© ISAKOS 2017
Alberto Gobbi, João Espregueira-Mendes, John G. Lane and Mustafa Karahan (eds.)Bio-orthopaedics10.1007/978-3-662-54181-4_1313. Cell Culture Approaches for Articular Cartilage: Repair and Regeneration
(1)
Vericel Corporation, 64 Sidney Street, Cambridge, MA 02139, USA
(2)
Department of Orthopedic Surgery, Connective Tissue Biochemistry, University of California, 9500 Gilman Drive, La Jolla, San Diego, 92093-0630, CA, USA
13.1 Introduction
There are several published methods for culturing human cells including chondrocytes, osteocytes, and tenocytes to study musculoskeletal development, disorders, and for tissue engineering [1–8]. In this chapter we will focus on cell culture approaches used for articular cartilage repair. Articular cartilage defects are found in nearly 60% of knee arthroscopies [9, 10]. Many times these defects are painful and may result in loss of function. Articular cartilage lacks a blood supply and innervation and therefore has a limited capacity to heal itself. Untreated chondral defects may lead to a cycle of cartilage degradation and eventual osteoarthritis. The goals of cartilage repair are to restore smooth articular cartilage surface, relieve patient symptoms, and improve function. Nonsurgical options available for treating cartilage defects include debridement and lavage. First-line surgical treatment options include bone marrow stimulation techniques such as microfracture, abrasion arthroplasty, and subchondral drilling. Other treatment options include osteochondral autografts and allografts or autologous chondrocyte implantation (ACI). ACI uses autologous cell culture-expanded chondrocytes for the repair of cartilage defects. ACI is the first cell therapy approved for cartilage repair in the USA and is marketed under the name Carticel.® The technique was first described by Peterson, Lindahl, and Brittberg in 1987 [11]. It is a two-step procedure involving the harvest of cartilage from a non-weight-bearing location, in vitro expansion of chondrocytes, and reimplantation into the cartilage defect covered by a periosteal graft or off the shelf membrane. Very recently in December 2016, a third generation ACI product MACI® (autologous cultured chondrocytes on porcine collagen membrane) was approved in the USA. The MACI® technique is similar to ACI. The main difference is that with MACI,® expanded chondrocytes are delivered already seeded on a collagen I/III membrane for implantation with use of fibrin glue. On the otherhand, ACI involves a cell suspension injected under a periosteal flap or membrane sutured over the defect at the time of surgery. Mesenchymal stem cells (MSCs) are multipotent cells with enhanced proliferative capabilities that provide an attractive alternative source of cells for cartilage repair and other orthopedic applications. Cultured MSCs have been successfully used to treat cartilage defects. As an emerging technology, MSCs do not have as extensive a body of evidence as ACI, but clinical reports showing positive outcomes and ongoing clinical trials are advancing the field.
13.2 Basics of Cell Culture and cGMP Manufacturing
Cell culture involves removing tissues or organs from living organisms (e.g., humans) and propagating these in a laboratory to increase the cell numbers and to maintain the cells in an artificial environment for future use. Cell culture labs, regardless of cell type and intended applications, have some basic requirements including the maintenance of an aseptic environment. This is achieved by having a dedicated and aseptic work area in a laboratory such as laminar flow hood or biosafety cabinet that can be used for sterile handling of cell culture medium, reagents. In addition, some basic equipment required in a cell culture laboratory include incubators, water baths, centrifuges, sterilizers, cell counters, microscopes, refrigerators, and (−20, −80) liquid nitrogen freezer. The main difference between cell cultures which are intended for research use versus that intended for clinical use in patients is the requirement for good manufacturing practices (GMPs). GMPs provide for systems that assure proper design, monitoring, and control of manufacturing processes and facilities. This involves implementing a cell culture laboratory according to a stringent set of guidelines including standards for donor suitability, reagent qualification, testing, processing, preservation, storage, and distribution of human tissue. In the USA the standards are set by the Food and Drug Administration [12], and in Europe similar regulations exist [13, 14].
13.3 Articular Cartilage and Chondrocytes
Articular cartilage lines the surfaces of diarthrodial joints and is thickest over the ends of the femur and tibia where it ranges from 2 to 4 mm. This connective tissue serves to facilitate the movement of joints by providing a well-lubricated surface for distributing and transmitting loads [15]. Articular cartilage is avascular and lacks innervation and therefore has limited capacity to heal itself as a result of trauma or degeneration. Nutrition and oxygen to the tissue are supplied via diffusion from the synovial fluid [16]. Articular cartilage is highly specialized and biphasic with an extracellular matrix (ECM) composed of collagens, proteoglycans, and up to 80% water [15]. The ECM has a well-organized multilayered structure and is able to withstand high mechanical load. It is divided into four distinct zones, the superficial, middle, deep, and calcified zone; the tide mark demarcates the deep zone from the calcified layer. The main functional units of cartilage are chondrocytes, which make up about 1–5% of total volume of articular cartilage. These chondrocytes are responsible for producing the ECM. The main ECM produced by chondrocytes are collagens and glycoproteins with collagen type II making up to 90% of the collagen produced by chondrocytes; other collagens including VI, IX, X, and XI are produced in much smaller amounts [15, 17]. The chondrocytes are arranged within the ECM layers of cartilage, and they vary in size, shape, and number depending on location. The chondrocyte density is highest at the superficial layer [16]. Detailed reviews on the structure, function, and development of cartilage are available from various sources [15, 17].
13.4 Cultured Chondrocytes and Clinical Applications
13.4.1 Chondrocyte Cell Culture
Cell culture techniques have been developed to replicate and maintain the chondrocyte phenotype in vitro for clinical and cartilage tissue engineering applications [1]. When chondrocytes are isolated from cartilage tissue and grown in monolayer culture, they undergo a reversible dedifferentiation process whereby the cells lose their chondrocyte phenotype with a change in morphology from a round shape to fibroblastic-like [18, 19]. This physical change in the cells is accompanied by a downregulation of collagen II expression and several chondrogenic genes including SZP, BMP-2, TGFb1, FGFR3, COMP, aggregan, collagen IX, and SOX 9. Furthermore, there is an upregulation of collagen I, collagen X, collagen III, and tenascin and versican gene expression [20–22]. However, dedifferentiation can be reversed by transferring the cells from a monolayer culture to a three-dimensional culture such as an agarose or alginate suspension culture [18, 23–25]. Upon redifferentiation there is an upregulation of chondrogenic genes [26–28]. The cell and molecular mechanisms which control the dedifferentiation and re-differentiation of chondrocytes in cell culture have not been fully elucidated; however it has been found that the actin stress fibers which are involved in cell spreading play a role [29, 30]. The addition of growth factors of PDGF-BB, TGFβ1, and FGF2 has been found to increase cell proliferation and aid the recovery of chondrocyte phenotype in vitro [31, 32]. These in vitro properties of chondrocyte cultures and growth factors that affect them have been harnessed to develop cell culture protocols that optimize chondrocyte phenotype toward cartilage repair.
13.4.2 Method to Culture Chondrocytes for Autologous Chondrocyte Implantation
The purpose of this protocol is not to provide enough detail to replicate but rather have the reader develop an appreciation for the steps, processes, and reagents involved in chondrocyte cell culture. More detailed protocols are available elsewhere [1, 33–35]. In the USA, culture and manufacture of Carticel® is based on the protocol originally developed by Tubo and Binette [1, 11]. A cartilage biopsy of about 200–300 mg is retrieved from a non-weight-bearing area preferably the intercondylar notch in an outpatient arthroscopic procedure by the treating surgeon. The biopsy is then transported in serum-free DMEM to the cGMP laboratory for processing and cell culture expansion [11]. Within 48 h of arrival in the laboratory, the biopsy is treated with enzymatic digestion and minced in to 0.5 mm to isolate the chondrocytes [1]. The digestion medium consists of 0.1% w/v collagenase solution in DMEM at 37 °C. The minced cartilage in digestion medium is transferred into a centrifuge tube and then put into an incubator at 37 °C overnight. The following day the digested cartilage is removed from the incubator, supernatant collected, and transferred to another tube. The enzyme is inactivated by adding more media and the cells counted with a hemocytometer. The cell suspension is then centrifuged to concentrate the cells and the cell pellet added with media (DMEM with 10% FBS) into a flask for expansion and placed in incubator at 37 °C [1]. The cell media is replaced every 2–3 days, and the cells are passaged once they reach 80–90% confluence. The passaged cells are diluted 1:10 in cell culture media (DMEM with 10%FBS) and seeded for further monolayer expansion [1]. Cell culture expansion for 2–3 weeks is needed to produce approximately 12 million cells. Typically, cells are cryopreserved after initial cell expansion and retrieved for further expansion after an order made. At which point, 2–3 days before the patient’s surgery for implantation, the cells are processed into shipping vials. Several assays are performed to verify the final product including sterility testing, Mycoplasma, viability, identity, potency, and visual inspection. The BacT/Alert system is used for sterility testing [36]. Cell identity assays involving gene expression analysis distinguish chondrocytes from synoviocytes and dermal fibroblasts [37]. There are different protocols available for chondrocyte culture and expansion, and many of those used for commercial production of chondrocytes for clinical applications are propriety. In general, differences in protocols will include the type of cell culture medium used, use of serum, growth factors, and other supplements [20].
13.4.3 Current and Emerging Clinical Applications of Cultured Chondrocytes
To date there have been two culture expanded cell therapy products that have been approved in the U.S.A by the FDA for cartilage repair, Carticel® and MACI®. There are several products that use culture-expanded chondrocytes that have been approved in other countries, are currently in clinical development, or have terminated their clinical trial or manufacture. ChondroCelect® was the first cell-based product to be approved in Europe. The company has ceased manufacture of the product. It is a two-stage procedure where cultured autologous chondrocytes are implanted in a suspension under a periosteal flap or membrane. MACI® (matrix-induced autologous chondrocyte implant) is a third-generation ACI product that was marketed in the EU. The manufacturer of the product, Vericel Corporation, received approval for MACI® in the USA in December 2016. MACI® consists of autologous cultured chondrocytes seeded onto a 14.5 cm2 resorbable type I/III collagen membrane at a density of 500,000 to 1 million cells per cm2 which can be trimmed by the surgeon according to the size and shape of defect [38]. NOVOCART 3D® is also third-generation ACI product that is currently undergoing clinical trials in the USA for articular cartilage repair of the knee. The product consists of autologous chondrocytes seeded on a bovine collagen type 1/chondroitin sulfate sponge. Prior to seeding the chondrocytes are cultured in autologous serum for one passage without the use of antibiotics. The duration from isolation of the chondrocytes to completed product is 3 weeks. NeoCart® consists of autologous cultured chondrocytes on a bovine type I collagen scaffold. The cell culture involves a biomimetic bioreactor with hydrostatic pressure which is applied during the first 6 days of culture; hypoxia (2% O2) and perfusion at 5 μL/min are also part of the bioreactor culture process. It is believed that these conditions increase S-GAG production and cell proliferation. It takes approximately 67 days from biopsy before the implant is available.
13.5 Mesenchymal Stem Cells and Clinical Applications
Mesenchymal stem cells (MSCs) are self-renewing multipotent cells that have tri-lineage differentiation capacity. MSCs were originally discovered to reside in the bone marrow, but subsequent studies have shown that MSCs exist in multiple tissue depots including peripheral blood, adipose, synovium, infrapatellar fat pad, dermis, periosteum, trabecular bone, cord blood, and placenta [39–41]. In fact, it is hypothesized that MSCs reside in every vascularized tissue as pericytes [42]. The International Society for Cellular Therapy (ISCT) in 2006 has defined a limited set of criteria to identify MSCs experimentally [43]. The cells in culture must be plastic adherent, and more than 95% of the MSCs must express CD73/5′ nucleotidase, CD 90/Thy 1, and CD105/endoglin as measured by flow cytometry. In addition, less than 2% of the cell population should express CD34, CD45, CD11b/integrin alpha M por, CD14, CD79 alpha or CD19, and HLA class II a. Furthermore, the cells must demonstrate tri-lineage differentiation potential into osteoblasts, adipocytes, and chondrocytes under standard differentiating conditions as assessed by in vitro immunostaining. MSCs exist as pericytes on vascularized tissues and under the right conditions demonstrate tropic, paracrine, or immunomodulatory functions [42]. It is hypothesized that during injury these pericytes are released from the vessel wall and become activated and release bioactive factors that initially inhibit immune cells that come to survey the damage site and prevent autoimmune activities [44–47]. The trophic factors secreted by the MSCs create a regenerative microenvironment to support the healing of the damaged area. For example, trophic molecules inhibit apoptosis and secretion of VEGF stimulates angiogenesis [48]. It has also been discovered that MSCs make an antimicrobial protein called hCAP-18/LL37 [49]. MSCs from various sources including the bone marrow, adipose, synovial tissue, and umbilical cord have been shown to have chondrogenic potential, making these cells of great interest as an alternative source for cartilage repair. Furthermore, there is not the issue of donor site morbidity associated with the use of chondrocytes from cartilage biopsy.
13.5.1 Culturing Mesenchymal Stem Cells
There is a great variation in the initial number of MSCs depending on the source [39]. Cell culture can be used to expand the low populations of MSCs found in some tissues in order to generate sufficient quantities for therapeutic use. For example, MSCs within the bone marrow comprise just a small portion, approximately 0.001–0.1%, of the mononuclear cell population within the bone marrow [50–52]. Furthermore, MSCs are found within a milieu of heterogeneous tissue, and cell culture enables the enrichment of MSC populations. MSCs are capable of self-renewal and can be expanded considerably in vitro; however at about five or more passages in culture, MSCs start to exhibit changes in morphology, reduced proliferation, and shortening of telomeres and change the profile of MSC markers [53–57]. The addition of FGF2 has found to extend the ability of MSC cultures to maintain their self-renewing and differential potential for longer [58].
There have been several approaches reported to direct the differentiation of MSCs into chondrocytes in vitro. The most common protocols for chondrogenic differentiation involve pellet cultures [59, 60] with various media components and growth factors. In pellet culture MSCs undergo cell condensation as well as cell to cell and cell to extracellular matrix interactions [61, 62]. This is followed by a highly proliferative stage whereby the cells start to produce collagen type 2 and other components of the ECM [63–68]. In the terminal stages of differentiation, the cells become rounded and begin to exhibit signs of hypertrophy including the expression of collagen type X and MMP13. The different stages of chondrocyte differentiation are controlled by transcription factors including SOX5, SOX6, and SOX 9 [69–72]. The addition of key growth factors to the cell medium is essential for the differentiation process. TGFβ is a well-established growth factor known to induce chondrogenesis [60, 73]. Some protocols also include BMPs [74–76] or IGF1 [59] as growth factors. In addition, environmental cues such as mechanostimulation [77] and hypoxia [78, 79] have been shown to be modulators of MSC chondrogenesis. There are several methods to assess chondrogenesis in MSC cultures. The production of ECM is associated with a substantial increase in cell mass that can be assessed by changes in the weight of the cultures over time. The production of COL2A1 can be measured by gene expression with increase in expression from 100- to 1000-fold in chondrogenic cells compared to the primary MSC cultures. The generation of proteoglycans can be examined by histological staining with alcian blue, toluidine blue, or safranin O [80]. The tendency of in vitro MSC-derived chondrocytes to undergo hypertrophy and subsequent osteochondral ossification is undesirable. This transformation into hypertrophic chondrocytes triggered by the endochondral pathway is marked by an upregulation of osteogenic genes [81] and collagen type X [82]. There is still room for improvement in terms of understanding the basic biology of MSCs and optimizing culture conditions to ensure that tissue-engineered products provide high-quality and durable tissue.
13.5.2 Emerging Clinical Applications of Cultured Mesenchymal Stem Cells for Cartilage Repair
Currently there is no FDA-approved product that uses MSC-derived cells for cartilage repair or any other orthopedic indication. However, there have been several clinical reports and clinical trials using MSCs. None of the reported clinical applications of cultured MSCs for cartilage repair use directed differentiation into chondrocytes in vitro. Rather, the cultured multipotent MSCs are directly applied onto a scaffold, gels, and other carriers and surgically implanted into the defect, where presumable in vivo signals within the defect direct the differentiation of the MSCs for repair.
13.5.2.1 Clinical Reports
There have been several reports describing the use of culture-expanded MSCs for cartilage repair. The protocols involve isolation of MSCs and culture expansion in the laboratory for 2–3 weeks, and the cells are surgically implanted with or without membranes. The first report using autologous MSCs for the treatment of full-thickness cartilage defects was in 2002 by a group in Japan, Wakitani et al. [83], where BMSCs (bone marrow mesenchymal stem cells) were evaluated in osteoarthritic knees in combination with high tibial osteotomy (HTO). There were 24 patients of whom 12 received BMSC treatment and 12 had no cells. At the 16-month evaluation period, there was no statistically significant difference in the clinical outcomes between the two groups although the group with the BMSC treatment had better arthroscopic and histological grading scores [83]. Subsequently the group reported treatment using BMSCs for patella defects in a case report of two patients, a 26-year-old female and 42-year-old male [84]; patellofemoral defects in five knees of three patients, a 31-year-old woman and 46- and 42-year-old men [85]; and in the femoral condyle defect in a 31-year-old. There are two studies that compared cultured MSCs to autologous chondrocyte implantation. Nejadnik et al. [86] reported a study to treat grade III and IV chondral lesions of the knee. This level III (cohort) study involved 72 patients matched by lesion site and age. Thirty-six patients underwent treatment with ACI and 36 underwent treatment with BMSC. The etiology of the defects included trauma and OA. The mean defect size for the ACI treatment group was 3.6 cm2 (SD 2.84 cm2) and for the BMSC group 4.6 cm2 (SD 3.53 cm2) with no statistically significant difference in size between the two groups. The clinical outcomes were measured before treatment and several time points up to 24 months after surgery. The outcome measures were SF36, IKDC, Lysholm knee scale, and Tegner activity. The results of this study demonstrated significant improvement in SF36 after cartilage repair for both groups. There was no statistically significant difference between treatment groups on IKDC, Lysholm, and Tegner over time. However SF36 demonstrated greater improvement over time for the BMSC group. It was found that improvements were greater among men than with women. Furthermore, in the ACI group, improvement was statistically significantly better in patients younger than 45 years old.
Akgun et al. [87] reported a single-site prospective randomized single-blind pilot study of 14 patients; seven patients were treated with MACI and seven with synovial-derived stem cells which were culture expanded (m-AMI). The mean defect size for the m-AMI group was 2.9 ± 0.8 cm2 and 3.0 ± 0.8 cm2 for the MACI groups. The chronicity of symptoms was greater than 3 years in each group. The clinical and radiological outcomes were compared and assessments were performed preoperatively and at several time points up to 24 months after surgery. The results demonstrated that at all follow-up intervals, m-AMI-treated patients did significantly better than MACI-treated patients on the subjective subscale scores for pain, symptoms, activities of daily living, and sport and recreation of KOOS. Furthermore, m-AMI had significantly better scores at 6 months compared to MACI for the KOOS quality of life subscale and VAS. Magnetic resonance imaging (MRI) did not display any graft failure for either group at 24 months, and parameters were rated good to excellent in the m-AMI group and as fair or good in the MACI group postoperatively. In conclusion, use of m-AMI can accelerate recovery in the treatment of chondral defects of the knee.
Most of the other studies using cultured MSCs were case series. Most recently, Sekiya et al. [88] reported a case series involving ten patients with symptomatic single cartilage defects of the femoral condyle. The objective of the study was to evaluate if synovial stem cell transplantation for cartilage repair improves MRI features, histological features, and clinical evaluation scores in patients with cartilage defects in the knee. All were traumatic lesions. There were five males and five females. The mean age of patients was 41 years (range 20–43 years). The mean chronicity of symptoms was 3 years (range 0.6–16 years). The mean lesion size was 200 mm2 (range 25–500 mm2). The mean follow-up period was 52 months (range 37–80 months). The synovium with sub-synovial tissue on the femur at the suprapatellar pouch was harvested with a pituitary rongeur. The synovium was digested with lipase and culture expanded in 10% autologous human serum for 14 days. Forty-seven million ± 21 million expanded MSCs in Ringer’s solution were applied arthroscopically into the defect. Five patients also underwent concomitant surgeries. MRI and histology were used to evaluate the quality of cartilage repair, and clinical outcomes were assessed with Lysholm score and Tegner activity. Tegner activity did not decrease after treatment in each patient. There was an increase in Lysholm from the value prior to surgery (76 ± 7) to 95 ± 3 after surgery (median 95% CI, p = 0.005). MRI results were graded 1–5. The MRI score increased from a mean of 1.0 ± 0.3 prior to surgery to 5.0 ± 0.7 after surgery (p = 0.005). Second-look arthroscopy in four patients showed better cartilage. Histology showed hyaline cartilage in three patients and fibrocartilage in the deep zone of one patient. It was concluded that synovial MSC transplantation for cartilage defect repair was effective with a statistically significant increase in Lysholm and qualitative measurements of graft quality by MRI and second-look arthroscopy. Overall clinical studies have demonstrated positive results in clinical and histological grading score with minimum adverse effects. However, larger numbers of randomized clinical trials with long-term follow-up are needed.
13.5.2.2 Clinical Trials Using Cultured Mesenchymal Stem Cells for Cartilage Repair
There are several clinical trials exploring the use of cultured MSCs cells for the treatment of cartilage defects. These trials include allogenic or autologous sources. The tissue depots from which the MSCs are extracted include the bone, adipose, and cord blood. Details about the study design of these trials can be found on clinical trials dot gov website (www.clinicaltrials.gov). Allogenic sources of MSCs are of interest because they can provide an off-the-shelf product for cartilage repair that may cut down costs and provide the option for a one-step surgical procedure. CARTISTEM is an allogenic product produced by isolating MSCs from umbilical cord blood, which is then cultured and mixed with a semisolid polymer and administered into the cartilage lesion by orthopedic surgery in order to stimulate the regeneration of cartilage and improve function. There are four trials registered on clinical trials dot gov reporting the use of CARTISTEM for cartilage repair. The phase III trial NCT0141001 was a study to compare the efficacy and safety of CARTISTEM and microfracture in patients with knee articular cartilage injury or defect. There is also a long-term follow-up study to this NCT0162677 in which subjects who participated in and completed the phase III trial NCT01041001 will be tracked until the 60-month posttreatment time point. In 2015 the Korea-based company Medipost, the developer of CARTISTEM, announced 3-year results of the clinical trial from ten centers in Korea that demonstrated safety with superior treatment compared to the reference group in outcome measures of knee pain, function vitality assessment (IKDC), WOMAC, and VAS. There is also a phase 1.2a clinical trial of CARTISTEM in the USA in patients with focal, full-thickness grade 3–4 articular cartilage defects of the knee. This study led by Dr. Brian Cole entitled “Evaluation of Safety and Exploratory Efficacy of CARTISTEM” completed enrollment of 12 patients in 2015. Another study using CARTISTEM for osteochondral lesion of the talus (NCT02338375) is entitled “safety and efficacy of allogenic umbilical cord blood derived mesenchymal stem cell product.”
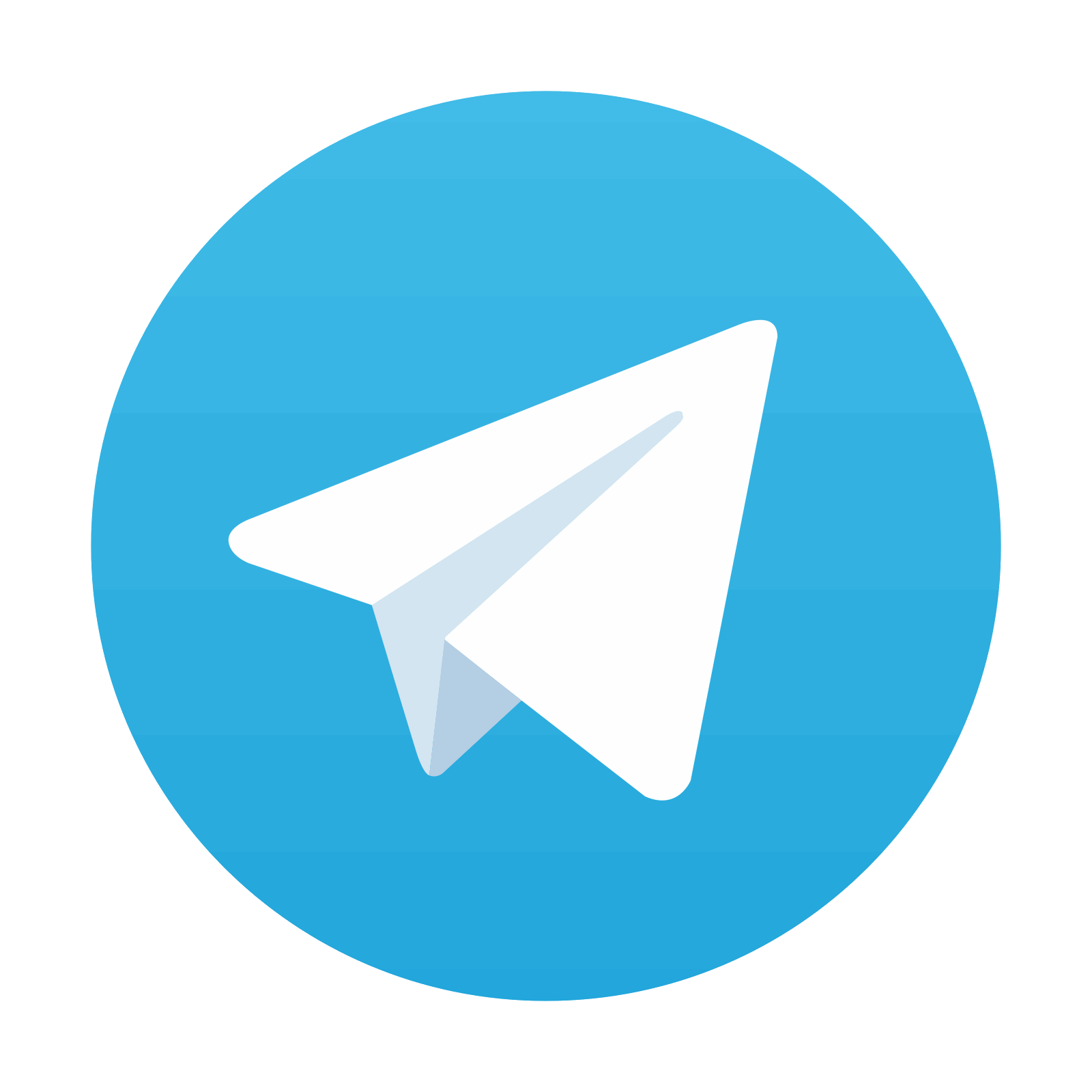
Stay updated, free articles. Join our Telegram channel

Full access? Get Clinical Tree
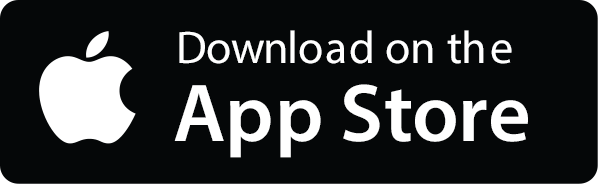
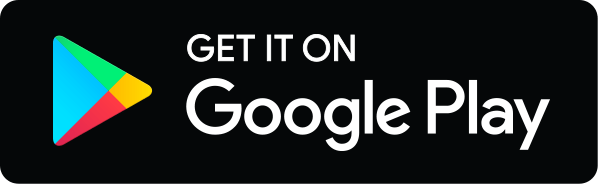
