Fig. 1.1
(a) Anterior view of a right cadaveric knee demonstrating the anterior cruciate ligament (ACL), posterior cruciate ligament (PCL), and lateral meniscal anterior root attachment (LARA). (b) Sagittal cross section of a right femur demonstrating the anteromedial (AM) and posterolateral (PL) bundle of the ACL in relation to resident’s ridge
Biomechanically, the ACL is the primary static stabilizer to anterior tibial translational forces [20–26], and it resists internal and external tibial rotation in flexion and extension [16, 27]. Cadaveric studies have demonstrated that in extension the PLB is taut and experiences the greatest force, whereas the AMB is taut in flexion with the highest transmission of forces at 60° [23]. In addition to resisting external forces, sensory and mechanoreceptors within the ligament contribute to proprioception and also assist in initiating important secondary stabilizing muscular reflexes [28, 29].
Loss of proprioception following TKA is one factor contributing to patient dissatisfaction [1], which may be avoided in cruciate-retaining knee implants. Furthermore, sagittal plane kinematics have been preserved in 10-year follow-up studies after UKA [30], which contrasts with many current TKA designs that can result in anterior tibial subluxation in full extension [31–33] and paradoxical anterior femoral translation during flexion [34].
1.3 Posterior Cruciate Ligament
The posterior cruciate ligament (PCL) is an intra-articular, extra-synovial [35] ligament comprised of two bundles. There is a larger anterolateral bundle (ALB) and a smaller posteromedial bundle (PMB) [16, 36–38], which are named for their respective attachments onto a depression on the posterior aspect of the tibia. An important landmark of the tibial PCL attachment is an anterior relation with the shiny white fibers of the posterior horn of the medial meniscus [39]. The center is located 1.3 ± 0.5 mm proximal to the bundle ridge, which is a bony prominence separating the ALB and PMB with an average distance of 8.9 ± 1.2 mm between their individual centers [39]. At the posterior aspect of the tibial plateau, a bony ridge marks the distal border of the PCL [40]. The two PCL bundles can often be distinguished more easily at their attachment to the lateral aspect of the medial femoral condyle, adjacent to the articular cartilage margin. The ALB is 12.1 ± 1.3 mm proximomedial to [39] and twice the size of the PMB [41]. Additionally, there are two meniscofemoral ligaments, an anterior (Humphry) and posterior (Wrisberg) that can often be found adjacent to the PMB at its femoral attachment [42]. Both of these structures may be present in up to 60% of knees, while 95% contain at least one [43].
The anatomy of the posterior cruciate ligament is relevant for cruciate-retaining prosthetic designs for which potential advantages are preservation of bone stock, knee kinematics that better resemble the native, improved proprioception, femoral rollback on the tibia during extension, and better prosthesis stabilization, with the PCL preventing anterior translation of the femur on the tibia. In this regard, when performing the tibial cut, the surgeon should be extremely diligent not to damage the PCL attachment which may be spared in the majority of patients by performing a tibial bony cut of 4 mm or less when a posterior slope of 3–5° is used [44] (Fig. 1.2)


Fig. 1.2
(a) Anteromedial view of a cadaveric knee demonstrating bony landmarks of the femoral attachments of the anterolateral bundle (ALB) and posteromedial bundle (PMB). (b) Posterior view of a cadaveric right knee demonstrating bony and soft tissue landmarks of the posterior cruciate ligament (PCL)
Biomechanically, the two bundles of the PCL provide codominant posterior translational stability [45, 46]. Secondarily the PCL resists rotational forces, particularly internal rotation between 90° and 120° [47, 48]. The individual bundles behave complementary at all flexion angles, demonstrating relative reciprocal changes in length, tension, and fiber orientation. At full extension, the PMB is taut and provides greater resistance to posterior tibial translational force [47, 49], becoming shorter and more horizontal with flexion [50]. Conversely, the ALB is longer and taut in 90° of flexion [51–54], but is also more vertical [50].
Understanding this relationship between tension, length, and orientation is the basis for codominant force resistance throughout knee motion [55] and helps elucidate the need for an anatomic double-bundle PCL when a reconstruction is needed. In cases where the cruciates are sacrificed in a TKA, the posterior cam-post-stabilization creates equivalent but nonanatomic medial and lateral femoral condyle posterior translation, which increases wear at the post and decreases internal tibial rotation [34]. A PCL-retaining TKA can lead to paradoxical anterior translation of the femoral condyles in flexion, which may be due to the vertical position the PCL adopts in the absence of the ACL [56]. Bicruciate-retaining TKA has shown good midterm results, and as acute injury reconstruction has shown, a shift toward more anatomic reconstruction leads to better results and improved kinematics.
1.4 Posterolateral Corner
The posterolateral corner (PLC) of the knee is comprised of three main primary lateral stabilizing structures: the fibular collateral ligament (FCL), popliteus tendon (PLT), and popliteofibular ligament (PFL) [57–61]. PLC injuries are present in nearly 16% of all knee ligament injuries [62]; however, the FCL, which is the primary varus stabilizer [52, 63, 64], is damaged in only 23% of PLC injuries [65] which may make identification difficult. The FCL attaches 1.4 mm proximal and 3.1 mm posterior to the lateral epicondyle [61]. It extends distally, with an average length of 7 cm [66], attaching 8.2 mm posterior to the anterior margin of the fibular head and 28.4 mm distal to the tip of the fibular styloid [61].
The popliteus muscle originates on the posteromedial tibia, becoming tendinous intra-articular as it courses superiorly, and runs deep to the FCL attaching 18.5 mm anterior to it with the knee flexed at 70°. In the lateral third of the popliteal fossa, the musculotendinous junction of the popliteus gives rise to the PFL which has two divisions. The larger posterior division attaches 1.6 mm distal to the posteromedial aspect of the tip of the fibular styloid process, and the smaller anterior division attaches 2.8 mm distal to the anteromedial aspect of the tip of the fibular styloid process [61].
In addition to the three main lateral knee stabilizers, a number of secondary structures provide static and dynamic resistance to the PLC. The mid-third lateral capsular ligament is a capsular thickening that attaches to the lateral epicondyle anterior to the popliteus and to the tibia just posterior to Gerdy’s tubercle. It may function as a secondary varus stabilizer [61] and has a meniscofemoral and meniscotibial ligament component [67, 68]. The coronary ligament is also a component of the capsule found both medially and laterally attaching the menisci to their respective tibial plateau [69]. The lateral gastrocnemius tendon is the next important structure, because it is less frequently injured and can be used as a landmark during surgical reconstruction [70]. It is found posterior to the femoral FCL attachment along the supracondylar process and courses distally, fusing with the medial gastrocnemius and the soleus to form the sural triceps muscle. Additionally, there are two heads of the biceps femoris that attach to the fibula and enclose the distal attachment of the FCL. The short head of the biceps femoris has two arms that attach along the lateral aspect of the fibular styloid. The capsular arm has a distal thickening that extends vertically from the fabella to the fibular styloid to form the fabellofibular ligament. The fabella is a sesamoid bone (or cartilaginous analogue the rest of the time) that is found within the proximal lateral gastrocnemius tendon in approximately 30% of individuals [71]. The long head of the biceps also has two arms: a direct arm that inserts onto the posterolateral aspect of the fibular head and an anterior arm that is a crucial access point during FCL reconstruction as it fans out superficial to the FCL [70, 72].
The peroneal nerve, which can be damaged in up to one-third of PLC injuries [65, 73], runs deep to the biceps femoris and must be identified surgically where it emerges 1–2 cm proximal to the fibular head before coursing around the fibular neck and dividing into superficial and deep branches [70, 72]. After a biceps tendon avulsion off the fibula, the nerve may migrate within the soft tissue of the posterolateral compartment, and additional care during dissection should be taken. Finally, the broad fascia of the iliotibial band (ITB) is the most superficial layer of the lateral aspect of the knee, covering all of the lateral femoral structures as it attaches from the anterior superior iliac spine onto Gerdy’s tubercle on the anterolateral aspect of the tibia.
Opposing convex articular surfaces of the lateral femoral condyle and lateral tibial plateau create inherent bony instability in the lateral knee [74, 75]. Consequently, hyperextension and noncontact varus stress can cause injury, as well as a direct blow to the anteromedial knee [65]. Restraint to varus force is primarily accomplished by the FCL, particularly at 30° of flexion when there is less contribution from the other PLC structures that lend secondary support [65, 72]. The FCL also provides external rotational stability between 0° and 30° of flexion, along with the PLT in greater flexion and the PCL beyond 90° [76]. The PLT and the other PLC structures also provide secondary stabilization for anteroposterior tibial translation [52, 77, 78] and minor secondary restraint to internal rotation; however, those forces are controlled primarily by the ACL in low flexion angles and the anterolateral ligament in higher flexion [70, 79]. Although PLT release may be useful for lateral flexion gap tightness [80], resection can affect gap balancing and stability in TKA [81], and iatrogenic laceration results in decreased functional scores 2–3 years postoperatively following TKA [82]. Furthermore, overaggressive lateral structure releases have been implicated in TKA dislocations [83], and intraoperative injury to these structures can result in acute instability in flexion [84], warranting a preservation of the native anatomy (Fig. 1.3).


Fig. 1.3
Lateral view of a right cadaveric knee demonstrating isolated fibular collateral ligament (FCL) with attachments to the lateral femoral epicondyle (LE) and the fibular head, popliteus muscle and tendon, popliteofibular ligament (PFL), and proximal tibiofibular joint (PTFJ)
1.5 Medial/Posteromedial Structures
The medial collateral ligament (MCL) can be divided into a deep (dMCL) and superficial (sMCL) component. The two divisions of the MCL, along with the posterior oblique ligament (POL), provide the primary stability to the medial knee [85–88]. The MCL is a well-vascularized, extracapsular ligament, which grants it superior intrinsic healing capabilities compared to the anterior cruciate ligament [89–92]. Evidence of growth factor bioactivity and healing following injury has validated these early observations and provided a foundation for future treatment modalities [93–95].
The sMCL is recognized as the largest medial structure. It has a single femoral attachment 3.2 mm proximal and 4.8 mm posterior to the medial epicondyle and two distinct but synergistic tibial attachments [89]. The distal tibial division blends deep to the pes anserine bursa and has a bony attachment 61.2 mm distal to the joint line. The proximal attachment blends with the soft tissue of the anterior arm of the semimembranosus tendon 12.2 mm distal to the tibial joint line.
Additionally, fibrous extensions from the distal aspect of the semimembranosus tendon blend with the posteromedial aspect of the joint capsule to form three POL arms. The superficial and capsular arms are thin fascial expansions. The central (tibial) arm is the largest and thickest reinforcement of the posteromedial joint capsule, and it attaches on the femur 7.7 mm distal and 6.4 mm posterior to the adductor tubercle or 1.4 mm distal and 2.9 mm anterior to the gastrocnemius tubercle.
Finally, the medial aspect of the joint capsule thickens to form two components of the dMCL. The meniscofemoral component attaches 12.6 mm distal to the femoral attachment of the sMCL, and the shorter and thicker meniscotibial component attaches just distal to the edge of the articular cartilage of the medial tibial plateau [93]. In addition to the three primary medial structures (sMCL, POL, and dMCL), other major structures of the medial compartment include the adductor magnus tendon (AMT), medial patellofemoral ligament (MPFL), medial hamstring tendons, medial gastrocnemius tendon (MGT), and vastus medialis obliquus muscle.
Medial knee stability is provided by the sMCL, POL, and dMCL [85–88]. The sMCL and POL also contribute to anterior and posterior drawer loads in the intact knee [88]. The fixation differences between the proximal soft tissue attachment and the distal bony insertion of the sMCL provide biomechanical synergy [87, 96]. The proximal tibial division opposes valgus forces independently of flexion angle, whereas the more static distal division experiences the highest valgus load at 60° of flexion. Additionally, the sMCL provides resistance to external rotation, and to a lesser extent internal rotation, at increasing flexion angles [87, 88].
The POL functions reciprocally and complementarily to the sMCL, producing significantly higher load responses to internal torque at full extension. The POL also resists valgus forces, along with the meniscotibial attachments of the dMCL. The meniscotibial attachment resists valgus forces at 60° of flexion, and the meniscofemoral attachment resists valgus forces throughout flexion, though the dMCL mainly opposes external rotation between 30° and 90° [87].
In TKA with varus deformity, subperiosteal detachment of the medial soft tissue at the proximal tibia affects balancing relative to the function of the structures in the intact state. Flexion tightness requires anterior medial soft tissue release, whereas posterior release affects the extension gap [97]. Additionally, a sleeve of medial soft tissue is often tethered to osteophytes during removal, and greater bone removal may lead to increased gapping and early implant failure [98]. While soft tissue balancing is an important aspect of successful TKA, a preservation of the normal anatomy is important for implant longevity (Fig. 1.4).


Fig. 1.4
Medial view of a right cadaveric knee demonstrating isolated superficial medial collateral ligament (sMCL), medial patellofemoral ligament (MPFL), posterior oblique ligament (POL), semimembranosus tendon (Semimemb), and vastus medialis oblique (VMO)
1.6 Conclusions and Future Perspectives
Detailed anatomic knowledge is of outmost importance at the time of surgical procedures such as ligament reconstructions and joint arthroplasty. Oftentimes, ligament imbalances are present at the time of knee arthroplasties that may need to be addressed in conjunction with the bony work, and therefore a precise understanding of the anatomy and biomechanics is key. Furthermore, with the advent of cruciate-retaining prostheses, the awareness of the anatomy and the biomechanical consequences of the disruption of the structures can potentially yield better results. Further studies are needed to more thoroughly evaluate the long-term clinical effectiveness of various surgical techniques and prosthesis models, potentially with native ligamentous sparring methods to better preserve the anatomy and joint proprioception.
References
1.
2.
Grazio S, Balen D. Obesity: risk factor and predictor of osteoarthritis. Lijec Vjesn. 2009;131(1–2) 22–26. PMID: 19348352.
3.
4.
5.
D’Lima DD, Chen PC, Colwell Jr CW. Polyethylene contact stresses, articular congruity, and knee alignment. Clin Orthop Relat Res. 2001;392:232–8.CrossRef
6.
Berend ME, Ritter MA, Meding JB, Faris PM, Keating EM, Redelman R, et al. Tibial component failure mechanisms in total knee arthroplasty. Clin Orthop Relat Res. 2004;428:26–34.CrossRef
7.
Ensini A, Catani F, Leardini A, Romagnoli M, Giannini S. Alignments and clinical results in conventional and navigated total knee arthroplasty. Clin Orthop Relat Res. 2007;457:156–62.PubMed
8.
Jeffery RS, Morris RW, Denham RA. Coronal alignment after total knee replacement. J Bone Joint Surg Br. 1991;73(5):709–14.PubMed
9.
10.
11.
Giuliani JR. Anterior cruciate ligament anatomy a review of the anteromedial and posterolateral bundles. J Knee Surg. 2009;22(2):148–54.CrossRefPubMed
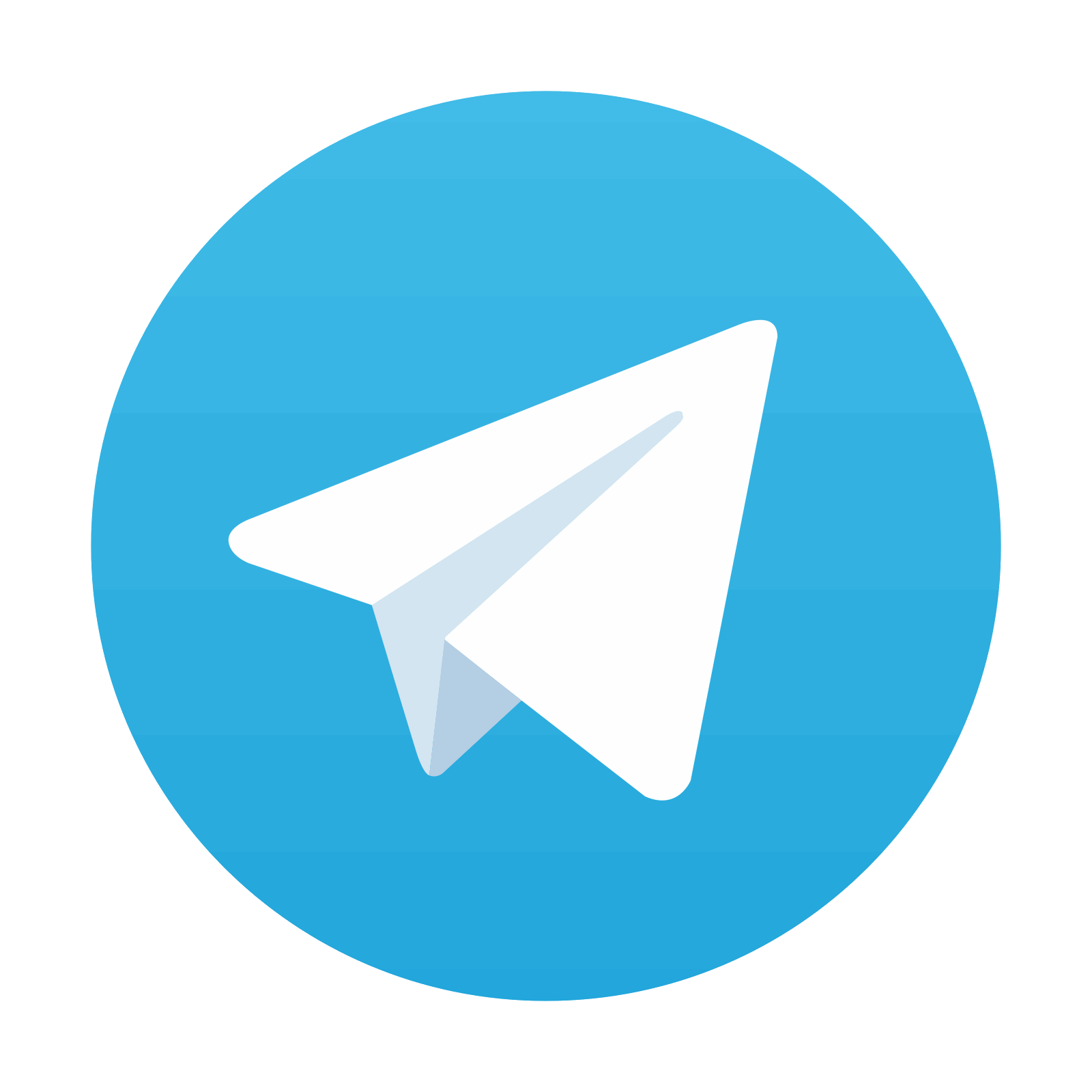
Stay updated, free articles. Join our Telegram channel

Full access? Get Clinical Tree
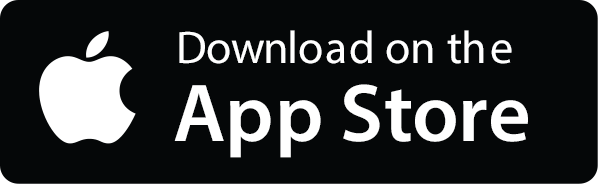
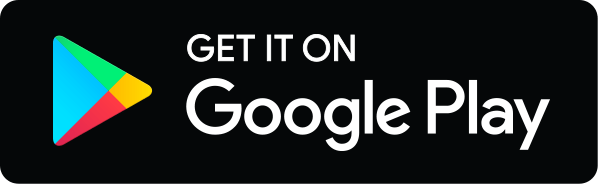