Pertinent anatomy of costovertebral articulations . Axial figure of costovertebral joint shows costocentral and costotransverse articulations, normal on the right and disrupted on the left. (From: O’Brien and Bui-Mansfield [41]. Reprinted with permission from Wolters Kluwer)
The true ribs are those that articulate directly, through their costal cartilage, with the sternum. The 8th, 9th, and 10th ribs are false ribs that articulate with the sternum indirectly, through the costal cartilage from other ribs. Ribs 11 and 12 are called “floating” ribs because they are shorter and do not articulate with the sternum at all, only with their corresponding vertebrae. The floating ribs have a single articular facet on the rib head, with no neck or tubercles.
The erector spinae muscles function to extend the trunk and provide support to the axial skeleton. They are located dorsal to the vertebral column and are innervated by the dorsal rami of spinal nerves. The spinalis is located most medial and connects spinous process to spinous process. The iliocostalis is most lateral and originates from the ilium and ribs, inserting on the ribs and transverse processes. In between the two, the longissimus links transverse process to transverse process.
The stability of the spine is often considered in the context of the three column spine theory, as popularized by Denis [4]. The anterior column includes the anterior longitudinal ligament and the anterior 2/3 of the vertebral body and annulus. The middle column includes the posterior longitudinal ligament and posterior 1/3 of the vertebral body and annulus. The posterior column includes the pedicles, lamina, facets, ligamentum flavum, spinous process, and posterior ligamentous complex. Instability is often dependent on the involvement of the middle column, as evidenced by widening of the interpedicular distance on the AP radiograph and/or loss of height of the posterior cortex of the vertebral body on the lateral radiograph. Furthermore, the additional stability provided by the rib cage and sternum has often been referred to as a “fourth column ” of spinal stability [5]. In fact, the sternum has been shown to contribute over 40% stability to thoracic spine range of motion [6].
Presentation
Athletes presenting with complaints in the thoracic region should be evaluated promptly. A senior athletic trainer is often responsible for on-the-field triage and making the initial diagnosis. Team physicians become involved when further testing is required or urgent medical attention is warranted. A comprehensive history should include characterization of the chief complaint (pain, stiffness, dysesthesia, weakness, etc.), timing (acute vs. subacute vs. chronic), associated symptoms (fever, chills, traumatic injuries, infections, dyspnea, chest pain), and prior treatments (activity modification, medications, physical therapy, injections, procedures). A corroborative history via trainer, coach, teammate, or parent is helpful, when possible.
Physical Exam


Neurologic examination recommended by the American Spinal Injury Association for each patient sustaining a spinal injury. (International Standards for Neurological Classification of SCI) (Copyright © 2011 American Spinal Injury Association. Reprinted with permission)
Imaging
Anteroposterior and lateral thoracic standing spine radiographs should be performed at the physician’s discretion if there is concern for spinal pathology. Chest radiograph should be performed if “anterior” pathologies are in the differential diagnosis, including rib fracture, pneumothorax, and costochondral separation [7]. Advanced imaging with computed tomography (CT) is used to further assess bony pathologies, while magnetic resonance imaging (MRI) is reserved for elucidation of neurologic, ligamentous, and soft tissue disorders.
Pathologies
Muscular and Ligamentous Injuries
Thoracic “myofascial” pathology may result from both acute trauma and with chronic, overuse mechanisms. High-energy acute injuries are caused by rotational or bending forces similar to whiplash injuries in the cervical spine. Pain and tenderness often present 12–24 h after injury secondary to the inflammatory cascade. The neurologic exam and radiographs are normal in purely myofascial injuries, and any signs of neurologic dysfunction should prompt further evaluation. An MRI is helpful in evaluating the neurologic and ligamentous elements of the thoracic spine, and CT can be useful in ruling out occult fracture.
Acute myofascial injuries are treated with conservative management, including hiatus from aggravating activities, physical therapy, and anti-inflammatory medication. Muscle relaxants and selective injections are often used as adjunctive therapies. Chronic overuse injuries from sustained, high-repetition activities can result in debilitating, long-lasting pain. The pull of the rhomboids, latissimus dorsi, and erector spinae at the T4–T7 junction is particularly susceptible to strains and stress fractures [1]. Physical therapy should focus on the kinematics of the thoracic spine, rib cage, and shoulder girdle. The therapist should use both passive and active modalities, if possible. Passive modalities do not rely on muscle activation and include hot-cold compress, ultrasound, and massage. These therapies may alleviate acute symptoms and facilitate transition to active therapy modalities focusing on postural mechanics, core strengthening, and trunk stabilization.
Fractures
Fractures to the thoracic spine are rare given the relative stability of this region of the spine. Nonetheless, myriad fracture morphologies are possible, ranging from minor injuries such as isolated transverse or spinous process fractures to major injuries such as unstable fracture/dislocations with high potential for associated spinal cord injury. Stress fractures may occur due to overuse activities and may affect the spine or the ribs. Clay-shoveler fractures are an overuse avulsion-type fracture of multiple lower cervical and/or upper thoracic spinous processes. They are caused by shear forces over the dorsal aspect of the neck, and several case reports include paddlers, baseball, and wrestling [8, 9]. Yamaguchi et al. reported on both a high school wrestler and baseball player, and acute posterior neck pain with an associated “pop” was reported by both patients. Both patients were treated conservatively and returned to play at 4 months.
Higher-energy activities such as snowboarding, rugby, American football, and motocross may cause higher-energy fractures. Anterior compression fractures should be considered in athletes with repetitive axial loading with flexion of the thoracic spine, including gymnasts, extreme sport athletes, and basketball players. Compression fractures are defined by anterior column failure with maintenance of the posterior elements of the spine, appearing as anterior wedging on lateral radiograph. Thoracic compression fractures rarely require operative management due to this isolated involvement of the anterior column and the stability inferred by the ribs and sternum. External orthoses such as a thoracic lumbar sacral orthosis (TLSO) or a Jewett brace are often used for comfort in patients with fractures involving T6 or below, although there is no data to support their use. Above T6, inclusion of the cervicothoracic junction is recommended with a Minerva brace. Elattrache et al. reviewed a professional football player who sustained T8 and T9 compression fractures after a football tackling injury [10]. There were no neurologic deficits, radiographs demonstrated 40% loss of vertebral height, and CT scan demonstrated isolated anterior column involvement. He was successfully treated with an extension thoracolumbar spinal orthosis for 12 weeks and successfully returned to play once radiographs confirmed no increased vertebral collapse or kyphosis.
Major thoracic trauma may result in unstable thoracic fracture patterns that have significant potential for associated spinal cord injury. These include burst, translation-rotation, and flexion-distraction fracture patterns or patterns with greater than single column involvement. Burst fractures involve failure of the anterior and middle columns, and retropulsion of fragments into the canal is often elucidated with a CT scan. Indicators of instability include loss of disc height, local kyphosis, PLC injury, and neurologic deficits. Treatment is aimed at facilitation of fracture healing, preservation of neurologic function, preventing progression of deformity, and restoration of range of motion, if possible. Burst fractures are mechanically stable injuries but are sometimes associated with neurologic deficits (neurologically unstable). Operative intervention is reserved for patients who have neurologic symptoms in the setting of an acute thoracic burst fracture. These injuries are typically treated with a posterior decompression and stabilization. Anterior decompression is considered if there is a large retropulsed bone fragment causing spinal cord compression.
Thoracolumbar Injury Classification and Severity Score (TLICS)
Injury characteristic | Qualifier | Points |
---|---|---|
Injury morphology | ||
Compression | – | 1 |
Burst | +1 | |
Rotation/translation | – | 3 |
Distraction | – | 4 |
Neurologic status | ||
Intact | – | 0 |
Nerve root | – | 2 |
Spinal cord, conus | Incomplete | 3 |
Complete | 2 | |
Cauda equina | – | 3 |
Posterior ligamentous complex integrity | ||
Intact | – | 0 |
Indeterminate | – | 2 |
Disrupted | – | 3 |

Chance fracture in an 18-year-old bareback rider. Transaxial computed tomography image of T12 vertebral body showing superior articular processes of T12 without normal articulation of T11. (a) Findings represent a “naked-facet” sign and signify distraction of posterior elements. (b) Anterior compression or “sandwich” sign of the vertebrae. In addition, note the absence of the spinous process which was fractured and unable to be seen in this image. (Reprinted from: Boham and O’Connell [12]. Journal of Athletic Training is an open access publication)
Chronic overuse activities can be associated with stress fractures. Elite rowers have an 8–16% rib stress fracture rate over the course of a career due to continuous, repetitive motion [13, 14]. The posterior ribs of such athletes are at risk for stress fractures due to the pull of the serratus anterior muscle, and they often occur after time off from sport when endurance is suboptimal. Costal cartilage fractures have also been case reported in an amateur rugby player [15].
Disc Herniation
The intervertebral disc functions to absorb axial stress and provide flexibility. Traumatic herniation of the nucleus pulposus through the annulus is caused by axial load and rotation on a flexed spine. In the general population, the incidence of thoracic disc herniation is estimated between 1 in 1000 and 1 in 100,000 [16]. While reported at every thoracic level, 75% occur below T8, with T11–T12 being the most common level due to increased spinal mobility and weakness of the posterior longitudinal ligament [17]. Thoracic disc herniation are rare injuries, although there have been case reports in elite soccer, football, and baseball athletes [18–20]. Symptoms often manifest as axial pain with possible associated radiculopathy and/or myelopathy. Radiculopathy is described as a band-like discomfort in a dermatomal distribution. Myelopathy is indicative of cord compression and is evidenced by both upper and lower motor signs such as gait disturbance, spasticity, clonus, weakness, and positive Babinski reflex. Upper extremity symptoms may predominate in high thoracic herniations, while lower herniations may mimic lumbar disc disease due to involvement of the lower extremities.
Radiographs are not usually useful in diagnosing disc herniation, but they should be obtained to rule out other osseous pathologies. MRI is the imaging modality of choice to diagnose thoracic disc herniation, and findings must be correlated clinically . As with cervical and lumbar disc herniations, the majority of thoracic disc herniation cases are successfully treated with conservative management, including rest, oral steroids, NSAIDs, and physical therapy. Directed injections can also be used both diagnostically and therapeutically in select cases.
However, if the symptoms persist after at least 6 weeks of conservative management, or there is progressive myelopathy or neurologic deficits, operative intervention is recommended. Preoperative CT and MRI imaging is helpful in identifying the herniation’s location, size, intradural extension, and associated calcifications . Surgical treatment often consists of a minimally invasive or open discectomy, with the approach dictated by the location of the herniation and adjunctive fusion reserved for cases with associated instability [16]. While elite athletes undergoing single-level lumbar microdiscectomy return to play 83.5% of the time, there are no current studies effectively powered to evaluate thoracic disc herniation return to play after discectomy [21].
Spinal Cord Injury
Over 17,000 new spinal cord injuries (SCI) are diagnosed per year (54 per million) in the United States alone, changing countless lives and costing millions of dollars over a patient’s lifetime [22]. An estimated 282,000 people are currently living with SCI in the United States, and males account for 80% of newly diagnosed cases. Incomplete and complete paraplegia account for 41.3% of diagnoses since 2010. While motor vehicle crashes are the most common cause, sporting injuries are the fourth leading cause behind falls and acts of violence. Sports most frequently cited include diving, horseback riding, American football, and skiing [1].
Initial evaluation in the case of suspected SCI should include comprehensive neurologic exam, including rectal exam and evaluation of the bulbocavernosus reflex. Spinal shock is a transient period of hyporeflexia and autonomic dysfunction following spinal cord injury, with the return of the bulobocavernosus reflex marking the end of spinal shock . Conversely, neurologic shock results from the loss of sympathetic tone and is characterized by hypotension and bradycardia. Current guidelines suggest maintenance of mean arterial blood pressure (MAP) greater than 85 mmHg may predict favorable functional outcomes in patients with SCI, although these guidelines are evolving [23]. The use of high-dose steroids (NASCIS Trials) is controversial but may have a role in young healthy patients who are treated within 8 h of injury [24–26]. Appropriate immobilization and spinal precautions are vital to prevent further injury during initial management. Operative decompression and stabilization may be necessary depending on the associated osseous injury, and emerging evidence suggests that early surgery may improve neurologic outcomes, particularly with incomplete SCI, and may reduce non-neurologic complications and health care resource utilization [27].
SCI is a multisystem pathology with low likelihood for complete recovery. Harrop et al. found in their review of thoracic and thoracolumbar spinal cord injuries that thoracic, specifically T4–T9, had the least likely potential for neurologic improvement [28]. As such, multidisciplinary management is vital in the long-term treatment of patients with SCI , as 30% of persons with SCI are rehospitalized one or more times during any given year following their injury, most often for genitourinary issues followed by integumentary, digestive, circulatory, and musculoskeletal causes [22].
Deformity
Spinal deformity represents a spectrum of pathologies affecting up to all three geometric planes (coronal, sagittal, axial) that commonly begin in childhood or adolescence and may have lifelong consequences. Idiopathic scoliosis occurs in 2–3% of the population, while excessive kyphosis occurs in up to 8% [29]. These rates vary in certain athletic populations, with Warren et al. noting a significantly increased prevalence (24%) of a minor curve in young ballet dancers and Tanchev et al. noting a tenfold increase in scoliosis frequency among rhythmic gymnasts [30, 31]. Regarding the sagittal profile, both thoracic kyphosis and lumbar lordosis increase during the adolescent growth spurt. These may be accentuated further in sports that require repetitive activities, such as hyperkyphosis in swimming and hyperlordosis in gymnastics. Plausible explanations for this include the repetitive asymmetric forces across the growth cartilage of the spine, particularly in prepubertal and pubertal athletes. Swimming, throwing, and serving have also been associated with asymmetric torque forces contributing to a functional scoliosis [32].
The decision on type and frequency of radiographic evaluation is based on pubertal status and physical examination. The forward bend Adams test evaluates thoracic rotation with a scoliometer. If the scoliometer reaches 7° in an immature child, a PA radiograph from C7 to the iliac crest is obtained. For coronal curves less than 25° of radiograph, observational treatment is recommended with the frequency dependent on puberty. A prepubertal athlete with a curve 10–14° may be reevaluated in 1 year, whereas a curve from 15 to 19° should be evaluated again in 3–6 months with a history and scoliometer examination. In prepubertal athletes with a curve of 20–24°, a repeat radiograph is performed in 3 months. A curve greater than 30° or one that manifests a progression of more than 5° should be considered for bracing. Bracing may include the Boston brace, worn 18–23 h per day, or a nighttime brace such as the Charleston or Providence brace. Curves that reach 40–45° in immature patients should be considered for surgical stabilization.
For Scheuermann’s kyphosis , the treatment is largely conservative, with surgery indicated in rare cases of progressive deformity and persistent symptoms. For curves of less than 50°, conservative management with stretching of the anterior chest wall/shoulders/hamstrings, extension-based strengthening, and core stabilization exercises can be effective. Bracing may be recommended for those with a curve between 50° and 70° and growth remaining. The type of brace is dependent on the level of the apex, with the Milwaukee brace indicated for an apex above T7 and thoracic-lumbar-sacral orthosis (TLSO) for an apex below T7. Ideally, the brace should be worn 16–18 h per day.
Nonetheless, while scoliosis and kyphosis may be aggravated by certain sports, the causal association is minimal, and athletes with scoliosis are treated similar to the general population. Athletes should be encouraged to continue sports participation. Brace wear is commonly prescribed for scoliosis and kyphosis, and many sports can be played with the brace. Even with sports that cannot practically be played with the brace, most bracing protocols have enough time out of the brace during the day to allow for continued participation. Appropriate therapy and exercises should be continued to enhance flexibility or strengthening of muscles about the spine.
Costovertebral Dislocation
Dislocation of the costovertebral articulation requires significant energy and is very rare. They often present with subtle radiographic findings and can portend a more serious injury and need for further workup. Often both radiographs and computed tomography (CT) are necessary for diagnosis. On anterior-posterior (AP) radiographs, asymmetric rib interspace narrowing above and widening below the level of injury can be seen. Another radiographic finding is the displacement of the costal head from the pedicle. On CT, the finding of a “naked transverse process” sign, or a transverse process with its associated rib displaced, is identified on the axial view [33]. The need for operative intervention is dependent on the presence of neurologic compromise and regional instability.
Degenerative Disease
Degenerative thoracic spine pathology is rare in the athlete population, likely due to the relatively young age of this population and stability of the thoracic region of the spine. Nonetheless, literature is sparse and suggests certain athletic population may be at higher risk than others. A study of athletes from the 2016 Olympic Summer Games demonstrated no athletes with moderate or severe degenerative disease of the thoracic spine [34]. However, a retrospective cohort study of adolescent motocross riders identified a significant increased risk of degenerative changes in the thoracic spine of riders compared to age-matched controls [35]. Furthermore, a study of 24 elite male gymnasts demonstrated a significantly higher incidence of reduced thoracolumbar disc intensity on MRI compared to age-matched controls [36]. While the long-term consequences are unknown, this risk should be acknowledged by parents and participants in select athletic subgroups, some of which have yet to be identified.
Rare Conditions
Physicians evaluating athletes with thoracic complaints should be aware of rare spinal pathologies that may present in the presence or absence of trauma. Conditions such as congenital thoracic stenosis, synovial cysts, spinal epidural lipomatosis, tumor, and infection can often be confirmed with a complete history, physical, and advanced imaging. These disorders may also predispose an athlete to a worse outcome in the setting of a traumatic thoracic injury.
Rare anterior thoracic musculoskeletal conditions may also be misdiagnosed as spinal pathology. “Slipping rib” syndrome is a rare condition often misdiagnosed and can lead to months of unresolved symptoms, as reported in a collegiate swimmer [37]. The condition arises from hypermobility of the anterior ends of the false rib costal cartilages, which often leads to slipping of the affected rib under the superior adjacent rib. This movement can lead to an irritation of the intercostal nerve, strain of the intercostal muscles, sprain of the lower costal cartilage, or general inflammation of the area. Surgical resection of the abnormal cartilaginous rib attachment was shown to provide a successful result and return to sport for the collegiate swimmer.
Return to Play
Given the rare nature of thoracic spine injuries , outcome data used to construct return-to-play recommendations are sparse, and no consensus opinions currently exist. General return-to-play criteria include full strength without neurologic deficit and painless full range of motion [38]. Athletes sustaining acute fractures with associated instability or neurologic symptoms should not participate in athletics. After surgical stabilization, furthermore, there are few proposed guidelines for return to play. Burnett and Sonntag recommend that spinal fusions bypassing or terminating at transition zones in the cervicothoracic or thoracolumbar region are an absolute contraindication to participation in contact sports [39].
Time lost from play by location of spinal injury
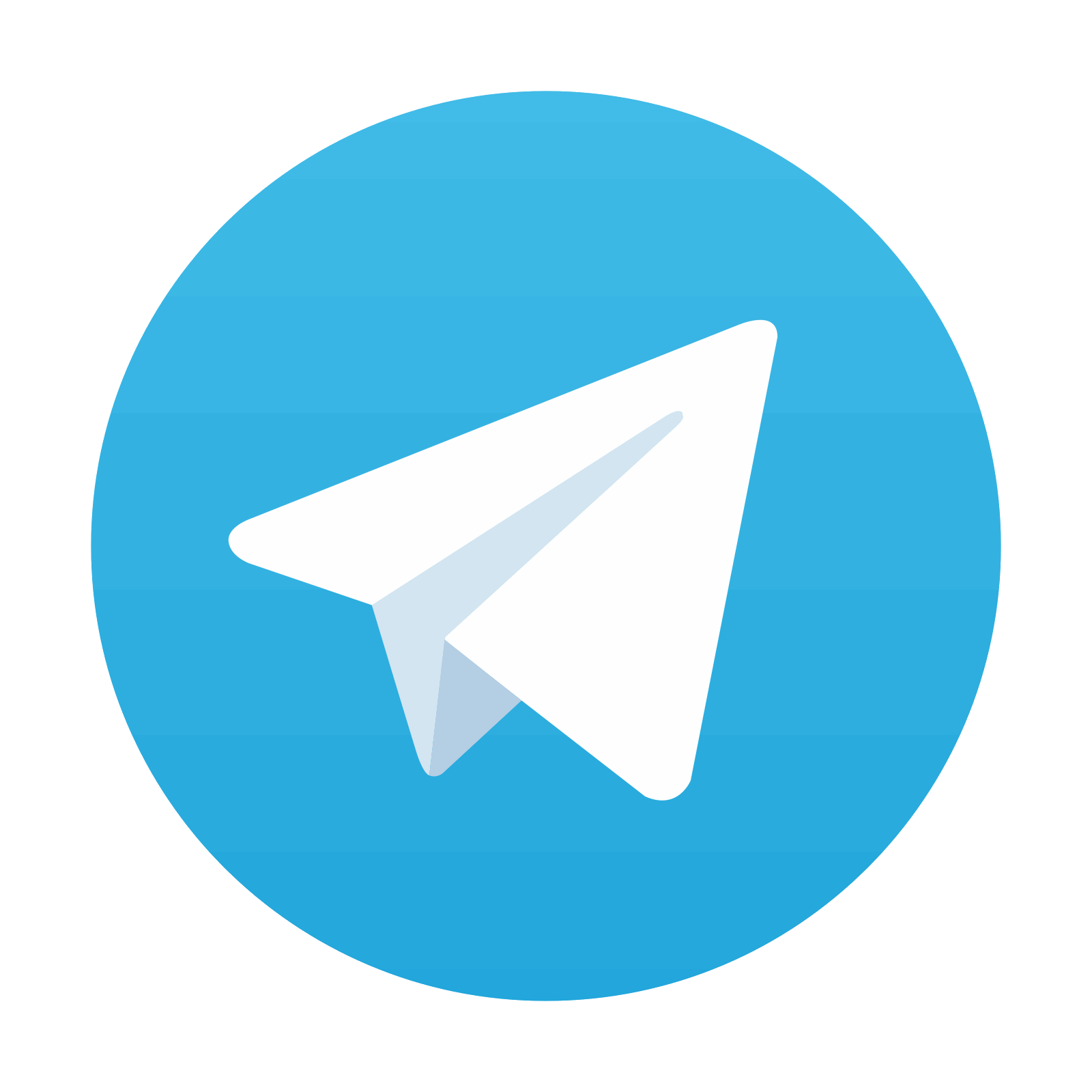
Stay updated, free articles. Join our Telegram channel

Full access? Get Clinical Tree
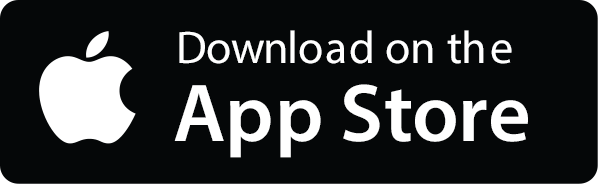
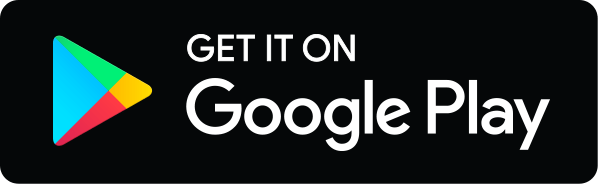