Chapter 69 Beta-Carotene and Other Carotenoids
Introduction
More than 600 carotenoids have been characterized, but only about 30 to 50 are believed to have vitamin A activity, and some 20 are found in human plasma and tissues. Biological activity of a carotenoid has historically been considered synonymous with its corresponding vitamin A activity. Beta-carotene has been termed the most active of the carotenoids due to its higher provitamin A activity (Figure 69-1). However, recent research suggests that this function of carotenoids has been overemphasized, since they have been found to exhibit many other important physiologic activities. For a carotenoid to have vitamin A activity, it must have an unaltered β-ionone ring with an attached polyene side chain containing 11 carbon atoms. In contrast, apocarotenoids are compounds that have been shortened by the removal of at least one end of the molecule beyond a designated location (e.g., β-Apo-8′-carotenal has been cleaved at the 8’ carbon). Apocarotenes and xanthophylls have reduced or no vitamin A activity, but many have been shown to have significant antioxidant and physiologic benefits.1–3 Also, approximately 25% of whites do not convert β-carotene to vitamin A.
Dietary Sources
Among the richest sources of carotenes are green leafy vegetables. The carotenoids in green plants are found in the chloroplasts with chlorophyll, usually in complexes with a protein or lipid. Beta-carotene is the predominant form in most green leaves. In general, the greater the intensity of the green color, the greater the concentration of β-carotene. Orange-colored fruits and vegetables (e.g., carrots, apricots, mangoes, yams, squash) typically have higher concentrations of provitamin A carotenoids, the provitamin A content again paralleling the intensity of the color. Yellow vegetables have higher concentrations of xanthophylls and, hence, lower provitamin A activity. In orange and yellow fruits and vegetables, β-carotene concentrations are high, but other provitamin A carotenoids typically predominate. Red and purple vegetables and fruits such as tomatoes, red cabbage, berries, and plums contain large portions of non–vitamin A–active pigments, including flavonoids. Legumes, grains, and seeds are also significant sources of carotenoids. Carotenoids are also found in various animal foods such as salmon and other fish, egg yolks, shellfish, milk, and poultry. Carotenoids are also frequently added to foods as colorants. Table 69-1 lists carotenoids with provitamin A activity found in common food sources, whereas Table 69-2 lists some important carotenoids that have no vitamin A activity. The structures of two of these, lycopene and zeaxanthin, are illustrated in Figures 69-2 and 69-3.
TABLE 69-1 Provitamin A Carotenoids and Food Sources
CAROTENOID | VITAMIN A ACTIVITY (%) | FOOD SOURCES |
---|---|---|
Beta-carotene | 100 | Green plants, carrots, sweet potatoes, squash, spinach, apricots, green peppers |
Alpha-carotene | 50-54 | Green plants, carrots, squash, corn, watermelons, green peppers, potatoes, apples, peaches |
Gamma-carotene | 42-50 | Carrots, sweet potatoes, corn, tomatoes, watermelons, apricots |
Beta-zeacarotene | 20-40 | Corn, tomatoes, yeast, cherries |
Cryptoxanthin | 50-60 | Corn, green peppers, persimmons, papayas, lemons, oranges prunes, apples, apricots, paprika, poultry |
Beta-apo-8’-carotenal | 72 | Citrus fruit, green plants |
Beta-apo-12’-carotenal | 120 | Alfalfa meal |
TABLE 69-2 Non–Provitamin A Carotenoids and Food Sources
CAROTENE | VITAMIN A ACTIVITY (%) | FOOD SOURCES |
---|---|---|
Lycopene | 0 | Tomatoes, carrots, green peppers, apricots, pink grapefruit |
Zeaxanthin | 0 | Spinach, paprika, corn, fruits |
Lutein | 0 | Green plants, corn, potatoes, spinach, carrots, tomatoes, fruits |
Canthaxanthin | 0 | Mushrooms, trout, crustaceans |
Crocetin | 0 | Saffron |
Capsanthin | 0 | Red peppers, paprika |
According to a detailed analysis of the levels of carotenoids in 120 fruits and vegetables, lycopene is found in few.4 Table 69-3 lists the foods that contained lycopene. These values indicate that lycopene levels are retained in food processing.
TABLE 69-3 Lycopene Content of Common Foods
FOOD | LYCOPENE (mg/100 g) |
---|---|
Apricot, canned | 0.06 |
Apricot, dried | 0.8 |
Grapefruit (pink and raw) | 3.4 |
Guava juice | 3.3 |
Tomato, raw | 3.1 |
Tomato juice, canned | 8.6 |
Tomato paste, canned | 6.5 |
Tomato sauce, canned | 6.3 |
Watermelon, raw | 4.1 |
Metabolism
Absorption
• The presence of fat, protein, and antioxidants in the food
• The presence of bile and a normal complement of pancreatic enzymes in the intestinal lumen
The absorption efficiency of dietary vitamin A is usually quite high (80% to 90%), with only a slight reduction in efficiency at high doses. In contrast, β-carotene’s absorption efficiency is much lower (40% to 60%), and it decreases rapidly with increasing dosage.1–3 Carotene supplements are better absorbed than the carotenes from foods.5 Supplying only β-carotene, whether natural or synthetic, may be exerting a detrimental effect to the absorption of other carotenes. For example, β-carotene supplementation has been shown to inhibit the absorption of lutein.6 Studies in humans have shown that with β-carotene supplementation, the absorption of lutein can drop by more than 50%.7 Interestingly, the absorption of lutein from vegetables is five times greater than that of β-carotene.8
Transformation in the Intestinal Mucosa
As stated earlier, of the more than 600 carotenoids that have been reasonably well characterized, only about 30 to 50 are believed to have provitamin A activity. However, carotenoids provide the majority of dietary vitamin A. Provitamin A carotene conversion to vitamin A depends on diverse factors9:
The conversion diminishes as carotene intake increases and when serum retinol levels are adequate.10 Beta-carotene and other provitamin A carotenes were originally believed to be cleaved by carotene dioxygenase at the 15,15’ double bond, which would yield two molecules of all-trans retinal. Current belief, however, is that the dioxygenase enzyme nonspecifically attacks any one of the double bonds of the β-carotene, resulting in the formation of a corresponding apo-β-carotenal or retinal.11 The apocarotenal formed can either be degraded to retinal or absorbed. The retinal formed is then converted to retinol by retinaldehyde reductase.
Transport, Storage, and Excretion
No specific carrier protein exists in the plasma for carotenoids. These compounds are typically transported in human plasma in association with the plasma lipoproteins, particularly by low-density lipoprotein (LDL). As a consequence, patients with high serum cholesterol or LDL levels tend to have high serum carotene levels. The concentrations found in the plasma usually reflect the dietary concentration, with β-carotene typically comprising only 20% to 25% of the total serum carotene level.12
Carotenes may be stored in adipose tissue, the liver, other organs (the adrenals, testes, and ovaries have the highest concentrations), and the skin (Table 69-4). Deposition in the skin results in carotenodermia. This is a benign (and probably beneficial) state. Carotenodermia not directly attributable to dietary intake or supplementation, however, may indicate a deficiency in a necessary conversion factor (e.g., zinc, thyroid hormone, vitamin C, or protein).
TABLE 69-4 Distribution of Carotenoids in Some Human Tissues (milligrams per kilogram)
TISSUE | CAROTENOIDS | BETA-CAROTENE |
---|---|---|
Adrenal | 20.1 ± 11.9 | 10.8 ± 5.5 |
Liver | 8.3 ± 21.3 | — |
Testis | 5 ± 7.7 | 4.7 ± 2 |
Fat | 3.9 ± 6 | 1.3 ± 1.1 |
Pancreas | 2.3 ± 1.2 | 1.1 ± 1 |
Spleen | 1.6 ± 2.2 | 1.2 ± 0.5 |
Lung | 0.6 ± 1.0 | — |
Thyroid | 0.6 ± 0.4 | — |
Physiologic Roles
Antioxidant Activity
In general, carotenes exert significant antioxidant activity, whereas the antioxidant activity of vitamin A is relatively minor.13 However, its antioxidant actions are specific in that they are involved primarily in scavenging singlet molecular oxygen and to a much lesser extent peroxyl radicals. The efficacy of the various carotenoids for physical quenching is related to the number of conjugated double bonds present in the molecule determining their lowest triplet energy level. The most efficient singlet oxygen quenching carotenoid is the open-ring carotenoid lycopene.
The antioxidant activity of carotenes is thought to be responsible for their anticancer effects. Because aging is associated with free radical damage, a hypothesis developed that carotenes may also protect against aging as well. Evidence seems to support this hypothesis. It appears that tissue carotenoid content is the most significant factor in determining maximal life span potential (MLSP) of mammalian species (r = 0.835 for 12 mammalian species, and for primates alone, r = 0.939).14 For example, human MLSP of approximately 90 years correlates with a serum carotene level of 50 to 300 mg/dL, whereas other primates, such as the rhesus monkey, have an MLSP of approximately 34 years, correlating with a serum carotene level of 6 to 12 mg/dL.
Although β-carotene has received most of the attention, many carotenes that have either low or no vitamin A activity exert much greater protection compared with β-carotene. For example, β-carotene generates vitamin A much more efficiently than α-carotene, but α-carotene is approximately 38% stronger as an antioxidant and 10 times more effective in suppressing liver, skin, and lung cancer in animals compared with β-carotene.15 Even more powerful are lycopene, lutein, and astaxanthin.16–21 Studies have shown lycopene to exhibit the highest overall singlet oxygen quenching of the carotenoids thus far studied.22 Its activity is roughly double that of β-carotene. Furthermore, lycopene exerts even more impressive anticancer effects.17,18
The data were obtained from a series of hospital-based studies on various cancers of the digestive tract from 1985 to 1991.23 Frequency of consumption of raw tomatoes was divided into four levels: fewer than two; three to four; four; five to six; and more than seven servings per week. Results showed a consistent pattern of protection by high intake of raw tomatoes in all examined cancer sites of the digestive tract. The degree of protection was similar to, but somewhat more marked than, those afforded by green vegetables and fruit studies carried on in the same areas. The results supported the findings of other researchers who found, for example, a 40% reduction in the risk of esophageal cancer by simply consuming one serving of raw tomatoes per week and a 50% reduced rate for cancers of all sites among elderly Americans reporting a high tomato intake. These results suggest that increasing dietary lycopene levels may be a significant protector against cancer.17,18
Immune System
Carotenes have demonstrated significant effects in enhancing immune function. Some of these effects are probably related to an ability to prevent stress-induced thymic involution, as well as promote thymus growth and function and increase interferon’s stimulatory action on the immune system.24 Interferon is a powerful immune-enhancing compound that plays a central role in protection against viral infections.
Reproduction
Beta-carotene reportedly has a specific effect in fertility distinct from its role as a precursor to vitamin A.25–27 In bovine nutritional studies, cows fed β-carotene–deficient diets exhibited delayed ovulation and an increase in the number of follicular and luteal cysts.25,26 The corpus luteum has the highest concentration of β-carotene of any organ measured.27 The carotene cleavage activity changes with the ovulation cycle, with the highest activity occurring during the midovulation stage. It has been speculated that a proper ratio of carotene to retinol must be maintained to ensure proper corpus luteum function.
As the corpus luteum produces progesterone, inadequate corpus luteum function could have significant deleterious effects. Inadequate corpus luteum secretory function is one of the characteristic features of infertile or irregular menstrual cycles, or both.28 Furthermore, an increased estrogen-to-progesterone ratio has been implicated in various clinical conditions, including ovarian cysts, premenstrual tension syndrome, fibrocystic breast disease, and breast cancer.29 Because supplemental β-carotene given to cows significantly reduced the incidence of ovarian cysts (42% in control group vs 3% in the β-carotene group), it may have a similar effect in humans.26,27 Another bovine condition that benefited from increased dietary β-carotene levels was cystic mastitis.28 Apparently dairy farmers have a greater appreciation of β-carotene than do many nutritionists. Of course, there are significant financial reasons, because the annual monetary loss from bovine mastitis in the United States has been estimated at 1.5 to 2 billion dollars, and ovarian cysts represent the major cause of infertility in cattle.
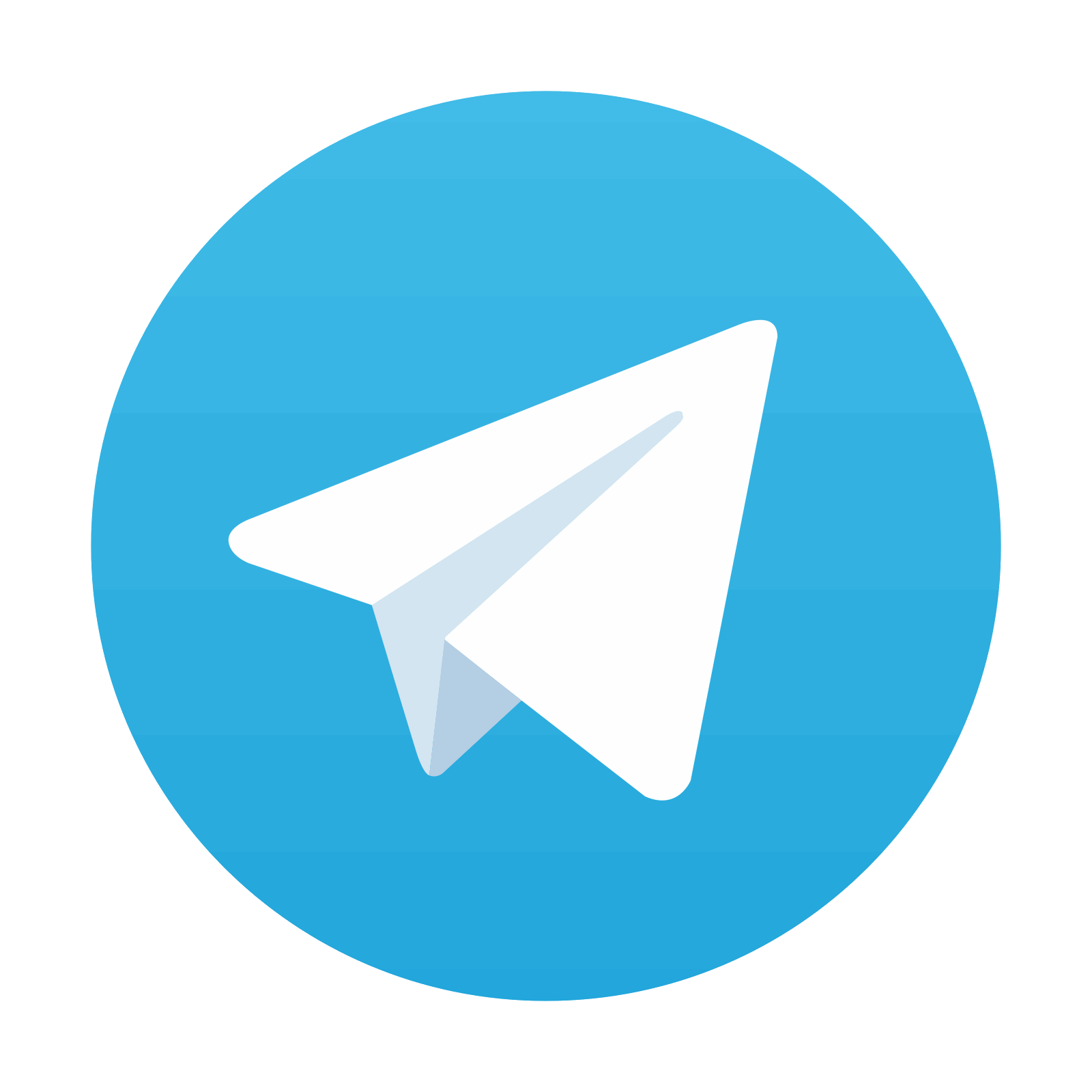
Stay updated, free articles. Join our Telegram channel

Full access? Get Clinical Tree
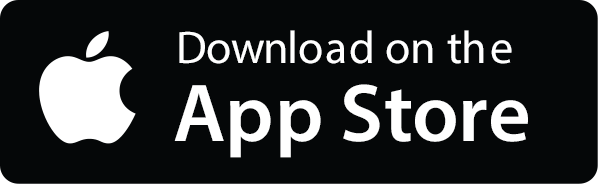
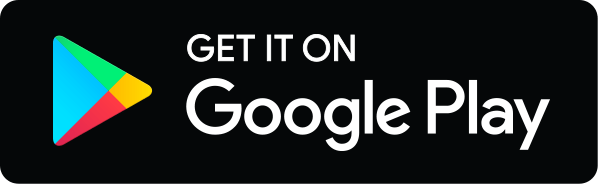