Fig. 18.1
(a) In some ways the extensor mechanism under load is like a drawn bow: When the energy in the bow is released, the ends spring apart and the bow string assumes the shortest distance between the two points at either end of the bow. The extensor straightens in the sagittal plane to extend the knee joint but it must still cope with angulation in the frontal plane due to the “Q” angle. (b) “The straight line” of the bow string propels the arrow forcefully. The patello-femoral joint converts quadriceps contraction to rotation or extension of the knee joint. The loads are significant and if the complex mechanism for guiding the extensor around the turning point at the knee fails, the patella will seek the shortest path and dislocate

Fig. 18.2
The running rigging on a sailing boat are under hundreds of kilograms of load. Sometimes they must be angulated such as at the bottom of the mast or along the deck as indicated by the path of 3 arrows. The pulleys that accomplish this function like the lateral facet of the patellofemoral joint
Knee extensor mechanics are usually analyzed as two separate systems: extensor power [1] and patellar tracking [2, 3] (Fig. 18.3a, b). Tracking, or the path of the patella in the frontal plane, including planar changes due to knee flexion, accommodates valgus knee alignment characterized by the “Q” angle (Fig. 18.3b). Reductive analyses like extensor power and “Q angle” are useful simplifications, but the real knee extensor tracks in three dimensions through a multitude of knee flexion angles, tethered to the tibia that rotates in two planes (flexion-extension and internal-external rotation) under the femur.


Fig. 18.3
(a) Usual biomechanical analysis of extensor function quantifies the joint reaction forces (J) based on the angle of knee flexion and the magnitude of force vectors representing the patellar tendon pull (P) and the quadriceps pull (Q). The direction and magnitude of J are solved with the parallelogram law of forces using the law of cosines: J2=P2+Q2-2PQ × COS angle between P and Q. This is only part of the story of patellar behaviour. (b) shows the frontal plane depiction of “Q” angle, defined as the angle subtended by lines from ASIS (anterior-superior iliac spine) to the centre of the patella, with the line of the patellar tendon. This analysis changes as the knee flexes and one plane (frontal) becomes two planes (of the tibia and the femur)
The phrase “patellar tracking” is misleading, as the behavior of this sesamoid bone cannot be understood in isolation from the entire limb. Tracking concerns the entire extensor, comprised of the origin of the quadriceps muscle, the muscle itself, the quadriceps tendon, the osseous patella, the patellar tendon, the tibial tubercle, limb alignment, and the dynamic translation and rotation of these parts in three dimensions. In an arthroplasty, by contrast with the native knee, the rotational position of implants and altered rotational dynamics of the tibia under the femur are additional variables a surgeon must consider (Fig. 18.4).


Fig. 18.4
(a) A straighter “Q” angle should enhance the tendency for the patella to track centrally, by eliminating the laterally directed vector of the extensor. This can theoretically be accomplished by moving the proximal (Q) or the distal vectors (PT) medially. The former is theoretically possible but difficult (see text). (b) Tibial component rotation around the “y” axis is more likely to increase the Q angle than an increase in valgus alignment. For example, if valgus is increased by a clinically undesirable 5 degrees, the talus would be displaced 5 cm laterally but the tubercle about one tenth of that distance. (Tangent 5°=3.38= displacement/45 cm. Internal rotation of the tibial component will have a more direct effect on displacement of the tubercle. (c) Assuming a tibial component size of 50 mm medial to lateral and anteroposterior of 35 mm, internal rotation of 22° would displace the tubercle about 14 mm, just across the center of the lateral femoral condyle, so that a patella with a centrally placed dome would tend to articulate outside the trochlear groove. Berger et al. allowed up to 18° degrees of internal rotation as consistent with good function
18.2 What Is Tracking?
Extensor tracking describes all contact of the patellar and femoral articular surfaces from full extension to maximum flexion, with or without prosthetic resurfacing. How the extensor tracks affects knee function, patient comfort, and component wear. The extensor mechanism can be conceived as a flexible linear structure under dynamic loads. Tracking describes its physical relationship with a rigid but articulated “track,” formed by the femoral trochlea in extension and then by the distal intercondylar geometry as knee flexion proceeds.
18.3 Orientation of the Extensor: A Dynamic Structure
The orientation of the extensor is determined by the origin of the quadriceps muscle and its direction of pull proximally, the patella interacting with the trochlear groove, and the location of the tibial tubercle. Tensile load on a pliant extensor mechanism that is angled in valgus at the knee moves the (unrestrained) patella laterally. Straightening the pull of the extensor on the patella will enhance tracking. In most knees, maneuvers that move the proximal or distal vectors medially will align the quadriceps origin, the patella, and the tibial tubercle (Fig. 18.4a–c). These force vectors could theoretically be changed by modifications to the attachment of the extensor to the skeleton, but the anatomically complicated quadriceps origins are difficult to modify. Preferential vastus medialis strengthening [4] and medial surgical advancement are more practical interventions [5]. The tibial tubercle is probably the most significant structure in this equation. While the tubercle can be repositioned surgically, it is more commonly malpositioned inadvertently by the rotational position of the tibial component.


Fig. 18.5
Complete dislocation of a resurfaced patella in 45° of flexion. This patella will only be relocated as the knee extends fully, if at all. (Markings are from research protocol to measure “tilt” and “translation”)
18.4 The Trochlear Track
In contrast to the supple and dynamic extensor, which controls the patella, the trochlear groove is structural and static. Tracking will improve if the groove can be located where the patellar is driven by knee function. Because the patella moves laterally when loaded, anything that positions the prosthetic trochlear groove laterally is beneficial. In a somewhat frivolous analogy, the patella is a willful teenager, determined to go where it wants. The trochlear groove must be established in that place to contain it. In another analogy, centralizing the patella in the trochlear groove is a process of conciliation: reducing lateral excursions of the patella and simultaneously positioning the femoral trochlea in a more lateral position.
18.5 Maltracking
Maltracking is the converse of a matched trajectory of the extensor with the structure of the trochlea [6–8]. Maltracking can be described as incremental subluxation in millimeters of translation or degrees of tilt [9]. Complete escape from the trochlear groove is a qualitative change—dislocation. Then, the medial-patellofemoral ligament will have ruptured, and the patella can only return to the front of the knee with extension of the joint (Fig. 18.5). As the patella dislocates laterally, part of the quadriceps muscle vector creates a valgus moment on the tibia-femoral joint, exacerbating tibia-femoral instability (Fig. 18.6). If the extensor migrates behind the axis of knee flexion, it can temporarily and aberrantly function as a knee flexor (Fig. 18.6).


Fig. 18.6
In this arthritic knee with valgus deformity, the Q angle has “straightened” itself and the patella rides over the lateral femoral condyle, not in the trochlear groove. With the patella dislocated, the Q angle is in varus, the patella articulates with the lateral side of the femur and the extensor has become a valgus deforming force
Clinical problems that result directly from patellar maltracking include knee instability [10–12], buckling, pain, patellar fractures, patellar component loosening (Fig. 18.7), patellar component breakage, and wear [8]. Indirect problems associated with the same mechanics include stiffness [13].


Fig. 18.7
Many problems result from mal-tracking. In this case of maltracking, the patellar prosthesis cannot escape from the highly conforming trochlear groove. As the extensor dislocates, bone and prosthesis part company: patellar component loosening is caused by maltracking
18.6 Tracking Is Difficult Because of Valgus Knee Alignment
Extensor tracking might conceivably be improved in an arthroplasty by eliminating frontal plane angulation, except that some degree of anatomic tibial-femoral valgus (even in conjunction with an overall mechanical varus [14, 15]) is desirable. While ideal alignment has reemerged as a topic for discussion [16, 17], a valgus tibial-femoral angle decreases the energy consumption of human gait by decreasing medial-lateral oscillations of the human center of gravity with each step [18]. Despite current debates, most surgeons believe that some degree of valgus alignment also decreases medial load and aseptic loosening [19].
18.7 What Are the Forces on the Patella?
The patella-femoral joint experiences high anterior to posterior compression when the quadriceps contracts to extend the knee, to maintain a given flexion angle, or to slow the rate of flexion. Patellofemoral forces increase with flexion and diminish almost to zero in full knee extension [1]. The patella, sitting between the anterior femur and the extensor mechanism, displaces the quadriceps and patellar tendons anteriorly by a distance equal to its thickness, even in full extension, as a means to increase the moment arm in terminal extension. The extensor is never completely straight in the sagittal or frontal planes in most knees.
The magnitude of the lateral vector on the patella increases directly with the load generated by the quadriceps. The more forcefully the quadriceps contracts, the greater the force tending to straighten the extensor in both planes. Muscle action drives both desired knee extension and undesired lateral patellar subluxation. Extension power will be lost if the patella escapes laterally, out of the groove. Tension in the medial patella-femoral ligament (MPFL) resists lateral patellar dislocation, and the lateral trochlear ridge exerts compression medially against the lateral patellar facet to oppose patellar subluxation and keep the patella in front of the knee (Fig. 18.8a). Given the obliquity of the native lateral facet joint, much of this constraining force is shear. Part of the vector will be compression that forces the patella anteriorly in opposition to patella-femoral compression (Fig. 18.8b). In the words of Kapandji: “…the force of the quadriceps, directed obliquely superiorly and slightly laterally, is turned into a strictly vertical force” [3]. We fail to appreciate this interplay when extensor function is separated into extensor power and patellar tracking for analysis. A comparison of patellofemoral imaging with the extensor loaded and unloaded illustrates the potent effect of muscle contraction on a extensor tracking [20].


Fig. 18.8
(a) Intra-operative photograph at time of patello-femoral arthroplasty. The anteroposterior axis has been marked with dots. The prominent lateral trochlear ridge is indicated by the arrow. This is an anatomic mechanism opposing lateral patellar dislocation. (b) MRI of knee. As the patella begins to translate laterally with flexion (vector a) the shape of the lateral trochlear ridge forces it anteriorly. (vector b) This tendency is opposed by the patello-femoral joint reaction force (vector c) which maintains the patella in the groove
18.8 Rotational Mechanisms that Centralize the Native Patella
When the knee is fully extended, the patella sits proximal to and above the femoral trochlear groove. Even under conditions where maltracking is imminent, the problem will not be apparent in this position. As the knee flexes, the patella descends toward the groove, which is wider and flatter proximally, much like the opening of a funnel viewed in cross section. With further flexion, the patella is “captured” and descends into a conforming articulation, first in the trochlear groove and then between the distal femoral condyles, from which it cannot easily escape. In conditions of patella-femoral dysplasia, the confining lateral trochlear ridge may not easily guide and then restrain the patella, which can dislocate. Only with the patella in the groove can the quadriceps forcefully extend the knee joint.
Particular rotational phenomena aid patellar tracking at the extremes of flexion and extension. First, the “screw-home” mechanism locks the joint in full extension, diminishing quadriceps activity during standing. This involves external rotation of the tibia relative to the femur in terminal extension, displacing the patella laterally above the trochlear groove and seemingly increasing the risk of maltracking. The popliteus muscle however “unscrews” the mechanism prior to initiating flexion, rotating the tibia internally before the patella descends into the trochlear groove.
Secondly, femoral “rollback” describes the track of the femoral condyle on the tibial plateau anterior to posterior as flexion progresses. Though originally described as a symmetric path on both tibial condyles [21], more rollback occurs on the lateral tibial plateau. The tibia and its tubercle effectively rotate internally under the femoral condyles in deep flexion as the lateral femoral condyle progresses posteriorly on the tibia [22]. This draws the patella medially into the groove. Neither the asymmetric rollback [23] nor the popliteus-driven [24] screw-home mechanism is reliably replicated by arthroplasty surgery, leaving the replaced knee at an increased risk of patellar maltracking.
18.9 Arthroplasty Component Positioning to Avoid Maltracking
18.9.1 Depicting Component Position
Physical space can be depicted on three axes: X (medial to lateral), Y (proximal to distal), and Z (anterior to posterior). The position of objects, including knee arthroplasty components, can be defined as deviations from a neutral position in terms of translation (millimeters) and rotation (degrees) on these three axes, accounting for the frequent reference to “6 degrees of freedom” [25]. For example, rotation of a component around the “z” axis is commonly described as “varus” or “valgus” depending on the direction of rotation. Rotation around the vertical or “y” axis is reported as “internal” or “external” rotation, and translation on the “x” axis is described as “medial” or “lateral” (Fig. 18.9). Each choice can be described as beneficial, detrimental, or noncontributory to patellar tracking (Table 18.1).



Fig. 18.9
Component position can be depicted on three axes: x (medial-lateral) y (proximal-distal) and z (anterior-posterior). Component position can be defined as deviations from the central or neutral position in terms of translation (measured as distance) and rotation (measured in degrees). This accounts for 6 degrees of freedom. The bi-directionality of each vector doubles those options. Accordingly, varus and valgus can be described as counter-clock wise and clockwise rotation respectively around the z axis. Anterior and posterior as translation on the z axis
Table 18.1
“Six-degrees of freedom” for positioning of objects in three dimensions. The far left column refers to the three axes: “X” horizontal or medial-lateral, “Y” inferior-superior or vertical and “Z” anterior-posterior, or at right angles to the plane formed by the intersection of “X” and “Y”. Physical motion can be described as “translation” indicating a new location without re-orientation and measured as length or distance and “rotation” indicating a reorientation and measured in “degrees”. The second and third columns describe three degrees of freedom; translation on each of the three axes. Because translation is bidirectional, there are a total of 6 translational directions possible. The third column has common clinical terms used to describe these motions.Columns 4 and 5 refer to rotation about each of the three axes. Rotation is possible in two directions on each axis. Column five includes the common clinical descriptions for each rotation.The numbers 1 through 6 in the third and fourth columns describe the degrees of freedom. The areas shaded in gray are of less importance to patellar tracking

Six degrees of freedom, each expressible in two directions and applied to both tibial and femoral components, give a multitude of permutations. The addition of a patellar component and soft tissue techniques create more choices. Only some options enhance patellar tracking, but the ones that do are critically important. Choices that straighten the pull of the extensor mechanism, i.e., less valgus, or that lateralize the structural patellar track enhance tracking. We can consider each of the degrees of freedom for all components as a comprehensive assessment of how to avoid maltracking.
18.9.2 Position of the Femoral Component
18.9.2.1 Mechanical Axis Implications
For most techniques of knee arthroplasty, the tibial component position is predetermined, either at right angles to the tibial axis (classic alignment) or to reproduce proximal tibial geometry (anatomic and kinematic alignments) [26]. Control of knee alignment is generally a function of the angular (rotational) position of the femoral component around the “z” axis. A decreased valgus mechanical axis of the knee straightens the extensor mechanism pull by reducing the Q angle. Decreased knee valgus also straightens the track. Assuming that the summed vectors of all parts of the quadriceps originate close to the center of the femoral head, a straight or “neutral mechanical axis” of the limb (collinear centers of femoral head, knee, and ankle) means that the mechanical alignment of the extensor mechanism (center of femoral head to articular center of the patella to the tibial tubercle) and the mechanical alignment of the extensor track (center of femoral head to center of trochlear groove to center of the knee) are both straight lines, at least in the fully extended knee. This would effectively resolve problems of patellar tracking.
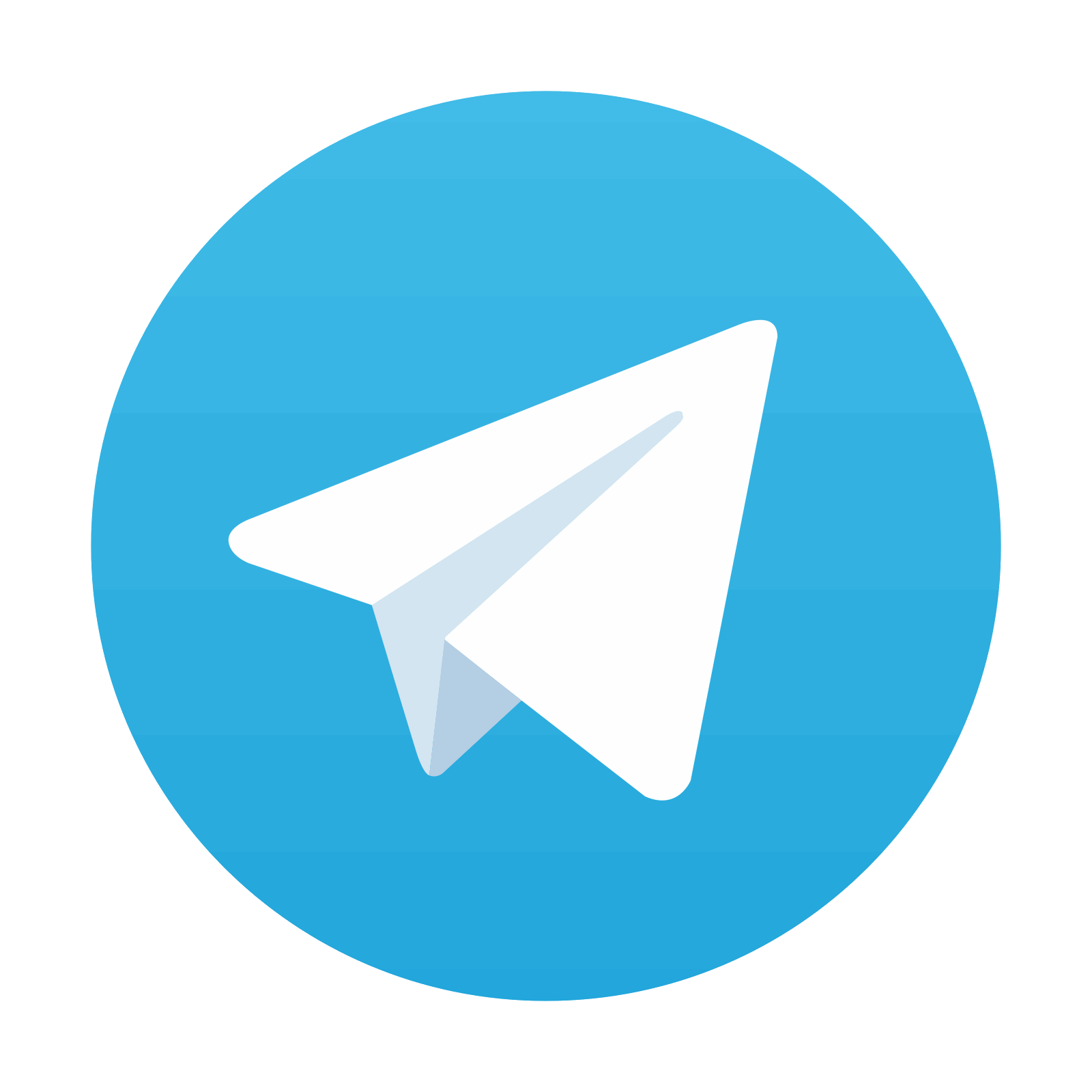
Stay updated, free articles. Join our Telegram channel

Full access? Get Clinical Tree
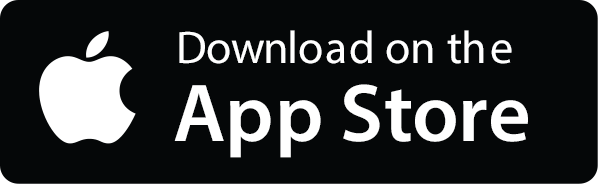
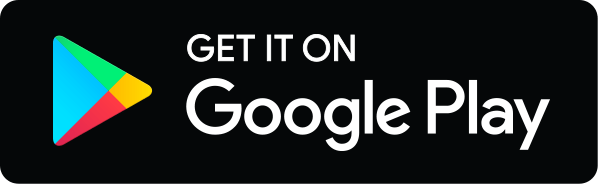