Fig. 34.1
Area of impingement between the polyethylene socket and the scapula. This is thought to be the cause of scapular notching in patients with reversed total shoulder implants
a.
As noted by Delloye et al. [8], progressive scapular notching may be of considerable concern as it may result in late glenoid-sided mechanical failures. Osteolysis in a joint with a well-fixed implant is a potentially devastating problem due to the substantial bone loss that may be associated with the failure of that device. The biologic reaction leading to aggressive bone resorption probably occurs when polyethylene and metal particles are released, as the polyethylene liner is abraded and the metal liner exposed [9, 10]. This process may be accelerated if a screw from the glenoid component is exposed from scapular bone loss and abrades the abutting humeral component.
The early experience with the Delta III, both its successes and shortcomings, inspired Dr. Mark Frankle to design the reverse shoulder prosthesis (RSP), which began in 1998 [11]. His rationale was to create a more anatomic alignment of the shoulder in an attempt to recapitulate the normal biomechanics of the shoulder and reduce the complications associated with the Grammont design. The basic concept of the design was to lateralize the humerus into a more anatomic position rather than lengthen the deltoid. This concept relied on two major modifications of the Grammont design: (1) creating more lateral offset with the glenosphere, which in some instances required moving the COR lateral to the glenoid and (2) maintaining a more anatomic inclination of the humeral component.
During the design process, he realized that there was a paucity of biomechanical data on the reverse shoulder arthroplasty design, and therefore, he embarked on numerous biomechanical studies in an attempt to understand the forces around and function of the prosthesis. This was done in an attempt to optimize the design and surgical technique, as well as to address the problems identified with previous designs.
The Reverse Shoulder Prosthesis
Initial Reverse Design
When the RSP was first designed, it consisted of four parts: (1) glenoid baseplate, (2) glenosphere, (3) humeral stem, and (4) polyethylene humeral socket [11]. The baseplate was fixed to the glenoid bone with a 6.5-mm central cancellous screw that was integrated into the backside of the baseplate. The central screw was supplemented with four peripheral, variable angle nonlocking 3.5-mm-diameter screws. There were two glenosphere choices: a 32-mm neutral glenosphere with a COR 10 mm outside the glenoid and a 32 minus 4-mm glenosphere with a COR 6 mm outside the glenoid. The glenosphere was attached to the glenoid baseplate via a Morse taper. On the humeral side, the titanium stem was cemented into the humerus and articulated with the glenosphere via the conforming polyethylene component. The polyethylene component was attached to a Morse taper using a screw-in system, and the Morse taper was locked into the humeral stem (Fig. 34.2).
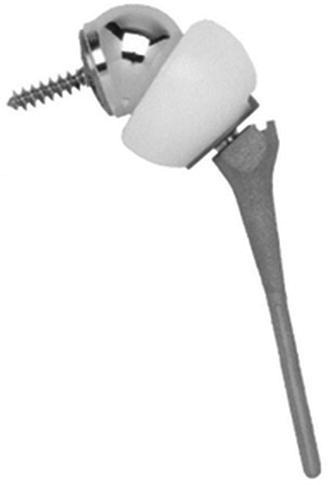
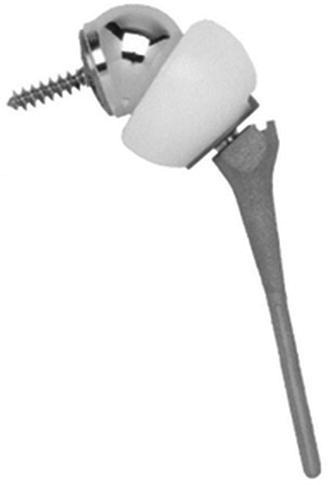
Fig. 34.2
The original design of the Reverse Shoulder Prosthesis™, shown without peripheral nonlocking screws in the baseplate. The all-polyethylene stem component would be later changed to incorporate a metal-backed socket
Complications that occurred during the clinical use of the initial RSP prompted several design changes that have modified this initial design into what it is today [11, 12]. The primary reason for providing a choice of two glenospheres with CORs outside the glenoid was to minimize inferior scapular notching, which has been seen in many patient series using reverse designs with a more medial COR [1, 3, 5]. Other goals for placing the COR more lateral in relation to the glenoid were to obtain a more anatomic COR for the shoulder, place the humerus into a more anatomic position, improve rotational strength, maximize glenohumeral motion, and restore the deltoid contour (Fig. 34.3a–d).
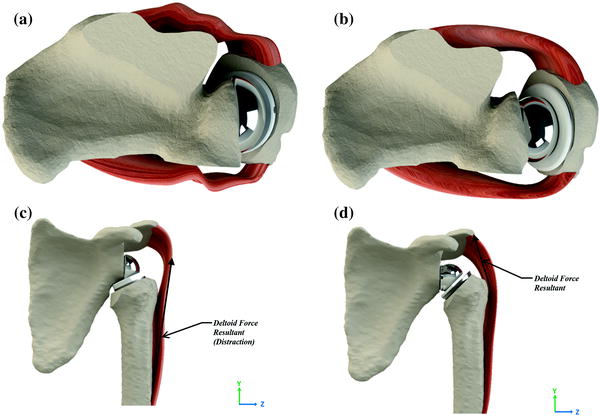
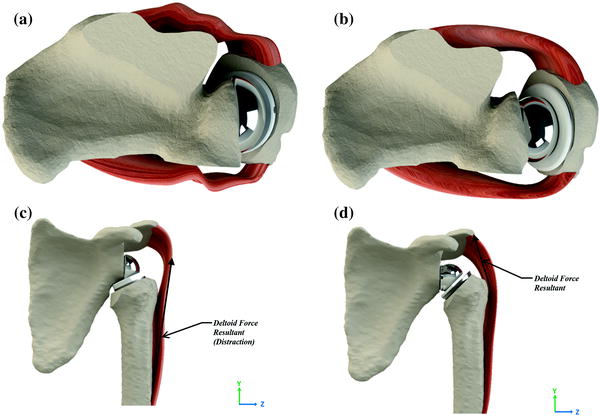
Fig. 34.3
Models depicting the restoration of tension between shoulder muscle groups. Medialization of the glenosphere results in insufficient tensioning of the subscapularis and the infraspinatus (a) that a lateral glenosphere accounts for (b). A medialized glenosphere results in inadequate contouring of the deltoid, resulting in a change in the direction of the force (c). A lateral glenosphere restores the contouring of the deltoid, bringing the direction of the force closer to the anatomic direction (d)
Current Reverse Design
The current reverse design consists essentially of the four parts described above with modifications based on clinical and scientific data described below. The glenoid baseplate still consists of a central 6.5-mm cancellous-type lag screw, integrated into the center of the baseplate. The backside of the baseplate is convex and was coated with hydroxyapatite, but is now manufactured with P2 (P-squared), a proprietary titanium porous coating to allow for osseous integration. The peripheral 3.5-mm nonlocking screws have been replaced by 5.0-mm fixed-angle locking screws. The two original glenosphere sizes are still available, and five additional sizes have been added to the standard set: 36 mm neutral, 36 minus 4 mm, 40 mm neutral, 40 minus 4 mm and 44 mm plus 8 mm. The distance of the COR from the glenoid with the currently available sizes ranges from 0 mm (40 minus 4-mm) to 10 mm (32 mm neutral) (Fig. 34.4).
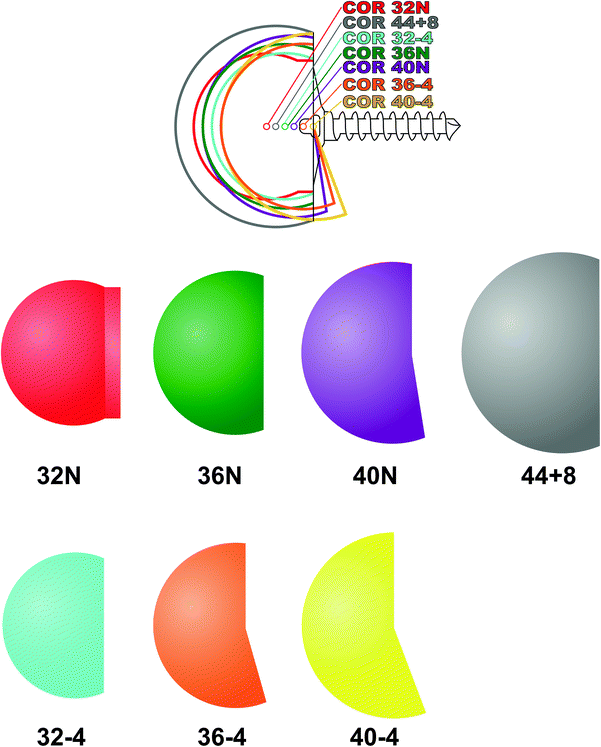
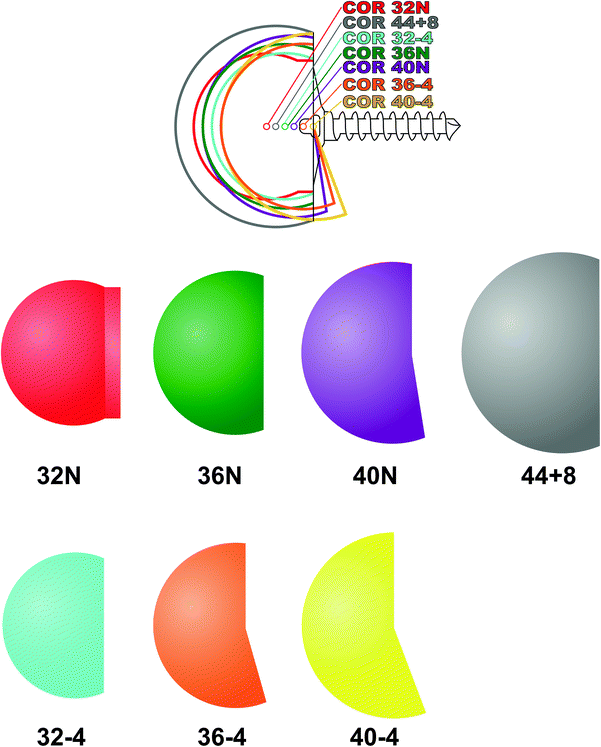
Fig. 34.4
Glenosphere sizes for the Reverse Shoulder Prosthesis™. The center of rotation is different for each size, with the exception of the 32-4 and 36 N spheres, as the two share the same center of rotation
The glenospheres lateralize the humeral component based on the distance from the baseplate to the lateral tip of the glenosphere and range from 20 to 26 mm. The glenosphere is attached to the baseplate via a Morse taper, with a central screw placed into the baseplate for additional stability. Two humeral stems are now available. The original titanium stem is cemented and articulates with the glenosphere via a modular titanium humeral socket and polyethylene insert. The humeral socket is available in neutral, +4 mm, and +8 mm heights, which will allow for additional soft tissue tensioning. The polyethylene liners are available in two heights, 0 mm and 4 mm, corresponding to the standard and semiconstrained liners, respectively. The semiconstrained liner deepens the articulation with the glenosphere compared to the standard insert. A monoblock titanium stem is now available which was initially designed for use in proximal humerus fractures, but can be used in any setting. The monoblock stem is also approved for use as either a cemented or noncemented press-fit implant. The variety of implant choices makes the RSP very versatile to treat a wide variety of clinical problems and anatomic differences. In order to get to the current prosthetic design, a great deal of research was performed to validate and optimize the design concepts.
The Glenoid Baseplate
If the ‘heart’ of the RSP design is the lateral offset, the design of the glenoid baseplate is the ‘backbone.’ Stable compressive fixation of the glenoid component, counteraction of the forces across the glenoid–bone interface, and reduction of micromotion at the interface to allow for osseous integration are features necessary for the long-term stability of the glenoid component. The essential biomechanical prerequisite for this process to successfully occur requires a stable interface between the bone and the prosthetic device during the initial healing phase. Excessive motion between the bone and prosthetic interfaces may result in failure of bone ingrowth and eventual mechanical failure of the device. The commonly accepted maximum motion that allows effective bone ingrowth is 150 μm [13].
Typical joint loads that occur after shoulder arthroplasty and during early recovery include up to one times body weight (756 N) [14]. The ability to tolerate the application of such loads while successfully allowing bone to integrate into the prosthesis is dependent on how the applied load is transferred to the interface between the prosthesis and the bone, as well as the security of the attachment. Methods used to ensure proper attachment of the prosthesis to bone include (1) improving the attachment strength to withstand the deforming loads transferred to the interface (improving fixation) and (2) limiting the deforming forces at the interface (immobilizing the shoulder, avoiding overtension of the reduction, or limiting the distance from the COR to the glenoid surface).
To provide adequate fixation, principles developed by the Association for the Study of Internal Fixation (ASIF) to improve fracture healing were used in the development of the RSP. A key ASIF concept is that compression across a fracture promotes successful bone healing. When applied to prosthetic implants, compression between the prosthetic surface and the prepared bone provides stability needed to allow bone ingrowth. To achieve compression, the RSP design uses a 6.5-mm cancellous-type lag screw in the center of the baseplate (Fig. 34.5).
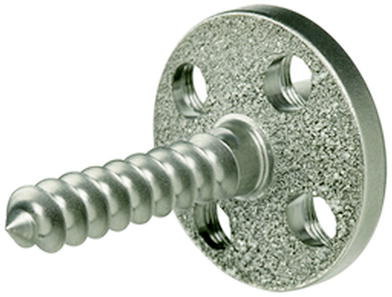
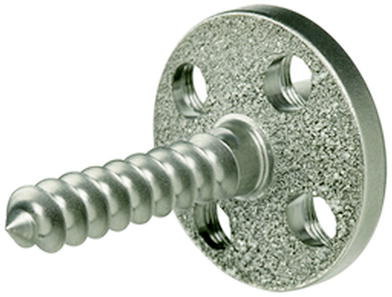
Fig. 34.5
The baseplate for the Reverse Shoulder Prosthesis™. Bony ingrowth is incorporated into the bottom of the base. The lag screw is integrated directly on the baseplate to achieve proper compression
The thread provides substantial compressive forces between the bone and the baseplate as the screw is driven into the bone. This differs from the Grammont design, and all others, which use a central post that does not provide compression.
It must be noted that a small amount of compressive force is provided in the Grammont design from the peripheral screws. A biomechanical study comparing the Grammont design of a central peg with the central screw fixation system of the RSP was performed [15]. The RSP baseplate provided 2000 N of compressive force, whereas the peg/peripheral screw system of the Grammont design baseplate provided 200 N of compressive force. The RSP baseplate, therefore, provides substantially more (an order of magnitude) compressive force at the bone–implant interface, suggesting a more optimal environment for osseous integration.
Baseplate micromotion is also an essential feature that must be reduced below the threshold of 150 μm to allow osseous integration. The initial design of the RSP baseplate used 3.5-mm nonlocking peripheral screws. A study was conducted to evaluate the initial glenoid component fixation of the three different designs (RSP 32 mm neutral, 32 minus 4 mm, and the Grammont 36 mm) [16]. Compressive and shear loads were applied to the glenoid components to create eccentric loading conditions. The results showed that fixation of the two available RSP glenospheres with 3.5-mm screws demonstrated increased baseplate micromotion compared with the Grammont design. However, micromotion for all devices was below 150 μm. This study substantiated the concept that a medial COR protected the glenoid construct by reducing stress and micromotion at the bone–implant interface. Additionally, it also validated the concept that a lateralized COR could be used and would still allow bone ingrowth to occur. Nevertheless, in clinical use, there were failures of the RSP baseplate. Of 267 shoulders implanted with the RSP using 3.5-mm peripheral nonlocking screws between 1998 and 2004, there were 21 (7.8 %) baseplate failures [11] (Fig. 34.6).
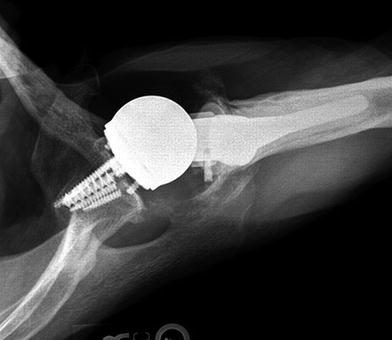
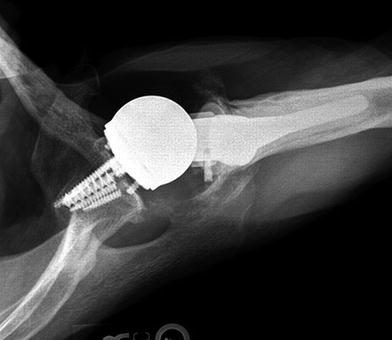
Fig. 34.6
X-ray showing a case of baseplate loosening caused by screw failure
Electron microscopic evaluation of two of the failed baseplates was performed [15]. Minimal bone ingrowth was observed in the porous coating on the undersurface of the baseplate. The striations of the central screw could be accurately characterized as fatigue failure. While the cause of ingrowth failure is likely multifactorial, one factor that could be addressed biomechanically was improved baseplate fixation.
Based on the early clinical results, the concept of using peripheral locking screws was employed to enhance baseplate fixation. Experiments were performed to assess the fixation using 5.0-mm peripheral locking screws [16]. The stability of fixation was measured as the amount of micromotion at the baseplate. Two variations of the RSP glenosphere (32 mm neutral and 32 minus 4 mm) were tested in addition to a 36-mm glenoid component of the Grammont design. These devices had varying lateral offsets, defined as the distance from the glenoid baseplate to the center of articular contact between the glenosphere and the polyethylene cup. There were no significant differences in component motion when the Grammont components fixed with 3.5-mm screws were compared with the two RSP components fixed with 5.0-mm locking screws, and all had micromotion less than 150 μm. A second experiment analyzed the relationship of lateral offset with micromotion [17]. Lateral offset was varied from 2 to 30 mm, and fixation with 3.5-mm nonlocking or 5.0-mm locking screws was tested. A positive linear relationship was shown between RSP baseplate motion and lateral offset when either four 3.5-mm nonlocking screws or four 5.0-mm locking screws were used for baseplate fixation. Additionally, RSP baseplates fixed with 5.0-mm locking screws had up to 29 % less motion than RSP baseplates fixed with 3.5-mm nonlocking screws, and motion was less than 150 μm even at 30 mm of lateral offset. Overall, both lateral offset and peripheral screw type affected the magnitude of baseplate motion. Additionally, stable fixation was achieved for the RSP 32-mm neutral components fixed with 5.0-mm locking screws despite a substantially (69 %) greater moment at the baseplate–bone interface compared with the Grammont design. The results of this study advocate the use of 5.0-mm locking screws when implanting the glenoid component, and currently is the recommended fixation method for the RSP in healthy bone (Fig. 34.7).
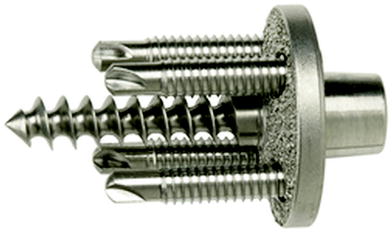
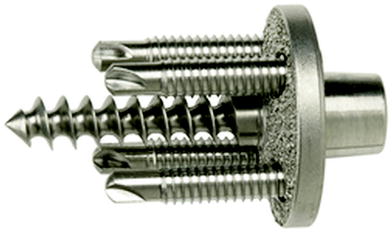
Fig. 34.7
The locked screws in the RSP™ resist shear and torsional forces, and offer 2000 N of compression alongside the lag screw to promote contact with the bony ingrowth surface
The Glenosphere
The initial design concept of the RSP glenosphere was returning the lateral offset (the distance of the humeral shaft from the glenoid surface) to a more anatomic position. This would presumably improve range of motion of the implant before implant–bone impingement would occur. In adduction, the offset would reduce impingement on the inferior scapular neck, thereby reducing scapular notching. In abduction, the lateral offset would provide a larger arc of motion before impingement on the acromion or superior glenoid occurred. Additionally, lateral offset would restore the deltoid contour and tension the remaining rotator cuff muscles so that they could function more efficiently. The addition of larger glenosphere sizes would allow for improved range of motion and also move the center of rotation closer to the glenoid, which as described above reduces the forces and motion at the baseplate.
Muscle function was assessed in vitro using a sawbones model as well as a mathematical calculation to determine the moment arms of the deltoid, subscapularis, and infraspinatus. Six RSP constructs and a 36-mm Grammont design were tested [11]. All reverse designs showed similar linear increases in moment arms throughout the range of abduction. The moment of the subscapularis and infraspinatus changes from adduction to abduction as the arm elevates above the prosthetic COR. The greater moment arms of the RSP in internal and external rotation demonstrate a mechanical advantage in having the COR lateral to the glenoid. A clinical study also showed improved strength before and after RSP implantation for rotator cuff arthropathy [18]. However, this study did not fully address how implant design affects motor strength and function. Nevertheless, indirect comparison of external rotation improvement using the RSP with a Grammont-style implant does show consistently better external rotation with the RSP design.
Glenosphere size can also improve function and stability of the RSP. Impingement-free ROM has been shown with larger sizes, but is likely dependent on other factors such as glenosphere position, which are related to surgical technique (described below). However, as described above, the larger glenosphere sizes available for the RSP have the COR closer to the glenoid surface. This has the effect of reducing micromotion at the baseplate, but retaining the advantage of increasing lateral offset of the humerus, thereby reducing impingement. An additional advantage of the glenospheres is that they overhang the edge of the baseplate and can directly contact the glenoid surface. An in vitro study showed that glenosphere contact with the bone decreases micromotion compared to when there is no glenosphere contact. Additionally, the larger glenosphere sizes had a greater reduction in micromotion [19].
Selection of glenosphere size depends on several factors including patient size, bone quality, and the condition being treated. Ultimately, the goal is to balance ROM and long-term stability of the implant.
The Humeral Component
The initial design of the humeral component consisted of a modular system, with a humeral stem and an all-polyethylene socket (Fig. 34.2). The polyethylene socket was attached to a metal button that was assembled to the humeral shaft via a Morse taper. The humeral construct was cemented into place with the socket insert into the proximal humeral metaphysis. The design differed from the initial Grammont design by the shape of the humeral stem, the all-polyethylene socket, attachment of the socket to the stem, and the inclination of the socket. The humeral stem of the RSP is rectangular at the proximal aspect, which is in contrast to the conical shape of the Grammont prosthesis. The all-polyethylene humeral socket attached via Morse taper differed from the metal socket and polyethylene insert of the Grammont prosthesis, which screwed into the humeral stem. Finally, and likely, the most important difference was a more anatomic humeral inclination of 135° compared to the nonanatomic 155° of the Grammont prosthesis [20, 21] (Fig. 34.8a, b).
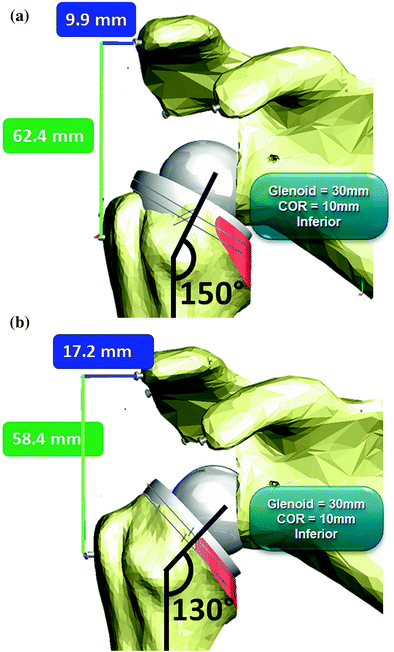
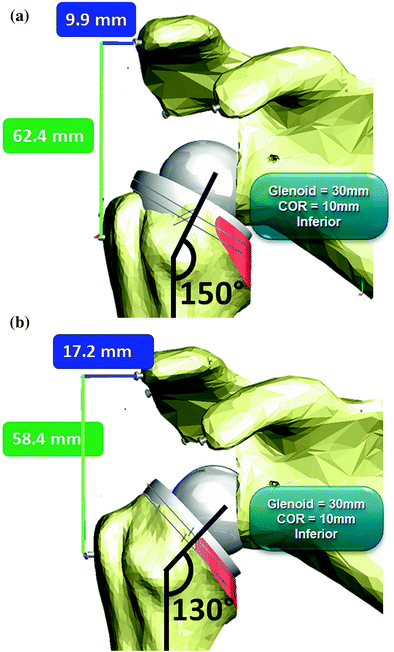
Fig. 34.8
Models of humeral-sided lateralization. A valgus implant results in an inferior displacement of 4 mm (a). A varus implant causes a greater lateral displacement of 7 mm (b)
Early experience with the all-polyethylene component resulted in a modification of the RSP design to a metal humeral socket with a polyethylene liner that allowed a more stable fixation of the polyethylene to the humeral component and easier exchange of the polyethylene to different sizes. These components comprise the currently available modular RSP system.
The Modular Humeral Component (Fig. 34.9)
As mentioned above, the humeral socket is available in standard, +4 mm and +8 mm heights. The plus sizes increase the length and lateral offset of the humerus, which further tensions the soft tissue. The polyethylene insert is available in a standard depth, as well as semiconstrained, which has a deeper socket. The advantage of the deeper socket is improved stability by increasing the translational force necessary to dislocate the glenosphere from the socket [22]. However, the improved stability is gained at the expense of impingement-free ROM. The increased depth of the socket results in medialization of the humeral component and less lateral offset, thereby decreasing the ROM before implant impingement occurs.
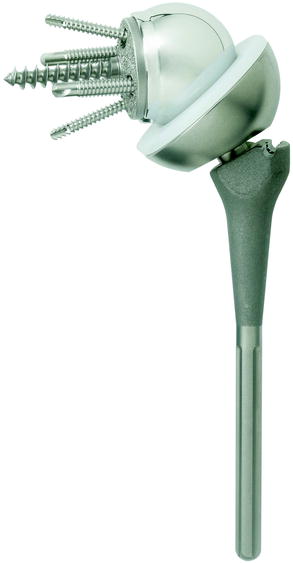
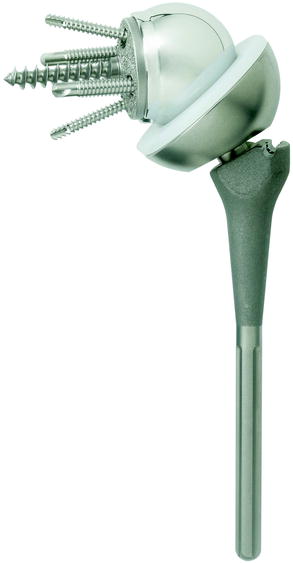
Fig. 34.9
The modular RSP®
The Monoblock Humeral Component
The modular humeral component has been the standard implant for the modern reverse shoulder arthroplasty. However, humeral-sided complications, particularly loosening and dissociation, did occur [4]. It was evident that these problems were a significant issue, especially in the setting of proximal humeral bone loss, which commonly occurs with proximal humeral fractures, revisions of shoulder arthroplasty, and proximal humeral tumors. The torsional forces with shoulder motion likely caused increased stress on the humeral component when there was less proximal humeral bone support. A study to test this concept was performed using both modular and monoblock humeral components and comparing the stability of these implants in the presence and absence of proximal humeral bone [23]. The study showed that the presence of proximal humeral bone improved the stability of the humeral components and that proximal humeral bone loss placed the implants, particularly modular implants at increased risk of failure. Therefore, a monoblock RSP stem was designed in an effort to improve the stability of the humeral component.
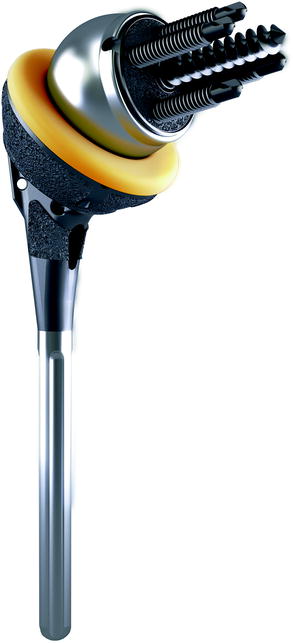
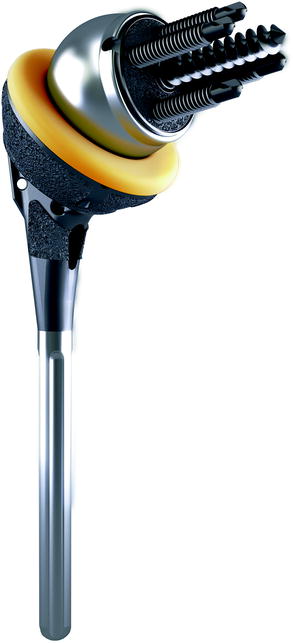
Fig. 34.10
The AltiVateTM Reverse® system incorporates advanced fixation technologies and precision instrumentation.
The most recent update to the RSP system is the AltiVate (Fig. 34.10). It has a monoblock humeral stem design but with additional features such as integrated fins & suture holes for tuberosity reduction and fixation, highly crosslinked vitamin E polyethylene, a bone graft window, and P2 porous coating.
The Overall Geometry: Restoring Anatomy
The overall design concept of the RSP combines a lateralized COR (relative to the glenoid) and an anatomic neck–shaft angle. The effect of combining these features, while not completely restoring the anatomic relationship of the glenohumeral joint, does restore a more anatomic alignment of the humeral shaft relative to a more medialized implant with a higher neck–shaft angle. The geometry of the reverse causes some obligatory lengthening of the arm; however, the lower neck–shaft angle reduces the overall lengthening of the arm. Additionally, the diameter of the glenosphere contributes to lengthening. The advantage of the lateral COR and anatomic neck–shaft angle is realized by allowing lateralization of the humeral shaft, which creates more tension on the soft tissue. The reduced lengthening also preserves a more anatomic alignment of the rotator cuff. Both of these provide a better mechanical environment for the rotator cuff to assist with shoulder motion, particularly external rotation [3, 7, 24–28]. Additionally, as shown by De Wilde et al. [29], the combination of lengthening and lateralization of the humeral shaft improves the function of the deltoid by increasing the lever arm of the deltoid, as well as preserving the wrapping angle of the deltoid.
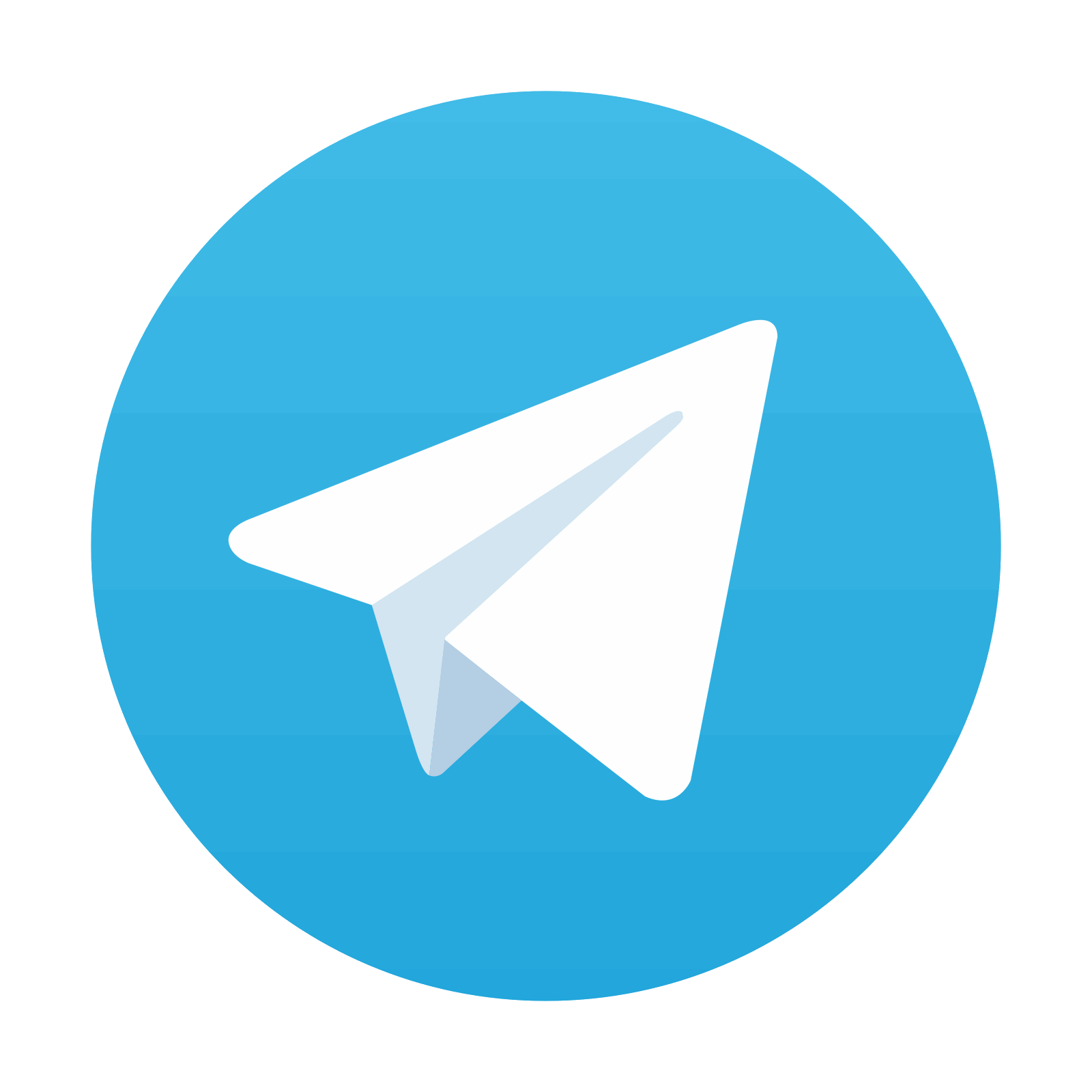
Stay updated, free articles. Join our Telegram channel

Full access? Get Clinical Tree
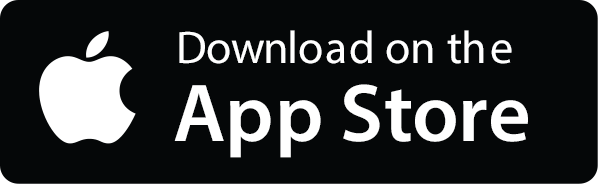
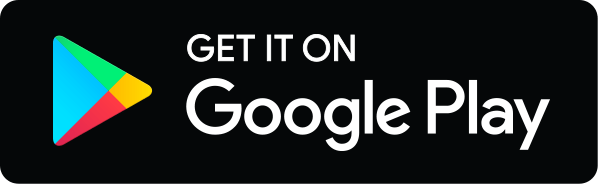