Chapter 27 Biomarkers for Stool Analysis
Introduction
Gastrointestinal (GI) dysfunction is a remarkably common experience in the United States.1 The popular media are saturated with advertisements promising to soothe a wide variety of complaints, including (but by no means limited to) constipation, indigestion, reflux, bloating, and cramping. Those seeking relief beyond that offered on their local pharmacy shelves are substantial in number: GI-related complaints account for a significant percentage of visits made to primary care providers’ offices.2 As a group, many GI diseases carry significant morbidity and mortality—and many are currently poorly treated or in some cases completely untreated.3 Yet the burden of GI diseases goes beyond individual physical costs: one survey estimated that over 40 billion dollars are dedicated to addressing GI-related issues annually.4 Given the substantive toll that GI-related illnesses place on patients and the health care system—and given that current treatment methods to address them may be less than effective—it must be asked: what options do clinicians have to fully support their patients?5
Laboratory diagnostics that assess the stool for insight into the state of health of the gut have existed for decades, yet the evolution of technology is such that more sensitive and specific tools now exist for easy clinical application. Biomarkers for stool analysis measure key physiologic aspects of the gut: digestion/absorption (D), immunology/inflammation/infectious processes (I), and gut microflora balance (G) (or DIG). This physiologic assessment of DIG mirrors functional status, and provides a critical noninvasive way to evaluate and clinically approach the patient with GI complaints (Figure 27-1). Assessed together, stool analysis biomarkers provide an effective means of capturing underlying physiologic disruption that manifests as disease: eliminating the need for costly diagnostic testing where unnecessary; highlighting those individuals specifically at risk for organic disease who require immediate specialty referral; and identifying the source of gut dysfunction to apply simple, cost-effective treatments.
Overview of the Gastrointestinal Tract
A popular adage expounds, “You are what you eat.” Over the course of a lifetime, it is estimated that a well-nourished adult will consume between 25 and 50 tons of food.6 Yet given that these macronutrients—fats, carbohydrates, and proteins—must ultimately be broken down, taken up, and assimilated, perhaps the more appropriate maxim is, “You are what you digest and absorb.”
Once taken in, dietary foodstuffs undergo a series of complex digestive processes in this tube within a tube. The human gut has a massive absorptive surface area to capture macronutrients and micronutrients critical to optimal physiologic function, yet the impact of its processes are increasingly understood to go beyond traditionally defined roles of digestion and absorption. The mucosal surface of the GI tract is the primary interface with our external environment, and gut commensal microflora interaction with this mucosal surface is critical for differentiating self from nonself—a phenomenon known as “oral tolerance.” In addition, more than 70% of the body’s immune tissue sits within the gut, and is both closely interconnected to—and continuously interacting with—the largest concentration of nervous tissue outside of the central nervous system (CNS). These immunomodulatory and neuroendocrine functions give credence to clinical concern that gut dysfunction may be a root cause not only at the level of local digestive issues like constipation, gastroesophageal reflux disease, and irritable bowel syndrome (IBS), but also for many chronic, systemic illnesses like inflammatory bowel disease (IBD), Hashimoto thyroidits, systemic lupus erythematosus (SLE), rheumatoid arthritis, and migraine.
Digestive Physiology 101
Brain
As anyone watching a late-night food commercial may attest to, the process of digestion begins long before any food enters the mouth or stomach. The cephalic phase of digestion begins when anticipatory, visual, gustatory, and/or olfactory stimuli activate CNS centers, and result in parasympathetic stimulation of gastric activity.7
Mouth
Homogenization of ingested food first occurs in the mouth via the grinding and mixing action of the teeth. Salivary glands provide hydration to form a bolus and protect the pharyngeal and esophageal mucosa, primarily with secretory immunoglobulin-A (IgA) antibodies. The process of starch and fat hydrolysis is initiated with the release of lingual lipase, salivary amylase, and ptyalin.8,9
Stomach
The stomach produces churning action, and initiates protein and lipid hydrolysis. Peptides, amino acids, and fatty acids released in this process synchronize the release of pancreatic juice and bile into the small intestine.8 About 2 L of gastric juice are produced each day, containing several important components.10 Hydrochloric acid (secreted by the parietal cells) activates pepsinogen to convert to pepsin, which renders some minerals (e.g., calcium and iron) more absorbable. It also creates an essentially sterile environment to prevent bacterial overgrowth. Mucus forms an acid- and pepsin-resistant coating for the stomach lining. Gastric lipase secreted by gastric mucosa begins to hydrolyze triglycerols, producing 1,2-diacylglycerols and fatty acids. The optimal pH of gastric lipase is about 4, but the enzyme is active up to pH 6 or 6.5.11
Pancreas
Primary pancreatic exocrine insufficiency results from organic diseases of the pancreas (e.g., chronic pancreatitis, cystic fibrosis, severe acute necrotizing pancreatitis, pancreatic cancer). Secondary pancreatic exocrine insufficiency results from extra-pancreatic diseases such as celiac disease and Crohn’s disease, GI and pancreatic surgical resection, as well as any condition that results in a compromise or blunting of the small intestinal brush border. These include inflammatory processes, infectious agents, food allergies, and dysbiosis and/or small bowel bacterial overgrowth. Whether of primary or secondary etiology, any compromise of pancreatic exocrine function can result in significant malnutrition related morbidity and mortality.12
Small Intestine
Most digestion and assimilation occur in the small intestine. Digestion is mediated chiefly by pancreatic enzymes, including proteolytic enzymes, nucleolytic enzymes, lipases, α-amylase, and phospholipase A2, which hydrolyze macromolecules to oligomers, dimers, or monomers.8 Pancreatic enzymes are most active in the neutral pH range,10,13 and bicarbonate begins the process of neutralizing stomach acid. Pancreatic proteases are secreted into the duodenum as inactive precursors, trypsinogen, chymotrypsinogen, and proelastase, and are activated by brush-border enzymes, such as enterokinase, to form trypsin, chymotrypsin, and elastase, respectively.10
The digestive and absorption capacities of the small intestine are greatly enhanced by circular folds and fingerlike projections of the intestinal mucosa called microvilli. This region, dubbed the “brush border,” increases the luminal surface area of the intestinal wall by about 600 times, creating a total absorptive surface of approximately 200 to 250 m2, essentially the size of a tennis court.10,13,14
Even after prolonged contact with pancreatic enzymes, a substantial portion of ingested carbohydrates and amino acids depend on hydrolytic enzymes (disaccharidases such as maltase, sucrase, lactase, enterokinase, and peptidases) produced by the brush-border membrane to complete their digestion.10
For this reason, damage to the intestinal brush border—resulting from inflammation, trauma, parasitic infection, bacterial overgrowth, long-term nonsteroidal anti-inflammatory drug use, and other factors—may interfere significantly with the proper absorption of nutrients. Such damage also can disrupt the intracellular tight junctions that are vital to the protective barrier function of the gut mucosal layer, increasing the intestinal permeability of potentially harmful macromolecules, such as bacteria and toxins, into the systemic circulation. This state of increased intestinal permeability, sometimes referred to as “leaky gut,” has been cited as a potential contributory or causative factor in gluten enteropathy, Crohn’s disease, food allergy, various arthritides and autoimmune diseases, chemotherapy complications, and a variety of other clinical conditions.15–19
Large Intestine
A primary role of the large intestine is to absorb water, about 1 L/day. The large intestine also provides an environment for microbial fermentation of soluble fiber, starch, and undigested carbohydrates. Soluble fibers are more readily fermented than insoluble fibers.20
Nondigestible carbohydrates (e.g., fiber), polysaccharides, and oligosaccharides contain chemical bonds that make them incapable of complete digestion by pancreatic or brush-border enzymes. As they move through the digestive tract, these undigested compounds play an important role in intestinal transport mechanisms. Once in the colon, they are cleaved and modified by enzymes produced by resident colonic bacteria to form short-chain fatty acids (SCFAs) and various gases, such as methane, hydrogen, and carbon dioxide.11
Digestion/Absorption
Digestive Dysfunction
As an example, hypochlorhydria has been hypothesized to be the root cause of a number of clinical conditions, including reduced mineral and amino acid absorption, depression, and other syndromes.21,22
In addition, interest has been expressed in the medical literature about the connection between the robust use of pharmaceuticals promoting gastric acid blockade and chronic clinical complaints. Research indicates that small bowel bacteria overgrowth, and associated disorders such as blunting of brush-border enzyme activity and decreased pancreatic exocrine function, may be an iatrogenic consequence of overprescribing of proton pump inhibitors.23–25 Given that long-term use of drugs that markedly reduce gastric acid secretion may negatively affect dietary and nutriceutical calcium absorption, concern has also been raised about their potential to increase the risk of fracture in individuals with osteoporosis, although the greatest potential for this may be in patients already at risk for loss of bone mineral density.26–29
Maldigestion associated with pancreatic exocrine insufficiency results in an inadequate delivery of enzymes to the small intestine, and can lead to inadequate breakdown of fats, carbohydrates, or protein. The net effect is poor nutrition and an unhealthy environment for the flora of the large intestine. Significant decreases in exocrine pancreatic secretion have been associated with the aging process,30 and clinically, may be associated with osteoporosis31,32 and diabetes.33–35
Absorptive Dysfunction
• Small bowel bacterial overgrowth
• Deficient bile production, resulting in inadequate solubility of fatty acids11
• Chronic inflammation of the small intestine
• Rapid transit, which does not allow adequate time for absorption
Malabsorption often increases with age36: atrophic gastritis is estimated to affect 10% to 30% of individuals more than 50 years old and often leads to inadequate absorption of key nutrients such as vitamin B12.37
Biomarkers for Digestion/Absorption
Pancreatic Elastase 1
Pancreatic elastase 1 (PE1) is a proteolytic enzyme secreted exclusively by the human pancreas, and as such, reflects overall pancreatic exocrine function.38 It is an extremely stable, reliable, and specific marker,39 and correlates well with gold-standard, stimulated pancreatic function tests.40 PE1 is concentrated five- to six-fold higher in feces than when it enters the duodenum, reflecting an overall stability of this biomarker in the GI tract.41 It is not degraded during intestinal transit, and is generally not affected by increases or decreases in intestinal transit time,42 although its sensitivity may be diminished in cases of liquid diarrhea.43 Unlike other pancreatic markers, such as chymotrypsin, PE1 results are not affected by pancreatic enzyme replacement therapy,39,40 a feature that makes it a valuable marker for monitoring and adjusting exogenous enzyme replacement.39
PE1 appears to have less sensitivity when diagnosing mild exocrine pancreatic insufficiency, and may have limited use in differentiating pancreatic from nonpancreatic steatorrhea or diarrhea.44
Although research typically indicate levels of PE1 less than 200 mcg/g to be consistent with normal pancreatic exocrine function, healthy individuals produce on average 500 mcg/g of PE1. Given that, PE1 levels greater than 200 mcg/g and less than 500 mcg/g suggest a deviation from optimal pancreatic function. PE1 values between 100 and 200 mcg/g suggest moderate pancreatic insufficiency, and values less than 100 mcg/g are considered to be consistent with severe pancreatic insufficiency.45,46 Enzymatic support of pancreatic insufficiency is warranted, and strength of supplementation–typically based on the level of lipase units–should be modulated based on the degree of pancreatic insufficiency indicated by the PE1 level (Table 27-1).
TABLE 27-1 Pancreatic Elastase 1 Levels, Degree of Pancreatic Insufficiency, and Enzyme Supplementation
>500 mcg/g | Optimal |
351-500 mcg/g | Normal, but not optimal |
201-350 mcg/g | Mild insufficiency |
101-200 mcg/g | Moderate insufficiency |
<100 mcg/g | Severe insufficiency |
Reduced PE1 has been reported clinically in patients with type 1 and type 2 diabetes.47,48 chronic pancreatitis,49,50 osteoporosis,32 cystic fibrosis,51 trauma states,47 and in intestinal malabsorption states associated with mucosal atrophy such as celiac disease.52,52a
Putrefactive Short-Chain Fatty Acids
Valerate, isovalerate, and isobutyrate are produced exclusively by bacterial fermentation of proteinaceous material (polypeptides and amino acids). These SCFAs are putrefactive, and their presence suggests underlying maldigestion and/or malabsorption from dysfunctional states such as hypochlorhydria or exocrine pancreatic insufficiency,53 or bacterial overgrowth in the small intestine. Other causes are GI disease (resulting from the fermentation of blood or mucosal cells delivered to the colon)54 and rapid transit time (resulting from inadequate time for digestion and absorption of peptides and amino acids).55
Fecal Fats
Fecal fats include triglycerides, long chain fatty acids, cholesterol, and phospholipids, and are derived primarily from the dietary ingestion of fat. Elevated levels of fecal fats in the stool suggest fat malabsorption (steatorrhea), which can occur as a result maldigestion and/or impaired uptake of fatty acids. In addition, any condition that results in impairment of lipase activity and bile acid production and release may result in fat malabsorption. These include pancreatic insufficiency, cholestatsis, short bowel syndrome, and celiac disease.56
Immunology/Inflammation/Infectious Processes
As noted previously, more than 70% of the body’s immune tissue sits within the GI tract.57 This immune tissue has a complex and dynamic relationship with the commensal microflora that colonize its surface—the primary interface with an external environment rich in both supportive dietary antigens as well as pathogenic agents. The gut commensal microflora exist in this macronutrient dense intestinal environment, and are significant contributors to the process of digestion, building of essential nutrients, and holding nonbeneficial bacterial counts in check. How is it then that host immune function is modulated to allow for the acceptance of self (as well as supportive commensal organisms and dietary antigens), even as it is vigilantly surveying for and combating against pathogenic microorganisms?
The answer appears to lay with an exquisitely balanced, ongoing inflammatory response by the intestinal immune system: it is capable of mounting strong inflammatory responses against pathogenic agents while concomitantly providing inhibitory signals that preclude responses against commensal bacteria.58,59 Any alteration of this equilibrium, for example, as a result of gut microflora imbalance, genetic predisposition, or immune dysregulation, will result in an upregulation of the gut inflammatory response. Diagnostically, this may be reflected by the release of the biomarker calprotectin from neutrophils.60 Clinically, the breakdown of this intestinal immune balance has been implicated in the development of diseases marked by significant inflammation, such as IBD,61–63 and has been postulated to play a role in the development of extraintestinal allergic and autoimmune disorders.64 Microflora imbalance has also been reported in patients with IBS.65
Biomarkers for Immune Function/Inflammation/Infectious Processes
Eosinophil Protein X
Eosinophil protein X (EPX) is a basic cellular protein with potent cytotoxic and neurotoxic properties, and is a marker of eosinophil activation and degranulation. Mobilization of eosinophils in the gut appears to occur in response to immune-mediated inflammatory responses.66,67
Clinically, fecal elevations of EPX have been described in IBD, IgE-mediated food allergy, parasitic infections, and collagenous colitis.68–79
Calprotectin
Calprotectin belongs to a group of calcium-binding neutrophil-derived proteins. Because neutrophils are typically mobilized in response to increased mucosal permeability, cell or tissue damage, and infectious processes, elevations in calprotectin may be seen in the presence of inflammatory, neoplastic, and/or infectious disease processes.80,81
Clinical application of this biomarker has been directed toward discerning the best approach for the patient with functional abdominal symptomology. Specifically, calprotectin has been evaluated (in conjunction with Rome criteria) as a diagnostic biomarker to distinguish between IBS and IBD.82–84 Surveys indicated that up to 12% of primary care visits were comprised of IBS-related complaints and accounted for 28% of referrals to gastroenterologists.85,86 Because symptoms often overlap, early IBD may be inappropriately labeled as IBS. At least one study reported that more than 25% of patients with Crohn’s disease had been misdiagnosed as having IBS in the prodromal stages of their disease.87 Attempts to define organic disease in patients with IBS inevitably results in the application of more invasive diagnostic imaging procedures and escalated health care costs.5
Calprotectin has been found to correlate closely with IBD activity88 and provides a highly sensitive, specific, and noninvasive alternative for assessing inflammatory activity in IBD.84,89 Fecal calprotectin levels have been shown to correlate significantly with histologic and endoscopic assessment of disease activity in ulcerative colitis90 and with indium-111–labeled white blood cells (the gold standard for assessing gut inflammation) in patients with Crohn’s disease.91 In one report, more than 95% of patients with IBD had elevated fecal calprotectin levels.92
Calprotectin has been used to predict relapse in patients with established IBD and to evaluate the effectiveness of therapeutic regimens as well.68,93,94
Because severity of inflammation is indicated by varying levels of calprotectin, treatment options and urgency of diagnostic intervention vary as well (see Table 27-2).
TABLE 27-2 Calprotectin Levels, Inflammation Severity Clinical Intervention
<50 mcg/g | No inflammation |
50-120 mcg/g | Mild inflammation |
120-250 mcg/g | Moderate inflammation |
>250 mcg/g | Severe inflammation |
Infectious Agents
Helicobacter pylori
Helicobacter pylori remains a prevalent worldwide infection, with infection rates in middle-aged adults reaching more than 80% in areas of low socioeconomic status and 20% to 50% in most industrialized countries.95 H. pylori continues to be a causal link for the development of peptic ulcer disease (an estimated 10% to 20% lifetime risk in infected individuals),96 as well as a variety of other GI concerns ranging in severity from dyspepsia to gastric malignancy.97,98
HpSA diagnostics have been approved by the U.S. Food and Drug Administration for the detection of H. pylori pre- and posteradication therapy. This biomarker indicates the presence of H. pylori antigens shed directly into the stool, and provides increased ease of clinical use as well as diagnostic performance characteristics comparable to urea breath testing in both adult and pediatric populations.99,100 It has been shown to accurately indicate treatment failure at 7 days after completion of eradication therapy (compared with 4 weeks for urea breath testing),100–102 an important consideration for directing additional clinical intervention in patients who remain symptomatic or develop recurrent symptoms posttherapy.
Although PCR testing for H. pylori is currently offered as a noninvasive diagnostic alternative to endoscopy and biopsy as well, concerns have been raised about the high degree of false-positive results with this methodology.103,104
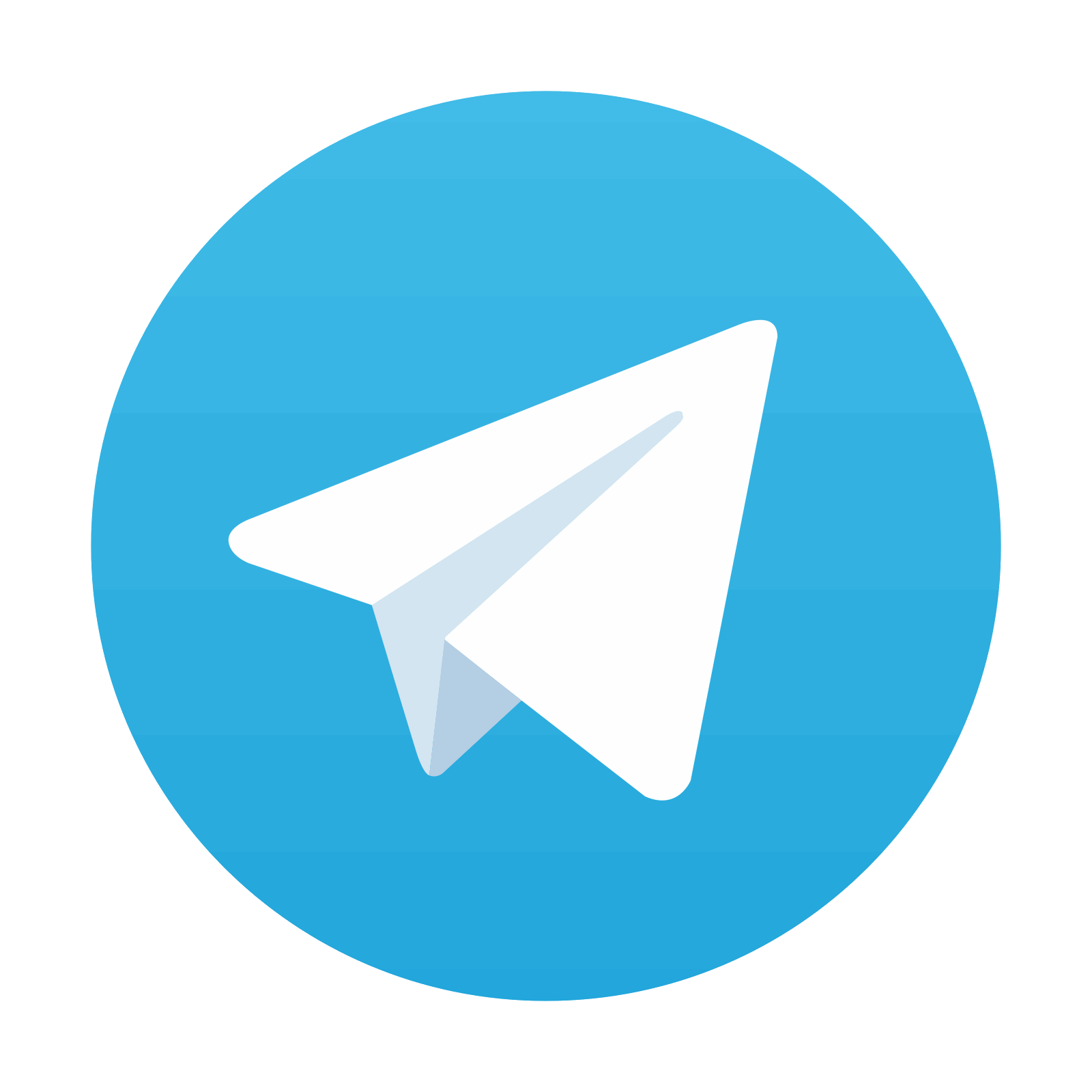
Stay updated, free articles. Join our Telegram channel

Full access? Get Clinical Tree
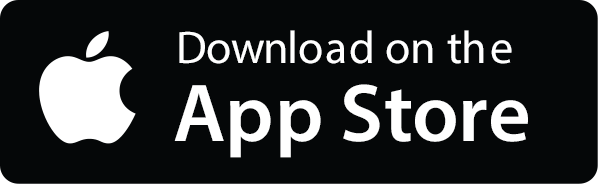
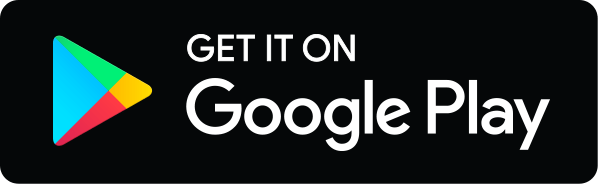