6
Discosis
Definition
The term “discosis“ encompasses all biomechanical and pathoanatomical changes in the intervertebral disk that are associated with disk degeneration.
Synonyms: Disk degeneration, degenerative disk disease, chondrosis intervertebralis.
Classification
There are three stages of discosis (Fig. 6.1):
the first, or early, stage, in adolescence and young adulthood
the second stage, in middle age
and the third stage, in old age.
The first stage of discosis begins with regression of the intradiscal vessels between the ages of 2 and 4 years.
From this time onward, the disk tissue receives nutrients only by diffusion. In childhood, the disk continues to function adequately as an osmotic system, so that its fibroblasts and chondrocytes are assured a sufficient supply of nutrients. The lower body weight of children and, above all, their relatively high degree of physical activity create favorable biomechanical conditions for the nourishment of the fiber-producing cells of the intervertebral disk. As body weight increases, so does the axial load on the disk, particularly in the lower lumbar segments. Furthermore, as children grow into adolescents and adults, their level of physical activity decreases as they become relatively sedentary, first at school and then at work. The fibrous lamellae of the disk, which are no longer adequately nourished and thus no longer able to withstand the strong outward pressure of the central, mobile disk tissue, begin to protrude outward. The earliest disk-related complaints may arise at any time between age 12 and age 20, when intradiscal shifting of disk material suddenly causes stretching or bulging of the posterior longitudinal ligament. In adolescents, this produces the clinical picture of iliolumbar extension stiffness (see Chapter 11).
a, b Sagittal section of the L4–L5 motion segment (anatomical preparation). Disseminated calcifications in the dorsal lamellae of the anulus fibrosus and the posterior longitudinal ligament. Isolated focus in the ligamentum flavum (arrow). Note the facet joint space (arrow). “Geographical” pattern of the disk in both the nucleus pulposus and the anulus fibrosus. The disk protrusion extends beyond the posterior edge of the vertebral body. Fissures in the anulus fibrosus: discosis, stage 2.
c, d L3–L4 motion segment, with a fissure in the anulus fibrosus extending into the upper cartilaginous end plate of L4, with displacement of disk tissue into the vertebral body. Geographical disk structure and fissures, corresponding to stage 2 discosis (from Ludwig, Tiedjen, and Kramer 2004).
The second stage of discosis affects adults aged 20–60. The poorly nourished fibroblasts and chondrocytes produce poorer-quality fibers that are no longer able to withstand the expansile pressure of the central, mobile portion of the disk, which, at this time of life, is still intact. The anulus fibrosus develops both radial and circular tears, into which the tissue of the nucleus pulposus protrudes. Entire sequestra composed of tissue from the anulus fibrosus and the cartilaginous end plates can form and then, under asymmetrical axial loading, follow the path of least resistance outward, sometimes beyond the boundary of the intervertebral disk. Disk protrusions and prolapses are the result. As long as the external layers of the anulus fibrosus are intact, it is still possible for the displaced tissue to return to its original position. In a disk prolapse, however, the displaced tissue penetrates the anulus fibrosus and can no longer go back inside the disk. Dorsally or dorsolaterally pointing disk prolapses come into contact with the posterior longitudinal ligament, which derives its sensory nerve supply from the meningeal branch of the spinal nerve. The resulting symptoms consist of locally circumscribed lumbago, chronic discogenic low back pain, and/or root compression syndrome (Fig. 6.2).
Fig. 6.3a–d Comparative gross and microscopic findings of advanced discosis (stage 3) (from Ludwig, Tiedjen, and Kramer 2004).
a L5/S1 intervertebral space, with subtotal destruction of the disk, loss of height, and focal zones of contact between the upper and lower end plates (magnification 4×).
b Histological section of the central part of the intervertebral space, with fibrous connective tissue deposits between the trabeculae of the bone marrow space. The intervertebral space contains a number of bony trabeculae. Same specimen as a (magnification 63×, H & E stain).
c Macroscopic photograph of disk tissue entering the vertebral body end plate at L4/5. Marked loss of disk height and end plate sclerosis. Consolidated Schmorl’s node under the inferior vertebral body end plate.
d The histological section corresponding to c. Concentric, fibrous lamellae of connective tissue surround the displaced tissue. Fat is seen in the neighboring marrow space (magnification 63×, H & E stain).
Pathoanatomical changes within the disk tissue, including fiber disruption, tissue loss due to prolapse, and dehydration, produce a further manifestation of the second stage of discosis: segmental instability. Slackening of the initially tautly elastic disk tissue loosens the mechanical link between adjacent vertebrae, enabling movements of nonphysiological amplitude and direction. This kind of instability may not be directly visible. It can often be detected only with the aid of functional images, e. g., lateral radiographs on forward and backward bending. Degenerative instability also causes slippage (olisthesis) of the vertebrae relative to one another, either ventrally or dorsally (retrolisthesis). Symptoms are produced by impingement on the neural elements in the vertebral canal or the intervertebral foramen. Most therapeutic procedures are undertaken in the second stage of discosis, because it is in this stage that disk displacement and slackening most commonly produce intense local and radicular manifestations.
In the third stage, the displacement of central, mobile disk tissue (loosening, protrusion, prolapse) ceases to progress. After age 60, the disk tissue loses water and becomes fibrotic and firm, and therefore less liable to dorsal displacement. Calcification of the ligaments that span the intervertebral space as part of the spondylotic process contributes further to the fixation of the motion segment (Figs. 6.3, 6.4). The pathoanatomical changes may appear quite impressive at this stage, but, as their net effect is partial stabilization, the patient is usually spared severe symptoms. This is the so-called “comfortable rigidity of the aging spine.” The age distribution curves for disk diseases show that protrusions and prolapses do, indeed, occur in old age, but with a different pattern than in middle age, mainly affecting the upper lumbar segments and producing extradiscal sequestra that are projected craniolaterally. Spondylogenic symptoms after age 60 tend to be caused not by disk pathology per se, but by secondary degenerative changes in the dorsal part of the motion segment. The most common problems are spondylarthrosis and degenerative spinal canal stenosis, with chronic radicular irritation syndrome due to lateral bony compression of the nerve roots, manifested as spinal claudication.
Fig. 6.4 a, b Advanced discosis (stage 3) with ventral osteophyte formation and condensation of the adjacent bone marrow space (arrow) (from Ludwig, Tiedjen, and Kramer 2004).
Etiology
The spine of a quadruped consists, in principle, of a horizontal chain of identical segments supported at two points. The vertical human spine is subject to much greater gravitational stresses.
We humans owe disk disease mainly to our upright posture.
Schmorl’s nodes, i.e., circumscribed protrusions of disk tissue into the vertebral end plates, are not found in quadrupeds. The vertebral columns of certain flightless birds, whose bipedal stance places gravitational stress on the spine much as it does in humans, display abnormalities of a comparable type (Klapp 1958). Exner (1954) described severe forms of spondylosis deformans in anthropoid apes with upright posture. Yamada (1962), Wassilev and Dimova (1970), and Cassidy et al. (1988) forced mice to walk upright by amputating their forelegs. One year later, the anulus fibrosus and cartilaginous end plates displayed structural changes attributable to vertical stress. Similar observations in animals were made by Palmer and Lotz (1993), Edwards et al. (1998), and Unglaub et al. (2003).
Though genetic differences between individuals are important, the main cause of generalized disk degeneration in humans is the premature aging of bradytrophic tissue induced by static mechanical forces.
The human intervertebral disk does not begin as bradytrophic tissue. In infancy, when the disk is not under any particular mechanical stress, intense metabolic exchange takes place across the intradiscal vessels. Twisting and bending of the trunk, as the infant crawls, further aid fluid exchange across the boundaries of the disk.
The assumption of an upright posture fundamentally alters the biomechanical situation of the disk. The intravertebral vessels are protected by the bony trabecular system, but the vertical compressive pressure on the disk (the sum of the weight of the body above the disk and the muscle tone across it) is transmitted directly to the blood vessel walls within the pulpy, homogeneous disk tissue. Because the arteriolar and venous pressure is lower than the hydrostatic pressure, the blood is pressed out of these vessels, which collapse, suffer pressure atrophy, and finally disappear. The nutrient supply of the fibroblasts and cartilage cells diminishes in a relatively short time because they are isolated from the bloodstream. Metabolic exchange can now take place only across long distances within the disk tissue, through transport mechanisms that are less effective than the previous normal vascularization.
Insufficient nutrient supply is associate with a lowered oxygen/glucose concentrations and acidic pH, and adversely affects the ability of disk cells to synthesize and maintain the disk matrix leading to disk degeneration (Mokhbi-Soukane 2006).
The disk is the largest contiguous structure in the body that lacks its own blood supply.
The developing disk, accustomed to adequate perfusion, low mechanical stress, and much movement, is suddenly subjected to new, unfavorable conditions when the infant begins to stand and walk. The inadequate capacity of the cells within the human disk to adapt to the new situation leads to a qualitative degeneration of its connective and supportive tissue, with early signs of wear and tear. This explains why degenerative disk disease sometimes occurs in childhood. Töndury (1968) found microscopic signs of degeneration in the disks of 4-year-old children. The anatomical and clinical data imply that discopathy in all three major regions of the spine can occur at puberty or earlier (Idelberger 1959). The youngest patient undergoing surgery for disk prolapse in the series of Daschner et al. (1971) was 13 years old; in three other series, the youngest patients were 10, 16, and 9 years old (Ford 1960, Mittelmeier 1970, Seyfarth 1970). Of the 2755 patients operated on for discogenic neural compression by the Düsseldorf University Neurosurgical Clinic (Barghoorn 1975), 36 were 18 years old or less; these patients mainly suffered from massive monoradicular sciatica. There are many other reports of disk prolapse in childhood (Key 1950, King 1959, O’Connell 1960, Epstein and Lavine 1964, DaSilva et al. 1977, Parsch and Eulenburg 1983, Bradbury 1996, Ishihara 1997, Panayiotis 1998, Balagué 2001, Schlenzka 2001, Arendt 2002, Kjaer 2005).
Beyond age 30, it is rare to find a human spine that shows no sign of degenerative changes in the disks.
In most individuals, the active and passive transport mechanisms across the boundaries of the disk cannot sustain, over the decades, a constant, adequate supply of nutrients to its cells.
A sedentary lifestyle with prolonged maintenance of unfavorable postures impairs movement-dependent fluid shifts across the boundaries of the disk.
Trauma and transient overloading impair the nutrient supply of the disk cells much less than a prolonged absence of movement.
In addition to the verticality of the spine and invariance of posture, genetic factors also contribute to the early appearance of regressive changes in the intervertebral disks. An endogenous component is postulated by many authors (Hanraets 1959, Armstrong 1965, Schmorl and Junghanns 1968, Wilson 1968, Braun 1969, Brocher 1973, Töndury 1981, Idelberger 1984, Simmons 1996, Matsui 1998, Kawaguchi 2002, Battié 2004, Bernadino 2004, Lattermann 2004). According to Idelberger (1952), some individuals have a constitutional predisposition to premature disk fatigue. Hanraets (1959) and Wilson (1968) showed familial clustering of disk disease. According to Wilson (1968) and Beard and Stevens (1985), the quality and configuration of the collagen fibers in the anulus fibrosus are influenced by genetic factors. Battié and Videmann (2004) found that near relatives of patients with disk prolapses are more likely to have spine-related complaints than near relatives of normal control individuals. Hestbaek et al. (2006) in their twin study demonstrated a correlation between low back pain in childhood/adolescence and low back pain in adulthood.
The existence of an inherited predisposition to disk disease thus cannot be rejected out of hand, but there is no apparent connection to any particular habitus, as is the case for some other diseases (diabetes mellitus, peptic ulcer disease). Our own studies in a large set of patients have unequivocally shown that disk lesions are equally common in individuals of athletic, leptosomal, pyknic, or dysplastic build (Krämer 1978).
Videman et al. (2006) identified new genes associated with signs of disk degeneration and presents evidence of a multigenic etiology.
Pathogenesis
The worsened metabolic situation beginning in childhood alters not only the chemical composition, but also the anatomical structure of the intervertebral disk. Because of their inadequate supply of energy and nutrients, the fibroblasts form fibers and matrix of deficient quality, and finally disintegrate. Many histological studies of disk material from unselected autopsy cases have shown this to be the case. The degenerative process begins in the disk (Eubanks 2006).
Overview of the literature. Basic studies have been undertaken by Hirsch and Schajowitz (1952–1953), Hirsch et al. (1953, 1963), Hirsch and Nachemson (1954), Hirsch (1960, 1966), Hirsch and Bobechenko (1965), Feng (2000), Roughley (2004), Battié and Videmann (2004), An et al. (2004), Crock (2004), Hansson (2004), Naturajan et al. (2004), Haefeli et al. (2005), Roh et al (2005), An and Masuda (2006), Brisby (2006), Guehring et al. (2006), Natarajan (2006), Setton (2006), and Unglaub et al. (2006). Growth factors, such as bFGF and TGF-β1, macrophages, and mast cells may play a key role in the repair of the injured anulus fibrosus and subsequent disk degeneration (Peng 2006).
Kuhlendahl and Richter (1952) found fatty deposits in the disk matrix of adolescents as well as occasional fat droplets in the cells of the anulus fibrosus. Among young adults (aged 25–40), they found further regressive structural changes including incipient dehydration, sharper contours of the fibrous lamellae, and blurring of the border between the anulus fibrosus and the nucleus pulposus. Histological studies regularly show degenerative changes in all structural components of the intervertebral disk beginning in the third decade of life, with cell degeneration, cell loss, fiber degeneration, and loosening of the matrix (Püschel 1930, Schaffer and von Möllendorf 1930, Erlacher 1949, Coventry et al. 1954, Harris and Macnab 1954, Lang 1962, Dahmen 1966, Rothman and Simeone 1982, Miller et al. 1988). Harris and Macnab (1954) studied the histology of adult nucleus pulposus tissue and found only pyknotic, clumped, degenerate, or shadow cell nuclei. Electron microscopy of adult disk tissue revealed irregular, disorganized fibrils of altered density and transverse striation (Dahmen 1966). Further extensive descriptions of histological changes in the intervertebral disk can be found in many publications (Friberg and Hirsch 1950, Lindemann and Kuhlendahl 1953, Güntz 1958, Schmorl and Junghanns 1968, Töndury 1981, Rothman and Simeone 1982, Wiesel and Rothman 1982, Genant 1984, Kirkaldy-Willis 1984, Roberts 1985, Rauschning 1986, Bell et al. 1990, Bernick et al. 1991, An 2004, Crock 2004, Hanson 2004, Bantrizos et al. 2005, Gruber et al. 2007, Waris et al. 2007, Zhang et al. 2008). Studies are under way to describe matrix constituents as targets in matrix breakdown (Feng 2006). The etiology of symptom manifestation in lumbar and cervical spine degeneration is multifactorial and includes cellular, biochemical, and biomechanical causes (Roh 2005).
Fig. 6.5 a–c Cavitary system of the third lumbar disk of a 42-year-old man with stage 2 discosis (a, b), and of a 66-year-old man with stage 3 disease (c).
Biology offers several strategies for restoring the degenerating disk, including the use of recombinant or natural proteins that increase matrix accumulation and assembly, enhance the number of disk cells, or in other ways lead to restoration of the native healthy disk. Recombinant bone morphogenetic protein-7 (osteogenic protein-1) shows promise in this regard. Other growth factors, as well as cytokine antagonists such as the interleukin-1 receptor antagonist, are also good candidates (Evans 2006).
As disk degeneration progresses, concentric and radial fissures develop in the disk. The cavitary system of the nucleus pulposus enlarges and connects to the fissures of the anulus fibrosus (Fig. 6.5).
The cavitary system and fissures can also be demonstrated by discography. An intradiscal cavity can be punctured transcutaneously and filled with contrast material or medication. The more severely the disk is degenerated, the greater the amount of fluid that can be injected into it at low pressure (Panjabi et al. 1988). In younger patients, this amount is of the order of 1–2 mL. In older patients, a lumbar disk may admit as much as 5 mL of injected fluid within its confines, without any damage to the borders of the disk or spillage of fluid outside it.
Collections of gas in the cavitary system of the disk are seen in plain radiographs as the so-called “vacuum phenomenon” (Fig. 6.6) (Knutsson 1944, Hoeffken 1951, Raines 1953, Armstrong 1965, Schmorl and Junghanns 1968, Genant 1984). The cause of this phenomenon and the composition of the gas are currently unknown.
Yellow and brown discoloration of disk fibers has also been described as a sign of disk degeneration. Güntz (1958), in a systematic study, found that such discoloration occurs only when fissures and tears in the disk tissue extend through gaps in the cartilaginous end plate into the vertebral body and make contact with the vascular structures originating in the bone marrow.
The term “discosis” is apt, because the degenerative process affects not only cartilage, but all of the other portions of the intervertebral disk as well.
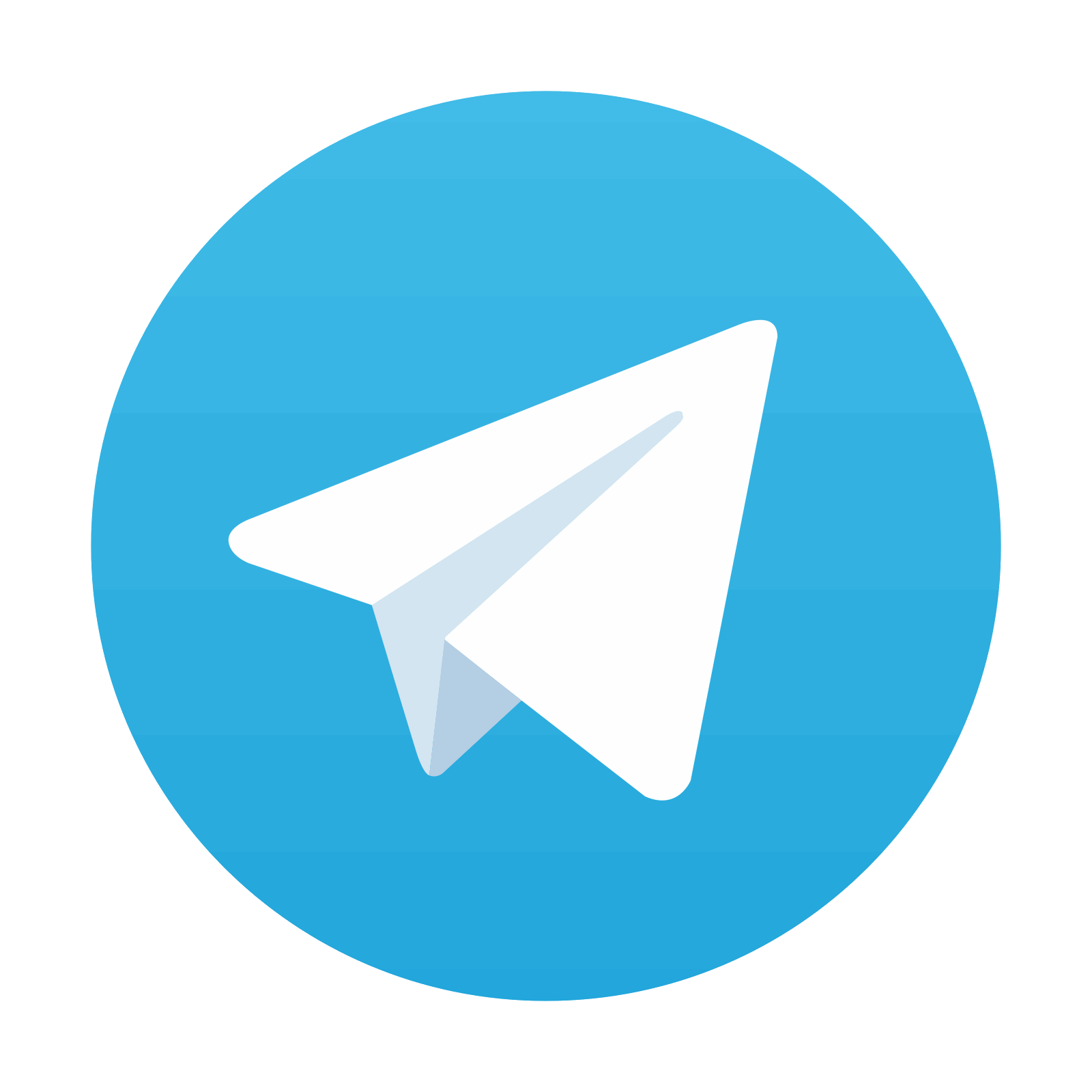
Stay updated, free articles. Join our Telegram channel

Full access? Get Clinical Tree
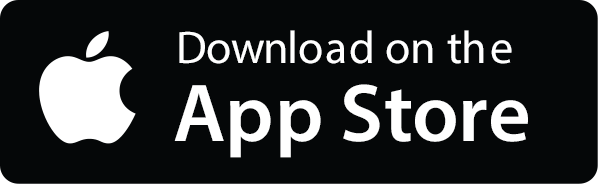
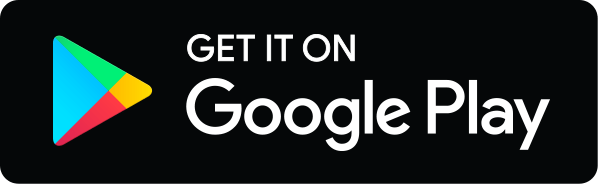