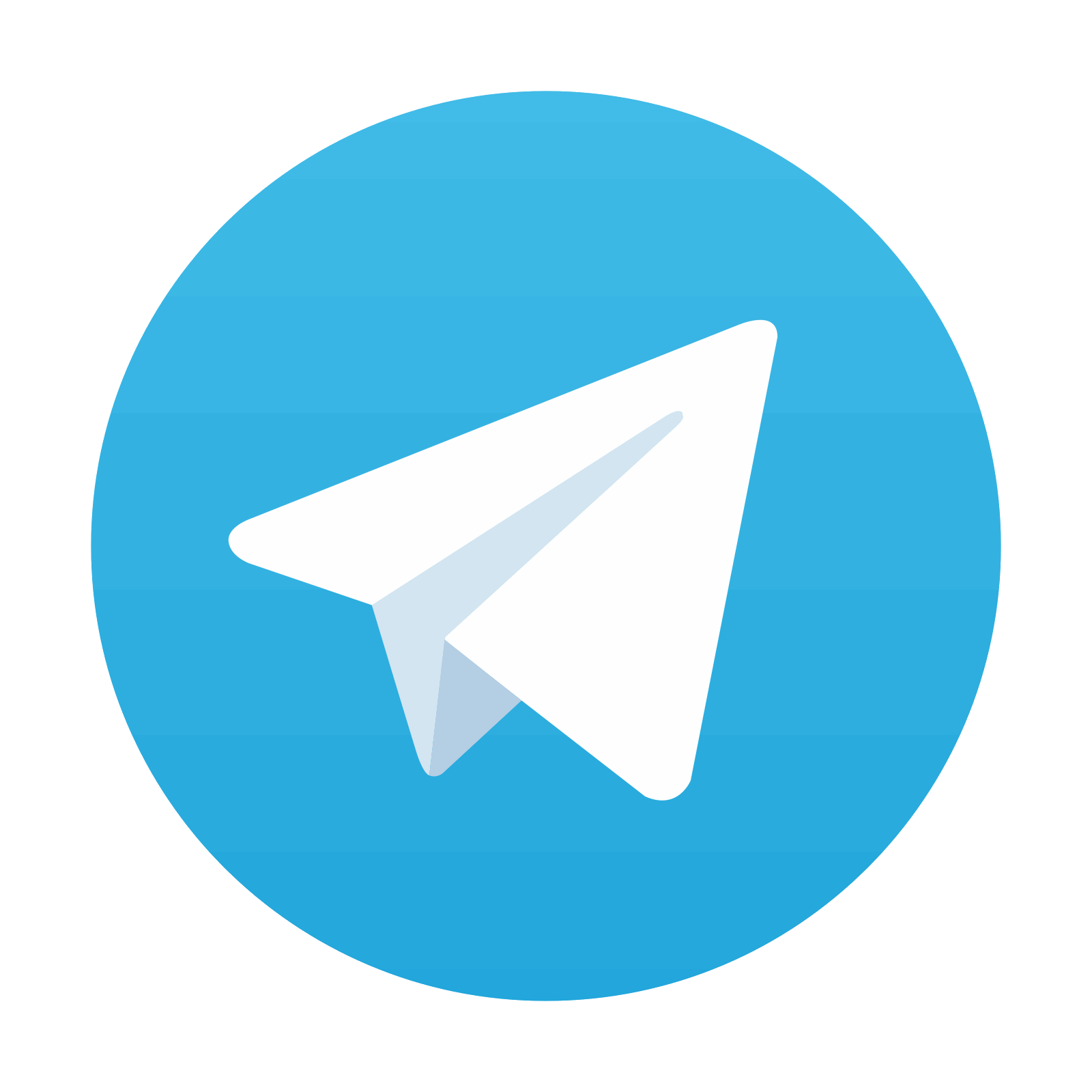
Stay updated, free articles. Join our Telegram channel

Full access? Get Clinical Tree
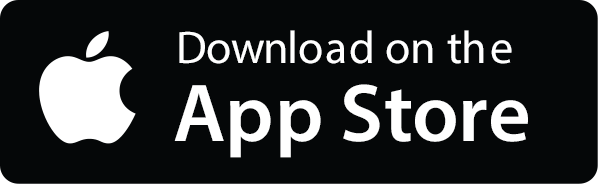
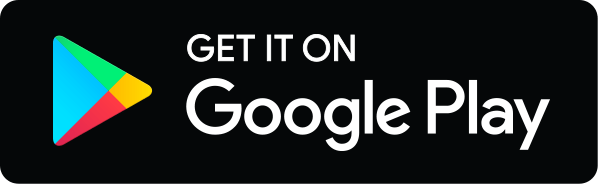