Fig. 7.1
Number of PubMed entries for sarcopenia and cachexia as respective search words (Assessed on 1 February 2016 from www.pubmed.gov)
To date, pharmacologic therapies for the treatment of sarcopenia and cachexia are largely still in development [4, 5]. When medications designed to treat sarcopenia are used, they can be enhanced by a regimen that also includes, when appropriate, resistance training, early ambulation, nutrition management, protein and amino acid supplementation, and smoking cessation [4]. For older people with sarcopenia and/or cachexia, the concept of rehabilitation and nutrition care management in combination is considered a useful approach to treatment [6]. In this review, we address the epidemiology, diagnostic criteria, causes, and therapies focusing on physical exercise/activity and nutrition as a comprehensive approach for the treatment of sarcopenia and/or cachexia.
7.2 Sarcopenia
7.2.1 Definition and Epidemiology
The term sarcopenia, first used by Rosenberg in 1989 to describe an age-related decrease in muscle mass, originated from the Greek words sarx, meaning flesh, and penia, meaning loss [7, 8]. Initially, this term denoted only the loss of muscle mass. In 2010, the European Working Group on Sarcopenia in Older People (EWGSOP) described sarcopenia as a syndrome characterized by the progressive and generalized loss of skeletal muscle mass and strength, associated with a risk of adverse outcomes such as physical disability, poor QoL, and death [1]. In 2011, the International Working Group on Sarcopenia defined sarcopenia as an age-associated loss of skeletal muscle mass and function [3]. Decreased muscle strength and physical function are also now included in the definition of sarcopenia.
According to a systemic review, the prevalence of sarcopenia in older adults, using the EWGSOP definition of sarcopenia, was 1–29% in community-dwelling populations, 14–33% in long-term care populations, and 10% in the acute care hospitalized population [9]. The prevalence of sarcopenia in patients who underwent surgery for gastrointestinal and hepatopancreatobiliary malignancies was 17–79% [10]. In another systematic review of patients with pancreatic ductal adenocarcinoma, the prevalence of sarcopenia among individuals of normal weight (body mass index [BMI] 18.5–24.9 kg/m2) and those who were overweight or obese (BMI >25 kg/m2) was 9.7–65% and 16.2–67%, respectively [11]. The prevalence of sarcopenia in older people in rehabilitation settings was 40–46.5% [12, 13].
7.2.2 Diagnostic Criteria
Sarcopenia is diagnosed by low muscle mass and either low muscle strength or low physical performance according to the EWGSOP definition [1]. This definition is similar to the criteria put forth by the Asian Working Group for Sarcopenia (AWGS) [14]. The International Working Group on Sarcopenia (IWGS) considers that sarcopenia is diagnosed by low muscle mass and low gait speed [3], whereas the Foundation for the National Institutes of Health (FNIH) sarcopenia project indicates that sarcopenia is diagnosed by low muscle mass and low muscle strength [15]. Sarcopenia is no longer diagnosed on the basis of low muscle mass alone, although the diagnostic criteria of sarcopenia are different between research groups. Presarcopenia is characterized by low muscle mass without considering the effects on muscle strength or physical performance, and severe sarcopenia is characterized by low muscle mass and low muscle strength, in addition to low physical performance as per the EWGSOP definition [1].
7.2.2.1 Muscle Mass
Appendicular muscle mass is most commonly assessed using dual X-ray absorptiometry (DXA) and bioimpedance analysis (BIA). However, the cutoff reference values for appendicular muscle mass are different between research groups. The AWGS divides appendicular muscle mass by height squared (DXA, 7.0 kg/m2 for men and 5.4 kg/m2 for women; BIA, 7.0 kg/m2 for men and 5.7 kg/m2 for women) [14], whereas the IWGS cutoff reference values are 7.23 kg/m2 for men and 5.67 kg/m2 for women [3]. In contrast, the FNIH sarcopenia project uses appendicular lean mass adjusted for BMI for cutoff values (<0.789 for men and <0.512 for women) [15], and the EWGSOP does not specify cutoff values.
Abdominal computed tomography (CT) is an accurate, practical approach to quantifying whole-body and regional skeletal muscle mass [16]. Cross-sectional imaging using CT is the preferred method for analyzing muscle mass in cancer patients, because CT is performed clinically for staging and diagnostic purposes [17, 18]. Core muscle size, measured as the total psoas cross-sectional area (TPA) at the third or fourth lumbar vertebra, is a precise indicator of total skeletal muscle mass [19]. The skeletal muscle index is calculated as the TPA divided by height squared and is associated with the severity of dysphagia in cancer patients [18]. Preoperative psoas muscle mass on the contralateral side is associated with postoperative gait speed following total hip arthroplasty for osteoarthritis [20]. The cutoff value when assessing the risk for the development of liver failure in Japanese cancer patients was defined as <5.67 cm2/m2 in males and <3.95 cm2/m2 in females [21].
7.2.2.2 Muscle Strength
Muscle strength is mainly assessed by grip strength; it is the default evaluation given the low cost, availability, and ease of use of this equipment during evaluations. The cutoff values for grip strength are as follows: EWGSOP, <30 kg for men and <20 kg for women [1]; AWGS, <26 kg for men and <18 kg for women [14]; and FNIH sarcopenia project, <26 kg for men and <16 kg for women [15]. The IWGS diagnostic criteria for sarcopenia do not include muscle strength assessment [3].
Knee extension is another method used to assess muscle strength; assessed in relation to body weight, it correlates with physical function [22]. A threshold ratio of knee extension strength/body weight between 2.78 kPa/kg and 2.86 kPa/kg is considered a potential cutoff value by which to identify women presenting with greater functional impairments [22]. Knee extension strength was determined to be a better predictor of functional performance than was handgrip strength, but only among residents in assisted living facilities [23].
7.2.2.3 Physical Performance
Physical performance is primarily assessed by usual gait speed. The EWGSOP and the AWGS cutoff values for usual gait speed are <0.8 m/s [1, 14], whereas the IWGS cutoff value is <1 m/s [3]. In the FNIH sarcopenia project, physical performance assessment is not included in the diagnostic criteria for sarcopenia [15]. The Short Physical Performance Battery that includes gait speed, ability and time to rise from a chair five times, static balance tests, the timed up and go test that measures the time to rise from a chair and walk a short distance, and the 6-min walk test is another method to assess physical performance [24].
7.2.3 Causes
The EWGSOP has categorized sarcopenia into primary or age-related sarcopenia and secondary sarcopenia that is activity, nutrition, or disease related [1].
Activity-related sarcopenia can result from prolonged bed rest, a sedentary lifestyle, and/or deconditioning, for example, in low- or zero-gravity conditions. Nutrition-related sarcopenia can result from the inadequate dietary intake of energy and/or protein, and it may also be associated with either malabsorption or gastrointestinal disorders. Disease-related sarcopenia can be associated with advanced organ failure (heart, lung, liver, kidney, and brain), inflammatory disease, malignancy, or endocrine disease. However, the etiology of sarcopenia in older people is often multifactorial so that it may not be possible to precisely characterize strictly primary or secondary sarcopenia in certain individuals [1]. For example, older patients with aspiration pneumonia tend to be prescribed nil by mouth and bed rest during treatment, and peripheral parenteral nutrition is usually administered during fasting periods of 1–2 weeks. Under such circumstances, older patients with aspiration pneumonia can simultaneously experience age-, activity-, disease-, and nutrition-related sarcopenia (Table 7.1).
Table 7.1
Causes of sarcopenia
Causes | Examples |
---|---|
Age-related sarcopenia | No other cause evident except aging |
Activity-related sarcopenia | Bed rest, a sedentary lifestyle, and deconditioning or zero-gravity conditions |
Hospital-associated deconditioning | |
Unnecessary non-eating and bed rest sarcopenia in hospitals (iatrogenic sarcopenia) | |
Nutrition-related sarcopenia | Inadequate dietary intake of energy and/or protein |
Inappropriate nutrition management in hospitals (iatrogenic sarcopenia) | |
Disease-related sarcopenia | Advanced organ failure, inflammatory disease, malignancy, or endocrine disease |
Invasion, cachexia, neuromuscular disorders |
7.2.3.1 Age-Related Sarcopenia
The etiology of age-related sarcopenia can be multifactorial in that it can include decreased activity (bed rest), low protein intake, chronic inflammation, increased oxidative stress, lipotoxicity, reduced neuronal stimulation, hormonal changes, increased myostatin concentrations, and/or decreased blood capillary flow [25]. The ubiquitin-proteasome system (atrogin-1 and MuRF-1), which is important in protein degradation in acute muscle atrophy, seems not to be implicated in age-related sarcopenia [26]. In contrast, the dysfunction of autophagy-dependent signaling seems to be profoundly involved in age-related sarcopenia [27]. Sarcopenic conditions appear to be characterized by a marked defect of autophagy signaling, because p62/SQSTM1, but not LC3, accumulates in the sarcopenic muscle of mice [28]. It remains to be elucidated whether the myostatin-Smad pathway is fundamental to the development of sarcopenia [27].
7.2.3.2 Activity-Related Sarcopenia
Activity-related sarcopenia can result from prolonged bed rest, a sedentary lifestyle, and/or deconditioning or zero-gravity conditions [1]. For this type of sarcopenia, the loss of skeletal muscle mass and strength occurs at an approximate rate of 0.5% (range, 0.3–4.2%) total muscle mass per day [29]. Prolonged disuse (>10 days) can result in a decline in basal and postprandial rates of muscle protein synthesis, without an apparent change in muscle protein breakdown [29].
Hospital-associated deconditioning is characterized by a functional decline that occurs during acute hospitalization due to illness or injury and is unrelated to a specific neurological or orthopedic insult [6]. Hospital-associated deconditioning includes activity-related sarcopenia. In our previous cohort study [30, 31], 88–91% of patients with hospital-associated deconditioning were malnourished, and the decline in nutritional status resulted in a decline in the rehabilitation outcome.
7.2.3.3 Nutrition-Related Sarcopenia
Nutrition-related sarcopenia results from inadequate dietary intake of energy and/or protein and can be associated with either malabsorption or gastrointestinal disorders [1]. Adult malnutrition is classified as malnutrition in the context of acute illness or injury or invasion, chronic illness or cachexia, and/or social or environmental circumstances that can lead to starvation [32]. Nutrition-related sarcopenia includes starvation, and disease-related sarcopenia includes invasive injury and cachexia.
Older patients with hospital-associated deconditioning can be at risk for an inadequate energy intake that does not meet their basal energy expenditure. A total of 75 (44%) out of 169 patients fell into this category based on one report [31]. The energy intake of older patients with hospital-associated deconditioning seems to often be suboptimal, resulting in nutrition-related sarcopenia. Nutrition-related sarcopenia causes hepatic glycogen depletion. Glycogen is stored only in the liver and muscles in humans. Muscle glycogen is stored as a readily available fuel source for muscle tissue; it is not released into the blood as a source of glucose following glycogenolysis. Therefore, glucose is synthesized from glycogenic amino acids derived via skeletal muscle catabolism in nutrition-related sarcopenia. A chronic negative energy and/or a protein imbalance result in nutrition-related sarcopenia.
7.2.3.4 Disease-Related Sarcopenia
Disease-related sarcopenia is usually associated with advanced organ failure (heart, lung, liver, kidney, and brain), inflammatory disease, malignancy, and/or endocrine disease [1]. It is characterized by invasion, cachexia, and neuromuscular disorders. An intensive care unit-acquired weakness [33] can be classified as disease-related sarcopenia. Acute inflammatory crises triggered by events such as sepsis, pulmonary decompensation, emergency surgery, and/or trauma can result in profound muscle wasting via mechanisms of action that may include tumor necrosis factor-α-mediated catabolism, mitochondrial dysfunction, and/or a reduction of muscle protein synthesis [34].
Phases of acute inflammation or invasion can be classified as catabolic and anabolic. In a catabolic phase, both muscle and adipose tissue are degraded by proinflammatory cytokines. In an anabolic phase, both muscle and adipose tissue can be synthesized and increased by an appropriate nutritional management and exercise. Clinically, a catabolic phase features a muscle that is more degraded than adipose tissue. In patients with a critical illness, the loss of muscle mass can reach 1 kg/day due to conditions causing severe inflammatory responses [35]. Disease-related sarcopenia can be further complicated by the other causes of secondary sarcopenia outlined previously that can compound the sarcopenic effect in the face of hospital-associated deconditioning.
7.2.3.5 Complications of All Causes of Sarcopenia
Older patients with a hip fracture and hospital-associated deconditioning can complicate all causes of sarcopenia [6, 36]. Activities of daily living are independent in frail older people with only age-related sarcopenia. However, the functional reserve in frail individuals is considered limited. For example, if they develop a hip fracture, their skeletal muscle mass can rapidly deteriorate as the other secondary causes of sarcopenia come into play.
Most patients with a hip fracture undergo surgery, and during the perioperative period, they tend to be prescribed bed rest and fasting. In acute hip fracture inpatients, the total 24-h energy and protein intakes before a multidisciplinary nutritional care intervention were 707 kcal and 33.8 g, respectively [37]. Collectively, extended bed rest, poor nutritional support, and the invasive nature of a surgery with its inflammatory sequelae can contribute to the type of skeletal muscle catabolism indicative of secondary sarcopenia (Fig. 7.2).


Fig. 7.2
Mechanism of sarcopenia with disability in frail older people with hip fracture. Frail older people with hip fracture become sarcopenia with disability because of extended bedridden, catabolism due to fracture and surgery, and poor nutritional support. They can complicate activity-, nutrition-, disease-related sarcopenia
7.2.4 Treatment for Sarcopenia
The treatment for sarcopenia can differ depending on its primary and/or secondary classification and its etiology (Table 7.2).
Table 7.2
Sarcopenia treatment
Causes | Treatment |
---|---|
Age-related sarcopenia | Resistance training |
Protein and amino acid supplementation? | |
Pharmaceutical therapies? | |
Activity-related sarcopenia (iatrogenic sarcopenia due to unnecessary non-eating and bed rest) | Avoid bed rest, sedentary lifestyle |
Promote early activity, mobilization, exercise, rehabilitation | |
Combination of early rehabilitation and nutritional intervention | |
Nutrition-related sarcopenia (iatrogenic sarcopenia due to inappropriate nutrition management) | Keep positive energy and protein balance |
Aggressive nutrition care management considering daily energy accumulation | |
Light load activity and exercise | |
Disease-related sarcopenia | Treat and prevent diseases |
In catabolic phase, modest (15–30 kcal/kg/day) nutrition care management, light load activity, and exercise | |
Nonsmoking |
7.2.4.1 Age-Related Sarcopenia
Resistance training has been shown to be the most effective intervention when striving to combat age-related sarcopenia, because it induces skeletal muscle hypertrophy which can enhance muscle strength [4], even in older people over 75 years [38]. In the Cochrane Database of Systematic Reviews, progressive resistance strength training was also reported as an effective intervention for improving physical functioning in older people by increasing muscle strength and gait speed[39].
Protein and amino acid supplementation can contribute to counteracting sarcopenia, because low protein intake is known to be associated with declining muscle mass in older adults [40]. In a systematic review, nutritional supplementation was reported to improve both muscle mass and muscle strength in older people with sarcopenia [41]. A meta-analysis showed that protein supplementation can also increase muscle mass and strength gains during prolonged resistance-type exercise training in sarcopenia [42]. However, another meta-analysis reported that amino acid/protein supplements did not increase lean body mass and muscle strength significantly in older people [43]. Furthermore, in a recent meta-analysis, combining protein supplementation with resistance training did not seem to increase muscle mass or strength [44]. Considering these results, whole-protein supplementation failed to show a consistent effect on muscle mass, strength, or function [45].
7.2.4.2 Activity-Related Sarcopenia
Increasing physical activity and avoiding prolonged and unnecessary bed rest or a sedentary lifestyle are good general strategies for mitigating activity-related sarcopenia, and if needed, rehabilitation may be advised. Early rehabilitation may be safe and feasible for critically ill patients in the intensive care unit when medically prescribed [46, 47]. Therapies to combat the loss of muscle mass have focused largely on strategies using resistance exercises and/or nutrition, but with mixed success [48]. Iatrogenic sarcopenia due to unnecessary fasting and bed rest in hospitalized older people should be avoided.
In a recent randomized controlled trial, an early nutritional intervention using nutritional supplements (600 kcal, 20 g/d protein added to a standard diet) together with early rehabilitation preserved the muscle mass and independence of ill, older patients hospitalized for acute diseases [49]. A combination of early rehabilitation and nutritional intervention seemed to be a highly effective method to treat and ameliorate activity-related sarcopenia, because many patients with hospital-associated deconditioning can be malnourished [30, 31].
7.2.4.3 Nutrition-Related Sarcopenia
Recommendations for the treatment of nutrition-related sarcopenia focus on the maintenance of a positive energy and protein balance. Daily energy requirements in cases of nutrition-related sarcopenia can be calculated as daily energy expenditure plus daily energy accumulation (200–750 kcal/day). The excess energy required to gain 1 kg of body weight was between 8856 and 22,620 kcal/kg in malnourished nursing home patients [50]. Gaining weight is more difficult in older compared with younger people. Therefore, aggressive nutrition care management is often necessary to improve nutritional status.
Prevention of nutrition-related sarcopenia is important, especially in some older inpatients with hospital-associated deconditioning who are prescribed peripheral parenteral nutrition that furnishes less than 300 kcal/day in the absence of an oral caloric intake and enteral nutrition [51]. Identification of iatrogenic sarcopenia in older hospitalized patients due to nutritional management issues should be addressed as soon as possible to determine if additional nutritional support can be provided.
Resistance training to improve muscle mass should be avoided if energy and protein balance is negative, because this type of exercise, as well as endurance training, exacerbates the negative energy balance and can worsen nutrition-related sarcopenia. Resistance training to improve muscle mass should be performed only if energy and protein are balanced or positive. However, prolonged bed rest should be avoided if energy and protein balance is negative, because inactivity enhances catabolic responses to calorie restriction [52]. Light activity and exercise should be performed to prevent activity-related sarcopenia.
7.2.4.4 Disease-Related Sarcopenia
The most important factor in disease-related sarcopenia is treatment of the disease. Appropriate treatment of disease-related sarcopenia is different in the catabolic phase compared to the anabolic phase. In a catabolic state, muscle degradation and endogenous energy production are usually increased, and disease-related sarcopenia can develop rapidly. Nutrition care management under these circumstances should be modest (15–30 kcal/kg/day), and daily energy expenditure beyond energy intake should be curtailed because of increasing endogenous energy production. Light load activity and exercise should be performed to prevent activity-related sarcopenia. Anabolic phase is the state during which muscle mass and adipose tissue can be gained as a result of physical exercise and activity and appropriate nutrition care management. Nutrition care management in the anabolic phase should be tailored to the patient’s daily energy expenditure plus daily energy accumulation (200–750 kcal/day) to improve muscle mass and strength. Resistance training to improve muscle mass is encouraged in anabolic phase.
In critically ill patients, neuromuscular electrical stimulation added to standard treatment has been proved to be more effective than the standard treatment alone in the prevention of skeletal muscle weakness [53]. In the Cochrane Database of Systematic Reviews, it has been shown that no harm has been associated with the following related to muscle disease: moderate-intensity strength training in myotonic dystrophy and facioscapulohumeral muscular dystrophy and aerobic exercise training in dermatomyositis, polymyositis, and myotonic dystrophy type I [54]. However, there is insufficient evidence to conclude that they offer any benefit. In mitochondrial myopathy, aerobic exercise combined with strength training appeared to be safe and may be effective at increasing submaximal endurance capacity [54].
Smoking cessation can be recommended for the prevention and treatment of sarcopenia. Cigarette smoking may contribute to the development of sarcopenia according to a meta-analysis [55].
7.3 Cachexia
7.3.1 Definition and Epidemiology
Cachexia, as defined at the Washington Cachexia Consensus Conference in 2006 [2], is a complex metabolic syndrome associated with an underlying illness that is characterized by a loss of muscle with or without a loss of fat mass. The prominent clinical feature of cachexia is weight loss (corrected for fluid retention) in adults or growth failure (excluding endocrine disorders) in children. Anorexia, inflammation, insulin resistance, and increased muscle protein breakdown are frequently associated with wasting disease. Wasting disease, which is distinct from starvation, age-related loss of muscle mass, primary depression, malabsorption, and hyperthyroidism, is associated with increased morbidity [2]. This definition can be applied to all causative diseases of cachexia.
The Special Interest Groups (SIGs), “cachexia-anorexia in chronic wasting diseases” and “nutrition in geriatrics” in the European Society for Clinical Nutrition and Metabolism (ESPEN), proposed that cachexia should be classified in accordance with its stage as either precachexia or cachexia in 2010 [56]. In 2011, cancer-induced cachexia was defined by international consensus as a multifactorial syndrome characterized by an ongoing loss of skeletal muscle mass (with or without loss of fat mass) that cannot be fully reversed by conventional nutritional support and leads to progressive functional impairment [17]. The pathophysiology of cancer cachexia is characterized by a negative protein and energy balance driven by a variable combination of reduced food intake and abnormal metabolism [17].
Cachexia is commonly referred to as protein-energy wasting in the context of renal diseases. The International Society of Renal Nutrition and Metabolism (ISRNM) defines protein-energy wasting as the state of decreased body stores of protein and energy fuels (i.e., body protein and fat masses) [57]. Cachexia is a severe form of protein-energy wasting that occurs relatively infrequently in kidney diseases [57].
The prevalence of cachexia ranges from 5 to 15% in end-stage chronic heart failure to 50–80% in advanced cancer [58]. The overall prevalence of cachexia is approximately 1% of the patient population; it affects an estimated nine million people and mortality rates of patients with cachexia range from 10 to 15% per year in chronic obstructive pulmonary disease to 20–30% per year in chronic heart failure and chronic renal disease and up to 80% in cancer patients [58].
In older inpatients with hospital-associated deconditioning, 88% were malnourished and 30% had precachexia [31]. Among them, nutritional status, low concentration of serum albumin, and precachexia were associated with poor rehabilitation outcomes as assessed using the Barthel index score at discharge [31]. Cachexia may be common in older patients who need rehabilitation, and it can adversely affect rehabilitation outcomes. Therefore, cachexia assessment is imperative in patients with wasting disorders.
7.3.2 Diagnostic Criteria
Several diagnostic criteria for cachexia have been proposed in consensus papers [2, 17, 56]. At the Washington Cachexia Consensus Conference, a weight loss of at least 5% of body weight within a period not exceeding 12 months, without the presence of edema or with a BMI <20 kg/m2 in the presence of wasting diseases, was necessary for cachexia diagnosis. Furthermore, three of the following five criteria are necessary for a definitive diagnosis of cachexia: decreased muscle strength, fatigue, anorexia, low muscle (fat-free) mass, and biochemical abnormalities characteristic of inflammation, anemia, or hypoalbuminemia verified by a blood test [2]. This diagnosis can be applied to all causative diseases of cachexia.
Cachexia can be classified into three stages that include precachexia, cachexia, and refractory cachexia. The ESPEN SIGs indicate a diagnosis of precachexia has the following criteria: an underlying chronic disease, an unintentional weight loss ≤5% of usual body weight during the last 6 months, a chronic or recurrent systemic inflammatory response, and anorexia or anorexia-related symptoms [56]. This precachexia diagnosis is applicable to all wasting disorders.
There is international consensus that the diagnosis of cancer-induced cachexia is different depending on the stage. Precachexia is diagnosed by a weight loss <5% over the past 6 months [17]. Cancer-induced cachexia is diagnosed by weight loss >5% over the past 6 months in the absence of energy intake reduction, or a BMI <20 kg/m2 and weight loss >2%, or an appendicular skeletal muscle index consistent with sarcopenia (i.e., males, <7.26 kg/m2; females, <5.45 kg/m2) and any degree of weight loss >2% [17]. A diagnosis of refractory cancer cachexia includes the following criteria: fulfillment of the criteria by which cachexia is defined, a prognosis of <3 months, an Eastern Cooperative Oncology Group performance status of 3 or 4, being unresponsive to anticancer therapy, ongoing catabolism at an increasing rate, and a status of unsuitable for artificial nutritional support [17]. These diagnoses can only be applied to cancer patients.
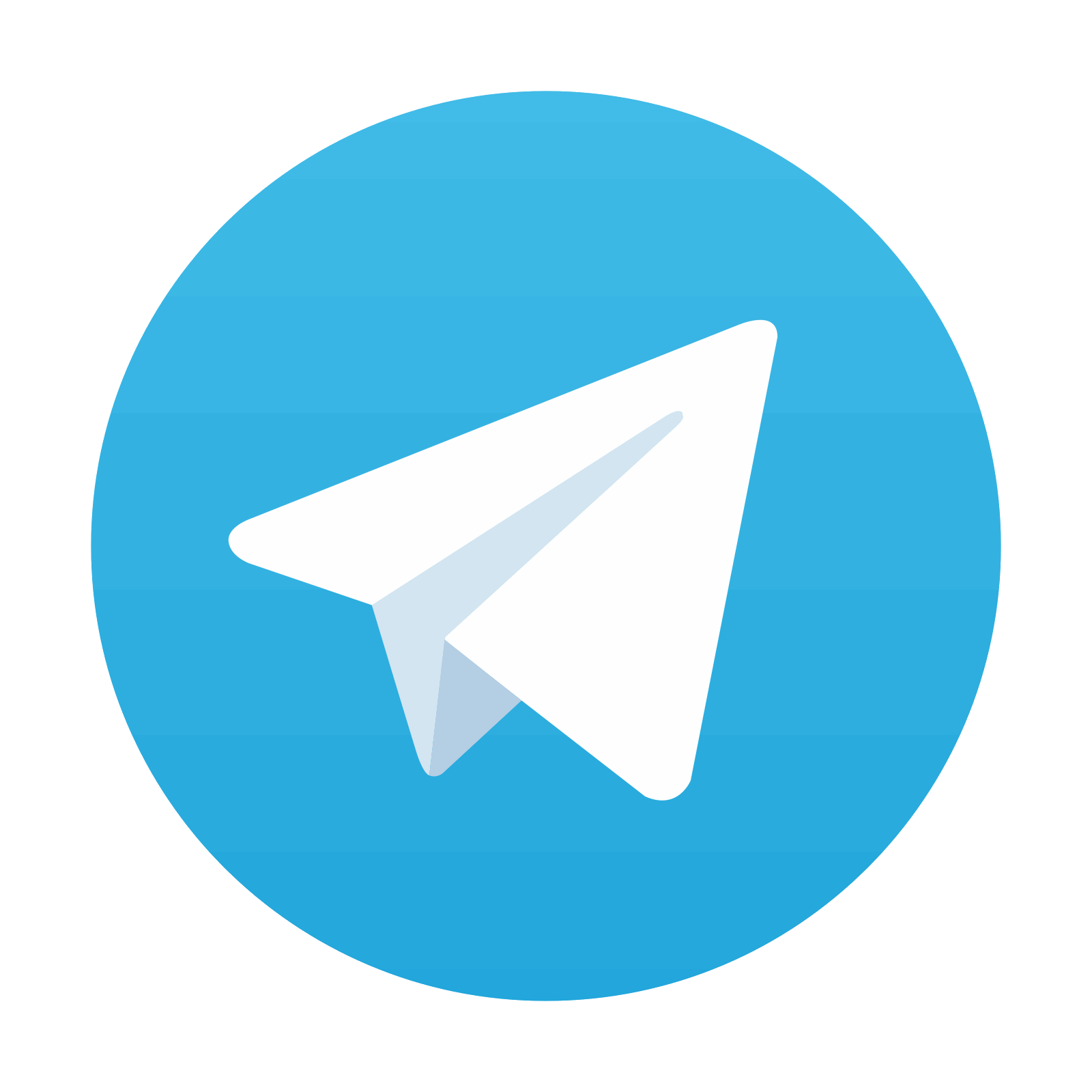
Stay updated, free articles. Join our Telegram channel

Full access? Get Clinical Tree
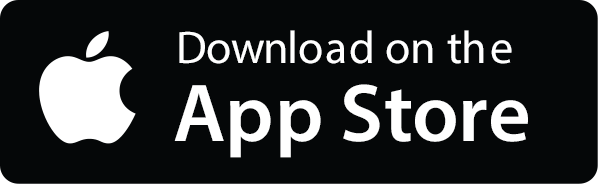
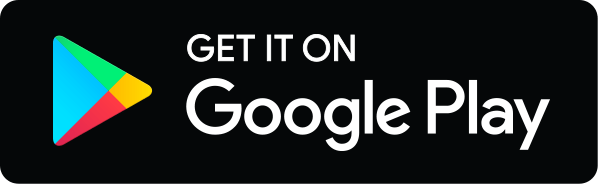