Classification system for posterior C1 ring anomalies. (a) Failure of posterior midline fusion of the two hemiarches. (b) Unilateral clefts. A defect is present in one of the two arms of the posterior arch ranging from a small gap to a complete absence of the half arch including the posterior tubercle. (c) Bilateral clefts. A bony defect is present in the lateral aspect of the arch bilaterally with preservation of the most dorsal part of the arch. (d) Absence of the posterior arch with persistent posterior tubercle. (e) Absence of the entire arch including posterior tubercle. The entire posterior arch is missing, but occasionally one or both roots of the arch near the lateral masses are preserved. (From: Currarino et al. [7]. Reprinted with permission from American Society of Neuroradiology)
Following an injury to the cervical spine, radiographic evidence of C1–C2 hypermobility with an anterior dens interval of 4 mm or greater and the presence of cervical myelopathy in the patient’s clinical history or physical examination are absolute contraindications for return to play (RTP) [11]. Considering the high potential for neurological injury and the potential jeopardy to the upper cervical spinal cord, it is highly suggested that unstable anomalies of the C1 ring be an absolute contraindication for return to play. Isolated Type A defects are inherently stable; therefore athletes with this particular defect would be allowed to participate in sporting activities without restriction. Stability should still be documented in these patients with dynamic imaging. Additionally, any neurologic symptom or deficit would preclude athletic participation.
Os Odontoideum
Os odontoideum is an oval or round-shaped ossicle of variable size with a smooth cortical border which may be located in the position of the odontoid process (orthotopic) or near the base of the occipital bone in the region of the foramen magnum (dystopic), without bony connection to the body of the axis [12]. The etiology of os odontoideum has been mainly attributed to embryological (congenital) or traumatic (acquired) causes [13–15]. Os odontoideum is considered to be a segmental anomaly resulting from a failure of fusion between the dens and the body of the axis. The congenital theory has two potential explanations. Firstly, the incomplete ossification of the intervertebral disc that separates the odontoid process from the base of the axis has been thought to be the reason for the formation of the residual ossicle. Secondly, it is felt that there is a familial component with several cases of os odontoideum within a family group having been reported. Wang et al. reported a familial example of os odontoideum with three occurrences [13].
However, current evidence now suggest that there is a traumatic etiology in the majority of cases [16, 17]. This hypothesis is supported by a history of trauma being present in several patients found to have radiographic evidence of an os odontoideum. There are also a large number of patients where it is difficult to draw a clear connection to a traumatic cause due to there being a delay in the clinical diagnosis and the traumatic event. At the time of injury, it is believed that the alar ligaments contract resulting in a distraction of the fractured odontoid fragment away from the base of the axis. As the fractured ossicle migrates rostrally toward the occiput and the fracture remodels, the smooth circumferential cortical margins remain. This remodeling process in an adolescent or juvenile patient makes os odontoideum different from a geriatric Type II odontoid fracture. Babak et al. agree that the os odontoideum is likely caused by a traumatic event [16]. They felt that a traumatic cause of os odontoideum is likely due to the gap between the os odontoideum and the remnant of the odontoid process characteristically appearing above the level of the superior facets of the axis. According to the congenital theory , the failure of fusion should be observed at the level of the neurocentral synchondrosis.
Plain radiographs are used to diagnose os odontoideum and to assess C1-C2 stability. The sensitivity and specificity of plain radiographs for this diagnosis remain to be reported. Radiographic evaluation should include open mouth odontoid, AP, and lateral views of the cervical spine in flexion and extension. Flexion and extension radiographs can add valuable information for the diagnosis; however neck pain can result in limited excursion between views and potentially cloud the diagnosis of instability (false negative). Caution is advised when obtaining flexion and extension views in an athlete complaining of neck pain or with neurological deficits on physical exam as pathologic motion can result in potential neurological consequences.
Acquisition of a CT scan and/or an MRI of the craniocervical junction is at the discretion of the healthcare provider and can be considered if plain radiographic evaluation is felt to be inadequate [18]. CT scans and MRI scans are useful for the more detailed evaluation of osseous abnormalities, vertebral arteritis, and spinal cord compression [16]. Hughes et al. suggested that dynamic MRI may have an advantage in visualizing the instability of the joints and surrounding soft tissues [19]. The os odontoideum should be clearly differentiated from ossiculum terminale, which refers to the nonunion of the apex at the secondary ossification center. Ossiculum terminale is rarely associated with atlantoaxial instability and usually does not require surgical treatment [16].
The presence of os odontoideum may be completely asymptomatic or present with isolated neck pain or potentially myelopathy. For the athlete with an asymptomatic os odontoideum, it is commonly an incidental finding with the athlete being completely neurologically intact; however, flexion-extension radiographs may show evidence of atlantoaxial instability. For the symptomatic patient, neck pain, especially occipitocervical pain, is a common presentation. Dai et al. reported that the most common neurologic finding was myelopathy with associated radicular symptoms (22 patients) [20].
The initial presentation of os odontoideum can be insidious in nature. Os odontoideum with atlantoaxial dissociation may develop acutely or chronically and cause compression of the cervicomedullary junction, vascular compromise, and cervical pain if left untreated [21]. Untreated, this may cause symptoms including transient myelopathy to tetraplegia, central cord syndrome, and even death. Clinically, patients with os odontoideum experience severe neck pain and myelopathy resulting from craniocervical instability and spinal cord compression. A close correlation between the extent of hyperintensity in T2-weighted images and the severity of neurological deficits, either on admission or at the last follow-up, has been reported. Zhang et al. suggested that a prophylactic surgery should be considered for patients at risk of developing myelopathy and to avoid the associated neurological deterioration [21]. A level III recommendation raised by Curtis et al. also suggests that clinical and radiographic surveillance or posterior C1-C2 fixation and fusion is recommended for patients with asymptomatic os odontoideum [18]. For the symptomatic os odontoideum patient, posterior fixation and fusion is recommended [18].
There are reports of major neurologic complications after minor trauma as the initial presentation of patients with previously undiagnosed os odontoideum. In one report, a 13-year-old girl with an os odontoideum, a bifid atlas, and a cyst around the odontoid tip, with consequent severe atlantoaxial and craniovertebral instability, developed quadriplegia after a minor injury to the head [10]. Tory et al. also report a case with quadriplegia after a minor trauma and recommended that an odontoid anomaly be considered an absolute contraindication for sporting activity [22].

An algorithm for the management of a patient with os odontoideum
Klippel-Feil Syndrome
First described in 1912 by Maurice Klippel and Andre Feil in France, Klippel-Feil syndrome (KFS) is commonly associated with a low posterior hairline, a short neck, and limited cervical range of motion [24–26]. To be diagnosed with KFS, the failure of segmentation of cervical motion segments , noted as congenitally fused vertebrae, must be present [24, 27]. When evaluating the athlete for the presence of KFS, the presence of the classically described clinical triad cannot be relied upon. In a retrospective review of 31 adolescent patients (12 male, 19 female), with an average age of 9.7 years at initial evaluation, Samartzis et al. found that limited cervical range of motion was the most common finding present in patients with KFS [28]. In 35.5% of the patients evaluated by Samartzis, none of the expected clinical findings were present, while one of the expected findings was present in 38.7%, two in 16.2%, and three in 9.7% of the cohort [28]. While the classically taught clinical triad represents the major clinical findings of KFS, there are multiple other associated findings that are important to consider when evaluating an athlete for sport eligibility. Major anomalies associated with KFS syndrome are small stature, thoracic kyphoscoliosis, lumbar scoliosis, Sprengel deformity, restricted mouth opening, and bilateral sensorineural hearing loss [29–32]. Minor associations are mild face asymmetry, high-arched palate, rhino scoliosis, high nasal bridge, renal agenesis, persistent trigeminal artery, thoracic bifurcation of the common carotid artery, aortic coarctation, anomalous course of the subclavian artery, and agenesis of the internal carotid artery [25, 27, 29, 32].
Klippel-Feil syndrome can be classified into three subtypes: Type I is defined as a single congenitally fused cervical segment; Type II is composed of multiple noncontiguous, congenitally fused segments; and Type III is multiple contiguous, congenitally fused cervical segments [24, 27]. The most commonly identified subtype is the Type II fusion with the most commonly fused segment being C2-C3, followed by C5-C6 [33]. The age at which complete fusion of the affected segments (C2-T1) occurs is 77.8% at ≥10 years of age, 87.5% at ≥15 years of age, 91.7% at ≥16 years of age, 95.7% at ≥17 years of age, 86.5% in skeletally mature patients, and 100% in adults [33]. Ultimately, in a review of 28 patients, Samartzis et al. found 36% of KFS patients to have axial symptoms, predominantly associated with Type I patients [24]. Type II and Type III patients predominantly presented with radiculopathy and myelopathy [24].
The impact of KFS on the diameter of the cervical spinal canal has been evaluated by Samartzis et al. in a prospective evaluation of 29 patients with KFS [34]. Congenital fusion of the vertebral bodies within KFS may also result in an alteration of appositional bone growth of the affected vertebral bodies. In this study, it was noted that not only were the diameters of the fused vertebral body segments affected but also the diameters of the cranial and caudal vertebral bodies. This results in an increased space available for the cord [34]. Therefore, the likelihood of congenital cervical stenosis associated with the presence of KFS is decreased in the pediatric and adolescent athlete. As the age of the athlete increases, it may be necessary to begin considering the potential presence of stenosis due to degenerative changes at the levels adjacent to the fused segments.
Patients with KFS have been noted to be at increased risk for neurologic injury from minor trauma [27, 35]. Vaidyanathan et al. reported on a 51 years of age patient that suffered an incomplete tetraplegia following a slip and fall to the ground where the patient struck his head [27]. Of note, the patient had a similar incident approximately 15 years prior which resulted in a transient numbness and paresis of the lower extremities. This patient was found to have congenital fusions of C2-C3 and C4-C5 (Type II KFS) [27]. The authors of this report recommend that patients with KFS “should be made aware of the increased risk of sustaining transient neurologic deterioration after minor trauma if there is associated radiographic evidence of spinal stenosis” [27]. Pizzutillo et al. in a study of 111 patients with KFS noted that those with hypermobility of the upper cervical spine were at increased risk of neurologic sequelae when compared to a similar control population [35]. If an injury resulting in airway compromise on the track, field, or court was to occur, it is important for the healthcare provider to know that the shortened neck, decreased cervical range of motion, or kyphoscoliosis present in some patients with KFS can make emergent airway management difficult [36].
While the clinical and radiographic presentations of Klippel-Feil syndrome are quite varied, recommendations regarding athletic participation of the athlete with subaxial Klippel-Feil can be based on basic principles. A Klippel-Feil patient with noted hypermobility (greater than 3.5 mm of horizontal displacement or 11 degrees rotation difference to the adjacent level on flexion-extension radiographs) of the cervical spine should be restricted from participating in contact or other high-risk sports [23]. The patient with cervical stenosis in the setting of Klippel-Feil syndrome should be advised that they are at increased risk of transient neurologic injury from minor trauma, but this should not necessarily preclude athletic participation. In general, the Type I KFS athlete should be treated as any other athlete; neurologic symptoms and stenosis should guide sport participation. In our opinion, Type II and type III KFS patients should be contraindicated from participating in contact sports.
Assimilation of the Atlas and Klippel-Feil Syndrome
The assimilation of the atlas is caused by the failure of segmentation between the fourth occipital sclerotome and the first spinal sclerotome. It may present with focal, segmental, unilateral, or bilateral fusion. It is usually observed in patients with Klippel-Feil syndrome. Ultimately, this deformity may lead to atlantoaxial instability and basilar invagination [9].
An occipital-C1 assimilation is a relative contraindication to return to participation in contact sports. The patient and family should understand that recurrent injury is a possibility and the degree of risk is uncertain. When C1 assimilation is combined with Klippel-Feil syndrome, basilar invagination, or atlantoaxial instability, it is an absolute contraindication for return to play [11].
Congenital Cervical Stenosis
Congenital cervical stenosis (CCS) is a complex multifaceted topic with implications for all athletes, especially those who participate in contact sports. Cervical stenosis can be congenital, degenerative, or acquired from accumulated trauma or a combination of each. For a detailed understanding of these topics and related clinical issues related to athletes, the reader is encouraged to refer to the appropriate chapters of this text. It is important to have a thorough understanding of CCS so that more objective return-to-play recommendations can then be made.
A significant number of athletes have congenital cervical stenosis. Some are diagnosed after the onset of neurologic symptoms, others following the onset of pain, and some are discovered incidentally during the evaluation of a brachial plexus neuropraxia. Over the last few decades, with the accessibility of MRI scans, the topic of spinal cord signal change and functional stenosis (amount of cerebrospinal fluid around the cord) have become increasingly studied [37–39], because not only are the bony dimensions of the canal important but also the role of the discs, ligaments, and osteophytes in creating extrinsic cord compression can be more easily and precisely evaluated. Additionally, the cord diameter [40] and cross-sectional area vary greatly [41].
There is not always a consensus of what is considered stenosis as there are numerous methods to measure this, and radiographic standards, both technically and anatomically, are not consistent. While there are varied definitions of cervical stenosis, most agree that with a static sagittal canal diameter >14 mm, the diagnosis is excluded. The Torg ratio (canal diameter-vertebral body diameter) has been used extensively, but with more advanced imaging, further evaluation can now be undertaken and the process further understood [37].
Bajwa evaluated 1066 American cadavers and defined congenital cervical stenosis as a geometric canal area 2 standard deviations below a standard measurement [42]. The values for CCS were defined at each vertebral level and ranged from 1.82 cm2 (C3/4) to 1.89 cm2 (C6/7). When this was correlated with sagittal canal diameter (SCD) and interpedicular distance (IPD), they concluded that values of SCD <13 mm and IPD <23 mm were strongly associated with the presence of CCS at all levels.
Attempting to predict the increased risk of potential neurologic sequelae in athletes with CCS has gained interest in the literature. Presciutti [43] determined that a space available for the cord (CSF surrounding the cord) of 5 mm in American football players resulted in a sensitivity of 80% and a negative likelihood ratio of 0.23 for potential neurologic sequelae. If the cutoff was decreased to 4.3 mm, the sensitivity is increased to 96%, and the negative likelihood ratio is increased to 13.25, respectively. Hence, a space available for the cord of 5 mm was determined to be a good test for screening athletes at risk, and a measurement of 4.3 mm added additional confidence as a confirmatory test.
Herzog et al. [44] radiographically evaluated asymptomatic professional football players to determine radiographic parameters in these athletes. They determined that the correlation coefficient was higher for CT scan than it was for MRI measurements. The diameter of the canal on plain radiographs in the subaxial spine, when corrected for magnification, ranged from 14.8 to 15.4 mm, with the cervical-6 level being the most capacious. These closely approximated a control group [45].
Nouri [39] studied cervical cord-canal mismatch . This accounts for both cord and canal size by measuring a spinal cord occupation ratio (SCOR) . When the SCOR was found to be ≥70% on midsagittal or ≥80% on axial imaging, a mismatch was identified, and the potential concern of an increased risk of spinal cord injury was determined to be present. The importance of this ratio is that canal size and cord size are both important factors in determining the risk of potential injury. A narrow canal as an independent factor may not be as predictive of increased risk of injury. Nouri found that the smallest average spinal canal diameter is at the cervical-5 to cervical-6 levels [39].
Cord size was found to vary independently of canal size in an MRI study by Kato et al. They determined that morphologically, the male spinal canal sagittal diameter was 11.2 mm and 9.5 mm at the cervical-5 mid-body and the cervical-5–6 disc level, respectively [41]. In reviewing similar studies, they noted that there were different findings of normal spinal parameter measurements and hypothesized that different MRI magnet strengths and different imaging modalities used (CT scans and plain radiographs) could potentially be the source of these observed differences. Hence, any individual imaging study must be taken in context, further complicating this topic. The cervical stenosis in this chapter lends additional information on diagnosis, management, and return-to-play.
Marfan Syndrome
Marfan syndrome is a relatively uncommon autosomal dominant disorder that results from a mutation of the fibrillin-1 gene. Musculoskeletal manifestations include scoliosis and ligamentous laxity [46]. Bony and ligamentous abnormalities of the cervical spine include increased atlantoaxial translation, basilar invagination, and focal kyphosis, but clinically symptomatic cases are rare [47]. Clinically symptomatic cases usually present with neck pain and may initially be managed with physical therapy, cervical traction, and non-operative management. Arthrodesis is an option for stabilizing the cervical spine should gross instability be present [48, 49]. For the subaxial cervical spine, kyphosis and subluxation can also be observed, and symptomatic cases may warrant arthrodesis [50, 51]. Preoperative evaluation and consequent care with intubation and positioning of these patients may be necessary.
Neck pain in the patient with Marfan syndrome should alert the physician to evaluate the patient for cervical instability. When a patient diagnosed with Marfan syndrome presents with a cervical spinal cord abnormality on MRI, asymptomatic ligamentous laxity (i.e., greater than 11 degrees of kyphotic deformity as compared with the cephalad or caudal vertebral functional spinal unit or more than 3.5 mm movement on lateral flexion-extension radiographs) or C1–C2 hypermobility is present with an anterior dens interval of ≥4 mm; it is an absolute contraindication to return to contact sports [11].
Basilar Invagination
Basilar invagination (BI) is a congenital abnormality of the craniovertebral junction in which the odontoid process prolapses into the foramen magnum. It is a radiographic finding with possibly significant clinical findings that can be the result of hypoplasia of the clivus, incomplete formation of the C1 ring with displacement of the C1 lateral masses, achondroplasia, or atlanto-occipital assimilation. Basilar invagination is often confused with basilar impression, platybasia, and cranial settling. Basilar impression results from the secondary acquired form of invagination, due to conditions such as rheumatoid arthritis (RA), tumor, Marfan syndrome, or other diseases. Platybasia is defined as an abnormal basilar angle when measured from the plane of the clivus to the plane of the anterior skull base and is often associated with BI. Cranial settling refers to a form of basilar impression caused by RA.
Basilar invagination is often associated with other craniovertebral abnormalities, including assimilation of the atlas, remnants of the occipital vertebrae, blocked vertebrae, short neck, or other vertebral anomalies. Between 25% and 35% of BI cases are also associated with a Chiari I malformation, syringohydromyelia, syringobulbia, and hydrocephalus [52]. Caetano et al. reported 66 cases of basilar invagination and showed that the most common clinical symptoms included weakness in the lower limbs (68%), unsteady gait (56%), and headache (53%) [53]. Goel et al. reported a surgical study on 190 BI patients in which 80 of the patients had no evidence of an associated Chiari I malformation. Among these 80 patients, the most common symptoms included weakness (100%), neck pain (59%), and posterior column dysfunction (39%). Physical exam findings included torticollis (69%), restricted neck movement (59%), low hairline (48%), webbed neck (47%), and short neck (41%). Trauma was a major factor that influenced the acute development of symptoms in these previously asymptomatic patients. However, in the second group of patients with associated Chiari malformations, symptoms progressed slowly without any antecedent trauma. Goel et al. postulated that the symptoms and signs in group 1 were related to brainstem compression by the odontoid process, whereas in group 2 they were related to the crowded neural structures at the foramen magnum. In group 2 patients with associated Chiari malformations, 44% are presented in the third decade of life, and the duration of symptoms was slowly progressive, and the most common symptoms included weakness (94%), paresthesia (79%), and posterior column and spinothalamic tract disturbance (56%). Localized findings are similar to those observed in the patients included in group 1 [54].

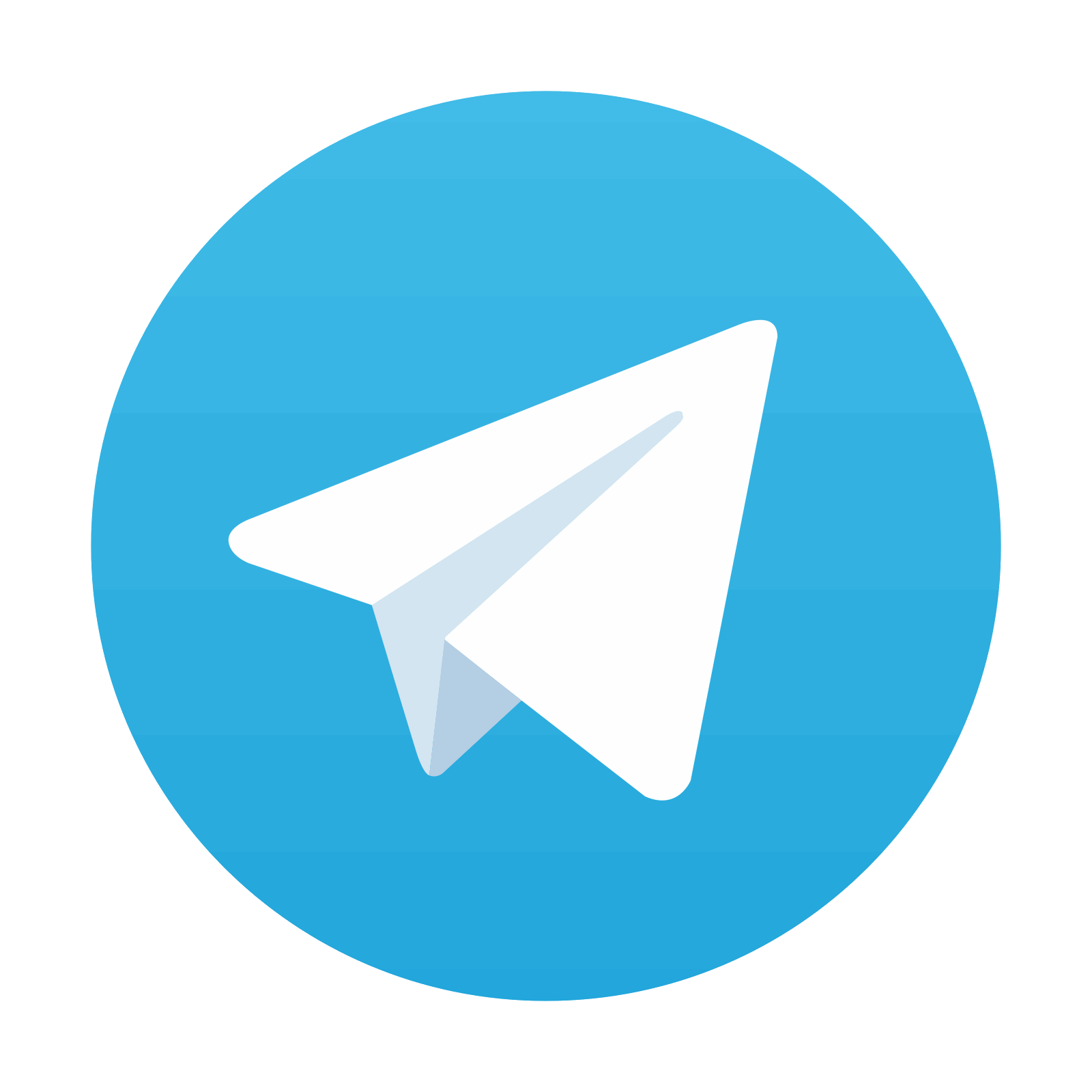
Stay updated, free articles. Join our Telegram channel

Full access? Get Clinical Tree
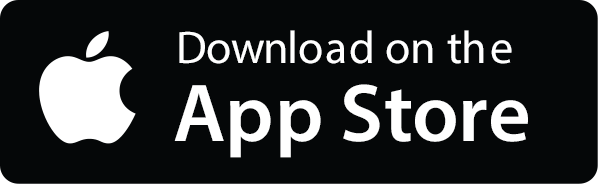
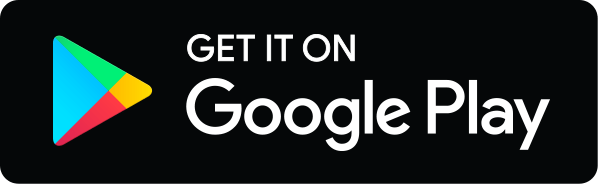