Total Ankle Replacement
A Historical Perspective
keywords
• Ankle • Replacement • Design • Prosthesis • Arthritis • Implant
Introduction
Total ankle replacement was first attempted in the early 1970s as an alternative to ankle arthrodesis.1–12 This procedure gave surgeons the opportunity to provide or preserve ankle motion for patients with end-stage ankle arthritis or posttraumatic arthritis (Figs. 1 and 2). During the 1970s, the devices that were available for ankle replacement implantation were widely criticized. This was primarily due to very poor patient outcomes with regard to the initial wave of implants that were available on the market.1,3–5,7,8,13,14 At times, this resulted in catastrophic failures with regard to the procedure.1,8,9,15 It was not until the 1980s and 1990s that interest was renewed as new designs that addressed the shortcomings of their predecessors were brought to the marketplace.4,5,7,15 In the years between the 1990s and today, ankle replacement has gained popularity to the point that it is becoming the viable, accepted alternative for certain patients with end-stage ankle arthritis.3–5,7,16–28
Ankle joint biomechanics
The nature and function of the ankle joint itself necessitated improved implant design from the start.16,17 The biomechanics of the ankle joint is unique because of its topography, its load-bearing characteristics, and its surrounding soft tissue tethers. The talocrural and subtalar joints endure forces up to five times body weight during gait, which must be observed over a much smaller contact area than the hip and knee of the ipsilateral extremity. The smaller size of the joint leads to a higher resultant moment and higher compressive force.16,17,29–31 The ankle joint also accepts two to three times the forces experienced by the hip or the knee, but only has one-third of the surface area to dissipate this load. Of greater concern is the available bone stock because the ankle joint has far less bone stock available for implantation of prosthetic replacement compared with the hip or the knee.16,17,31
Arthrodesis versus arthroplasty
There are many reports and studies by various investigators comparing ankle arthrodesis versus total ankle replacement. Haddad, SooHoo, Piriou, and Hintermann and their colleagues32–36 all compared the satisfaction rates of the fused ankle versus ankle replacement versus healthy ankle joints themselves. The Barg and Hintermann36 study showed that patients with fused ankles had 3% greater oxygen consumption than the ankle replacement group—yet another reason or impetus for developing an improved ankle replacement design. The study showed the stress on the additional joints when the ankle joint is fused and the impact of ankle fusion in significantly comorbid patient populations. The study also found the satisfaction rates of patients with replacements were 27% higher than patients who had ankle fusion. Piriou and colleagues34 found that neither group (arthrodesis or arthroplasty) had normal movement restored. However, “the arthrodesis group had a faster gait; the arthroplasty group had a reduction of limping with gait.” Piriou and colleagues34 also found that the arthroplasty could demonstrate more of a symmetric gait and timing when compared with the arthrodesis group.
Historical overview and the first generation of ankle implants
From a historical standpoint, there are three accepted generations of ankle replacement designs.37–42 Despite this assessment, the categories on which these are based are market introduction to the general populace. Often, implants may straddle two or more generations. Examples include Scandinavian Total Ankle Replacement (STAR, Morrisville, PA, USA), TNK First Generation (Japan), and the Agility Ankle (DePuy, Warsaw, IN, USA).
In the early and middle 1970s reports appeared of early success with first generation implants in 80% to 85% of patients. In 11 reports that included 346 arthroplasties, good or fair results were reported in 83% and failures in 17%, at a mean follow-up of fewer than 5 years.43–46
Because of these promising results, the potential for total ankle arthroplasty as a viable surgical alternative peaked interest and the indications for the procedure were expanded to include patients who were younger with active lifestyles. Unfortunately, long-term follow-up reviews of early series of total ankle arthroplasties showed poor long-term results, especially in younger patients with isolated traumatic arthritis. In later reports, in which the average follow-up was longer than 5 years, failure occurred in 35% to 76% of arthroplasties.8,43,47,48
First-generation implants
Marotte and Lord first implemented their reverse hip design for ankle replacement in the early 1970s (Figs. 3–5) with varied results in terms of satisfaction and success. Of the 25 implanted, only 7 were considered to be successful.49 Consequently, the design was abandoned to the more predictable arthrodesis procedure. This initial design lost favor and new designs began to emerge. Designs such as the Mayo,1,8,15 TNK First Generation,50–58 and the first Buechel-Pappas Low Contact Stress59,60 replacements were also received with skepticism during this same period. The MAYO success results were probably the most unsatisfactory of the first generation of ankle replacements between 1974 and 1984. Overall implant survival rate was 79% at 5 years, 65% at 10 years, and 61% at 15 years, with a revision rate of 41% for persistent pain according to Kitaoka and colleagues.1 Unfortunately, total ankle replacement as a viable surgical procedure hit yet another stumbling block.
The reasons for the initial poor results from total ankle replacement included use of cemented designs; a unappreciated or lack of recognition of coronal malalignment; inferior metallurgy or implant composition; too much surgical “eyeballing,” which led to overaggressive bone resection; and, finally, not fully understanding which cases were ideal for the procedure. These early mistakes led researchers and surgeons to refine implant design in hopes of increased success rates for total ankle replacement.1,7 To overcome the negative stigma associated with early total ankle replacement, these designs had to show vast improvement over those that had failed during the 1970s. It was these early failures that set the stage for the second generation of implants. An overall industry and surgeon-related push was made to come up with designs that could reproduce near anatomic ankle motion. These designs had to be supportive and resist implant failure. New designs also required improved metallurgy for support.
Ankle Anatomy Relative to Implant Design Strategy
In terms of ankle anatomy and biomechanics, the improvement of ankle replacements had to address a few issues, including subsidence or loosening of the components on the talar or tibial side—the main reasons for implant failure.16,17 Taking into account the overall long-term failures of the first generation of total ankle implants and a closer look at the structural shortcomings of the joint itself were instrumental in providing more robust and stable designs.
Tibial Component
In 1985, Hvid and colleagues61 showed the greatest bone strength is in the posteromedial tibial plafond (Fig. 6) and the region of least bone strength is in the anterolateral tibia. Implant designs improved in their ability to disperse the weight load from the distal tibial plafond away from the weakest points and toward the stronger posteromedial regions.61 This forced transmission is eccentric and essentially loads on the cortical rim of the outside weightbearing surface as described by Trouillier and colleagues62 in 2003 (Fig. 7). More current designs have addressed this issue in terms of their implantation or the stress applied across the implant surface from the distal tibia in hopes of preventing subsidence.63,64
Talar Component
The talar component implants have also evolved with new designs. Initial designs called for table-top cutting of the talus and nonisometric load and nonminimizing stress distribution. This practice led to subsidence and sinking of the talar components into the soft talar bone located just inside the anatomic talar wall perimeter. Newer designs have sought to maximize stress distribution while minimizing stress load by making more anatomic designs. The newer talar component designs not only disperse the vertical load to the outer rim of the talus where the stronger bone is found, but also prevent rotation that can lead to edge-loading and subsidence (Fig. 8).
Second-generation implants
The first second-generation total ankle replacements were designed or developed between 1979 and 2000. These include, but are no limited to, the first generation of STAR, the Agility Ankle (designed by Dr Frank Alvine; Food and Drug Administration [FDA] approval in 1995), and the Buechel-Pappas Low Contact Stress design (limited implantation) (Fig. 9).
In 2002, Easley and colleagues22 stated that four second-generation total ankle arthroplasty designs showed reasonable functional outcomes: (1) STAR, (2) the Agility Ankle, (3) the Buechel-Pappas Total Ankle Replacement, and (4) the TNK ankle. Although the Agility Ankle is noted here, intermediate results are promising but should be treated with caution. In 2004, Knecht and colleagues65–69 stated that arthrodesis of the tibiofibular syndesmosis impacts the radiographic and clinical outcomes with the Agility Ankle. The relatively low rates of radiographic hind-foot arthritis and revision procedures at an average of 9 years after the arthroplasty are encouraging. Replacement with the Agility Ankle was found to be a viable and durable option for the treatment of ankle arthritis in selected patients.15,70–73
The Agility Ankle can be classified as a fixed, semiconstrained implant because the poly ethylene is fixed to the tibial tray (see Fig. 8). It is also considered a semiconstrained implant because it does not completely rely on soft tissue adaptability, although it does take it into account. The Agility Ankle uses external fixation to allow for exposure to the ankle joint during implantation. This design requires performing a distal fibular roll out on the lateral ankle joint side of the implant, as well as a synostosis of the distal tibia and fibula, which allows for appropriate component seating. The talar component is flanged to disperse vertical load force laterally to talar cortical rim, thereby decreasing the risk of subsidence. This talar modification has proved to be more successful then the previous nonflanged design of the early Agility Ankle (Figs. 10 and 11).
STAR (see Fig. 11, Figs. 12–16), designed by Dr Kofoed in 1979, has also undergone some redesigns and was introduced in the United States in 2010 (SBI, Small Bone Innovations, Morrisville, PA, USA). The key features are its double-keeled distal tibial component, the sagittal design of the talar component, and its mobile bearing polyethylene design to decrease excessive contact and minimize stress fatigue. This also allows dynamic motion via the prosthesis by the mobility of the actual polyethylene meniscal component. The design has a radio opaque filament, which resides within the meniscal component. This design feature is helpful in demonstrating polyethylene fracture without operative determination.74,75 Alignment is accomplished with a sagittal alignment guide that is pinned to the anterior tibia (see Fig. 13). This guide allows for accurate resection of the distal tibia as well as mediolateral and rotational translation to ensure appropriate postoperative alignment (see Fig. 12).
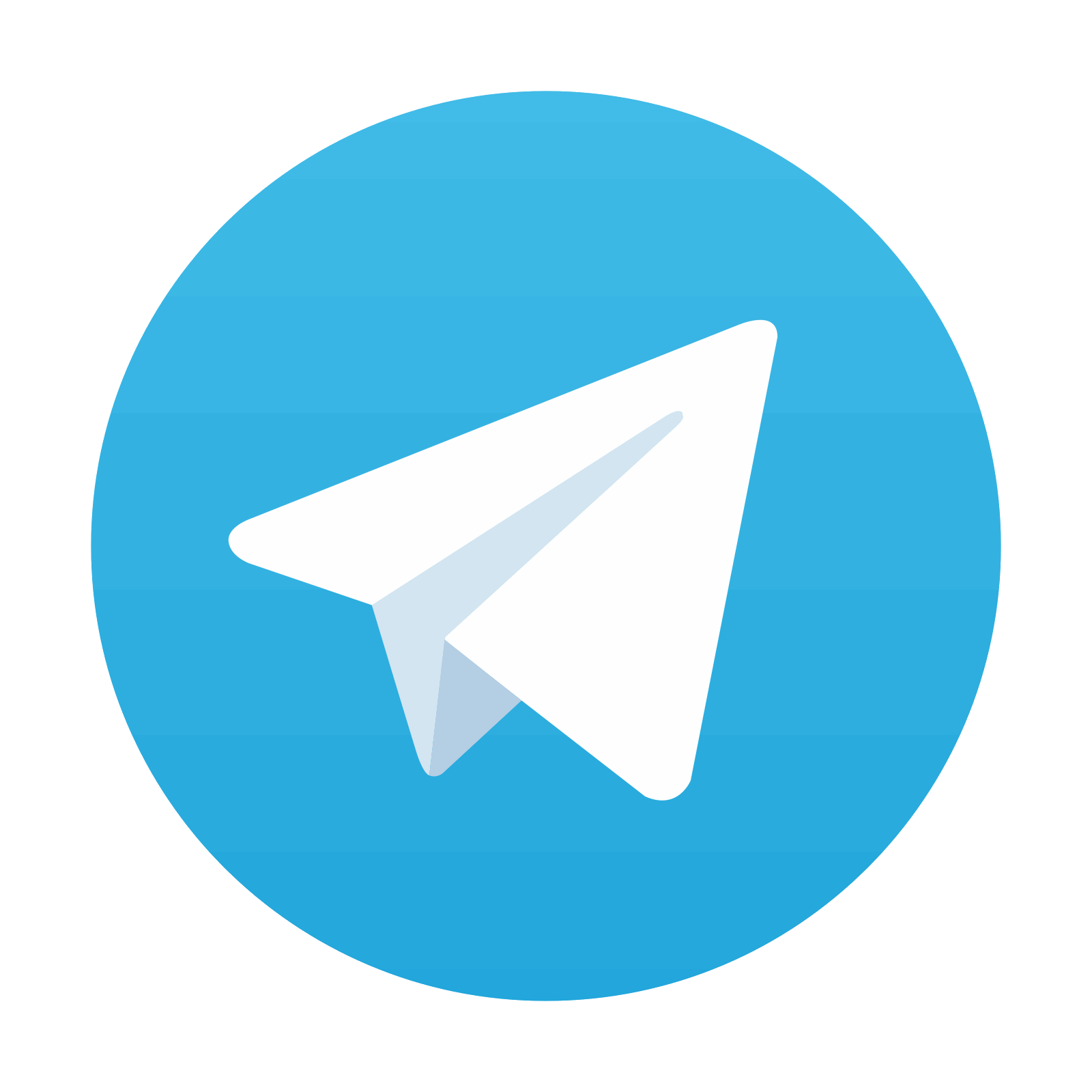
Stay updated, free articles. Join our Telegram channel

Full access? Get Clinical Tree
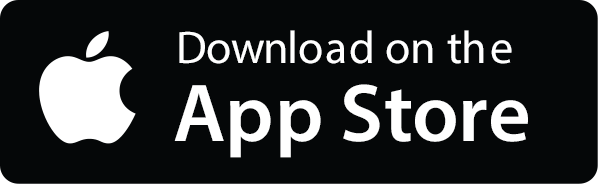
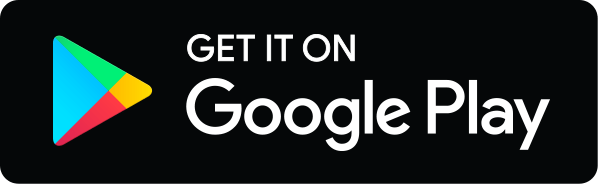