9 Infancy is a unique period of neural development and plasticity Interaction of brain and muscle injury and plasticity Muscle plasticity in infants with cerebral palsy Efficacy and effectiveness of early intervention: study design considerations Types and amounts of interventions that have been delivered The future of physiotherapy for infants with CP Brain and muscle plasticity in response to brain injury and specific types of physiotherapy have been demonstrated in cerebral palsy (CP) (Cope et al., 2010; McNee et al., 2009). Despite this exciting evidence, physiotherapists who provide early intervention services for infants with cerebral palsy and their families face a quandary. After several decades and multiple studies, the consensus among systematic reviews on physiotherapy for infants with or at high risk for cerebral palsy is that early intervention aiming to improve motor outcomes regardless of type has not been shown to produce positive mean group changes that are more than what would be expected due to maturation (Hadders-Algra, 2011). Comparisons with control groups receiving no or another intervention have similar results of no group differences in motor development. Some of the interventions appear to improve cognitive outcomes in infants (Blauw-Hospers et al., 2007; Orton et al., 2009), facilitate bonding (Kaaresen et al., 2008) or reduce parental stress (Badr et al., 2006), which are clearly important. However, these positive changes provide an interesting contrast to the consistent absence of statistically and/or clinically significant improvements in motor development. The consensus from all of these studies is perhaps even more baffling today as evidence is rapidly accumulating on the remarkable potential for the brain to recover from injury that is purported to be even greater in a more immature nervous system (Villablanca and Hovda, 2000). In fact, it seems almost inconceivable that early intervention strategies not only show no appreciable benefit on motor functioning but also fail to show a dramatic effect. Newer innovative treatment strategies have emerged that are based on sound developmental principles such as coping and caring for children with special needs (COPCA) (Blauw-Hospers et al., 2007) or context-based approaches (Law et al., 2011), yet evidence of superiority still remains elusive for these, as shown by results from well-controlled randomized controlled trials (RCTs) (Hielkema et al., 2011; Law et al., 2011). How can we forge a path forward based on these disappointing results that fly in the face of what we know are tremendous possibilities for brain plasticity and recovery of function? This chapter will explore possible explanations for this apparent ‘disconnect’, focusing primarily on the amount, type and timing of interventions that have been provided to infants and families and on study design issues and challenges. Intervention features will be evaluated in light of the current evidence that supports the fundamental role of motor activity in promoting brain and muscle development and plasticity throughout the lifespan. Central to these discussions are current assumptions and knowledge on the uniqueness of the infant with regard to these processes and the associated implications for therapeutic strategies. Although developmental changes occur in the brain throughout the lifespan, the processes that occur in infancy are dramatic and unique in many ways. During infancy there is an overabundance of neural pathways that are established and then pruned through programmed cell death (apoptosis) as well as experience-dependent enhancement or attenuation of synaptic connections within and across brain regions (Johnston, 2009). A brain injury disrupts normal developmental processes in ways that we are only recently beginning to understand. We now know that the brains of children with CP reorganize substantially in response to injury, as seen in infants with hemiplegia who may retain a higher percentage of bilateral corticospinal projections or whose brain may reorganize so that the dominant hemisphere provides ipsilateral input to the non-dominant extremity (Staudt, 2010). While these processes may restore some degree of functionality to the affected extremity, this is accomplished by the formation or strengthening of pathways between remaining brain regions, rather than by direct repair of the damaged areas. Substitution of function may also occur that could be at the expense of recovery or development in another skill or domain. These imperfect repair processes may also impose distinct limits on rehabilitation potential, at least given the current state of medical care and science. In children with congenital hemiplegia, the pattern of reorganization is intricately related to dexterity of the paretic hand, with those who demonstrate shared representation of the paretic and non-paretic hand in the intact hemisphere having the worst prognosis (Vandermeeren et al., 2009). In some cases where the damage occurs prior to or in a critical period of the development of a specific functional capability, that function may never develop (Rittenhouse et al., 1999). It has been well-argued that ‘developing brain’ is not a term that should be reserved just for the first few years of life (Kolb and Gibb, 2011). The brain is always developing, with no age limit on plasticity (Thickbroom and Mastaglia, 2009), but has different capabilities and vulnerabilities that depend on its current stage of development and thus may have divergent responses to the same insult at different time periods. An example is the differential effect of a traumatic brain injury in early versus late adolescence, in that motor outcomes are typically worse in the earlier years but frontal lobe outcomes are better compared with later adolescence where the pattern is often reversed (Kolb and Gibb, 2011). Anderson et al. (2011) describe a ‘recovery continuum’ for infants with early brain insults, with the opposing processes of heightened vulnerability versus plasticity in the immature brain at the two extremes of the outcome spectrum representing poor or excellent outcomes, respectively. They state that where a given child falls on that continuum depends on injury factors, e.g., the location, extent and timing of injury and environmental factors, e.g., parenting, socio-economic status and interventions; each of these factors may act independently or synergistically. Even though cerebral palsy is not a genetically transmitted disorder (although several genetic disorders have been uncovered that had been errantly diagnosed as CP), individual genetic factors may be largely responsible for modulating responses to environmental stress, injury and recovery and further contribute to variability in prognosis and response to treatment. A fascinating study on adaptive plasticity of the motor cortex in 16 pairs of identical monozygotic vs dizygotic twins demonstrated a strong ‘heritability estimate’, concluding that ‘genetic factors may contribute significantly to inter-individual variability in plasticity paradigms’, which may have implications for motor learning and rehabilitation potential (Missitzi et al., 2011). It has also been shown that maladaptive plastic changes are ‘plastic’ themselves, which provides even greater optimism for promoting and refining brain repair and reorganization over a more prolonged time period (Martin, 2012). Injury to the brain leads to alterations at all levels of the central nervous system as well as the peripheral nervous system with the converse also being true. For example, diffuse muscle atrophy can occur in the affected extremities after a middle cerebral artery infarct, or there can be a dramatic loss of cortical representation of an amputated limb. Movement is produced through the interplay of the central and peripheral nervous systems and therefore can be impaired by a disruption at any level with widespread secondary effects. In cerebral palsy the initial injury is, of course, in the central nervous system, which leads to deficits in motor function in specific body parts depending on the location and extent of the injury, often with a predictable bias towards preservation of some movements or patterns of movement over others. Further confounding the picture, the amount of voluntary motor activity in an affected body part is often less than that in an unaffected body part, which then leads to a competitive disadvantage for that body part at the joint level, the level of the neuromuscular junction and at the level of synapses in the brain. Muscle atrophy and increased intrinsic stiffness, joint contractures and bony deformities may all develop as a result of these movement biases. Current treatments in CP such as strength training, botulinum toxin injections or orthopaedic surgery have some measurable effects in alleviating some of the secondary musculoskeletal consequences in the short term, but since the brain injury is still present, the abnormal influences persist and continue to exert effects peripherally. These, in turn, may have further central effects, an iterative process that causes a ‘static’ injury to worsen over time, with additional confounding negative influences from other processes such as physical growth and ageing. Until recently, trying to manage the secondary peripheral effects was deemed to be the most, if not the only, effective management strategy. This approach has at best been marginally successful in maintaining or positively influencing the level of functional mobility for individuals with CP and has largely failed to successfully prevent the precipitous decline in function in older individuals (Ando and Ueda, 2000; Bottos and Gericke, 2003). Due to recent advances in neuroscience, our therapeutic approaches are beginning to be directed at the primary site of injury. The realization of the vast capacity of the brain to remodel and to some extent recover has dramatically shifted our focus on trying to better understand and therapeutically manipulate this process. The goal of what we need to try to accomplish was beautifully stated by Martin (2012) and highlights the formidable challenge ahead: ‘We must repair the damaged motor systems, so the brain reconnects with motor neurons. These connections must be sufficiently strong and precise to enable robust and proper voluntary muscle recruitment.’ This underscores why it is so important that muscles are sufficiently developed and structurally intact to both help drive and respond to these central nervous system changes. Muscles are now known to be one of the most malleable (‘plastic’) tissues in the body and respond very dramatically to increases or decreases in mechanical loading and activation throughout their lifespan (Narici and Maganaris, 2007). Muscle fibres respond to external stimuli or lack thereof by either a change in size or protein isoform content. Infants are believed to have nearly all of their adult muscle fibres present at birth, but have little antigravity muscle control, unlike many animals who walk independently shortly after birth. Dynamic systems theory, espoused by Thelen and Ulrich (1991), challenged the primacy of the neuromaturational explanation of motor development and explored biomechanical factors underlying this process. They postulated that the rate-limiting steps for the emergence of independent walking in infants were the development of postural control and adequate extensor muscle strength. Compelling indirect evidence for the critical importance of strength development in infancy can be seen through cross-cultural differences in infant handling and positioning practices. Clearly, those cultural practices that stimulate more antigravity movements or weight-bearing activities lead to systematically earlier skill acquisition. An example of how infant handling can influence motor development is the recent change in the USA in the recommended safe sleeping position for infants from prone to supine. This has had an appreciable adverse effect on the development of extensor strength and related milestone achievement, leading to public service announcements to encourage more ‘tummy time’ during play activities to ensure that children have sufficient opportunities to acquire adequate strength. Synapse formation at the neuromuscular junction in infancy mirrors the process that is occurring in the brain. In older children and adults, each muscle fibre is innervated by a single alpha motor neuron. However, during development many of the fibres are innervated by multiple axons. As in the brain, synapse elimination is an activity-dependent event with competition across axons (Lieber, 2010). Scientific data on the development of muscle strength and fibre type differentiation in infants is surprisingly lacking and is mainly inferred from invasive studies in young and older animals and in adult humans. Schiaffino et al. (2007) demonstrated remarkable plasticity in terms of fibre size (activity-dependent increases or decreases) and fibre type transformation in mature animal muscles from slow to fast isoforms in response to denervation and electrical stimulation. They further demonstrated the far greater capacity of immature versus mature muscle to remodel in response to external stimuli. There appears to be a set amount and intensity of physical activity that maintains muscle mass within a range that is commensurate with daily activity requirements. If activity levels drop or increase substantially, muscle adaptations are evident. The optimal amount of activity to maintain muscle integrity is difficult to estimate and in our increasingly sedentary society, ‘average’ activity amounts may be too low of a standard (Booth and Laye, 2010). Movement is ubiquitous and pervasive during fetal and child development and has been shown to be reduced in amount and amplitude in infants with motor disabilities as compared to those with typical development (Bell et al., 2010; Spittle et al., 2008; Vaal et al., 2000). How to provide them sufficient opportunities for self-initiated movement when movement production is the major deficit is a major challenge for physiotherapists and families. Intense and repetitive activation of muscles not only stimulates their own growth but also stimulates the release of neural and other growth factors that can enhance cognitive functioning and promote physical growth (Bell et al., 2010). These findings have major implications for children with cerebral palsy who typically move less and often have prolonged delays or difficulties in the acquisition of upright motor skills. Many children with CP also have restricted growth that correlates with their degree of involvement, which could reasonably be assumed to be mediated in part by their restricted muscle activity, although that has not been tested empirically. Alterations in spastic muscle extend far beyond changes in size and likely originate in infancy, although no longitudinal investigation of muscle changes during development in CP has been reported. Reported differences in CP include counter-intuitively longer sarcomere resting length in contracted muscles, marked disruptions in the extracellular matrix with excessive collagen content, and fat infiltration in muscle tissue, among others (Barrett and Lichtwark, 2010; Grant-Beuttler et al., 2000; Johnson et al., 2009; Lieber et al., 2004; Marbini et al., 2002; Theroux et al., 2005). The extent to which any of these structural changes can be modified is unknown, although recent evidence on changes in muscle volume after strength training has been reported (McNee et al., 2009). Activity enhancement should be the primary goal of early motor intervention for both the brain and the muscles, but how to best accomplish this in each child, when the best time to do this is and how much should be done are major unanswered questions (Damiano, 2006). Critical stages in brain and muscle development and the points at which the various maladaptive changes are likely to be irreversible also need to be determined.
Effects of motor activity on brain and muscle development in cerebral palsy
Infancy is a unique period of neural development and plasticity
Interaction of brain and muscle injury and plasticity
Muscle plasticity in infants with cerebral palsy
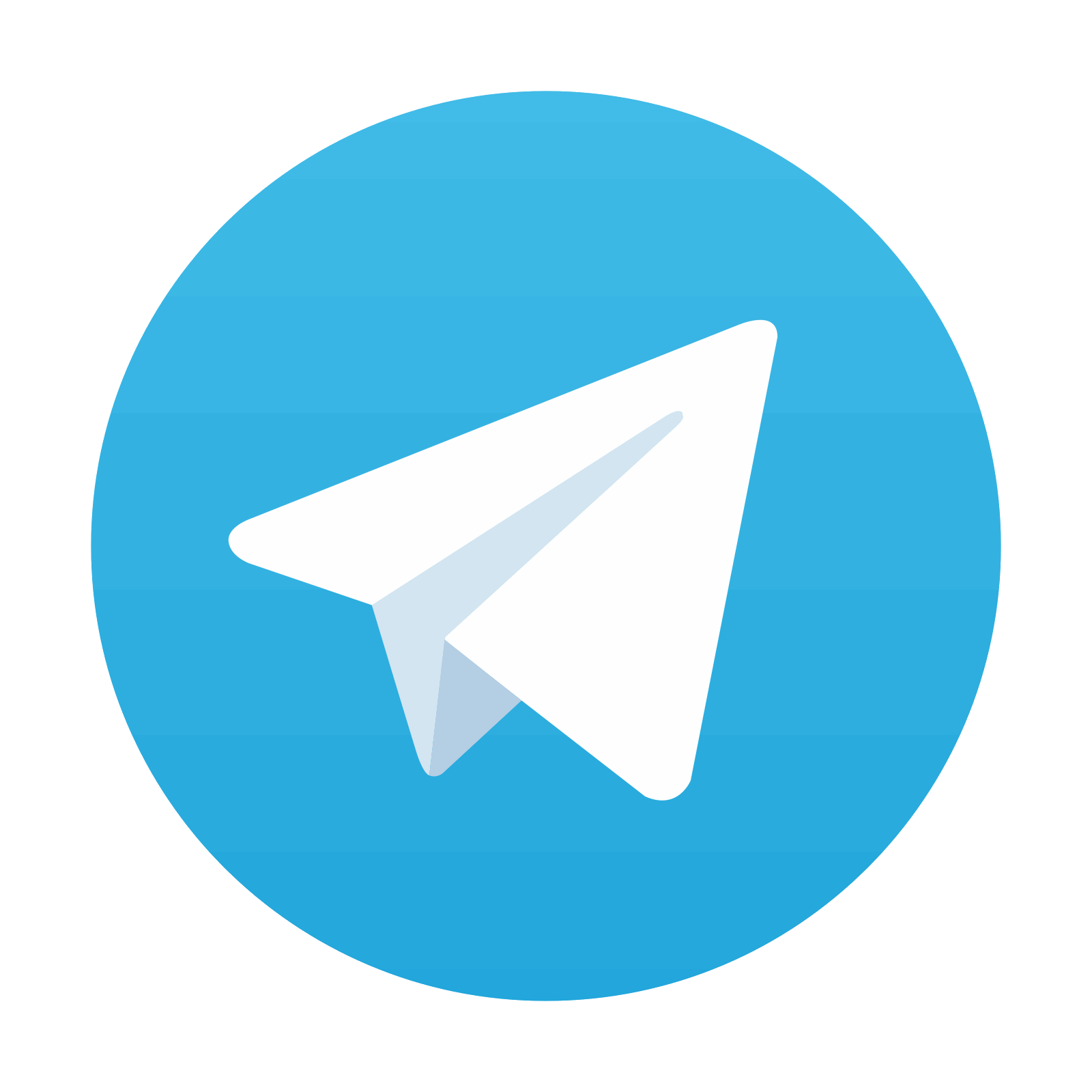
Stay updated, free articles. Join our Telegram channel

Full access? Get Clinical Tree
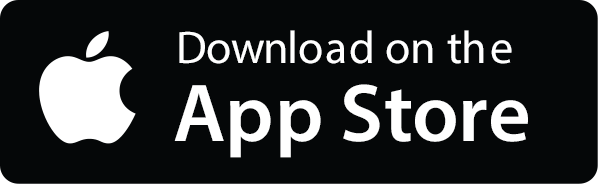
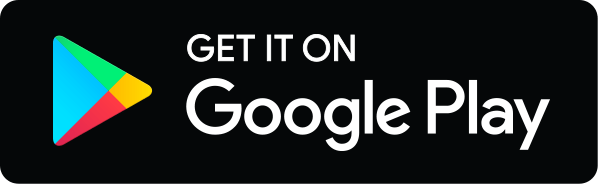