Superior and inferior surfaces of the clavicle. The origins of the muscles are shown with red color, and the insertions of the muscles and the tendons are shown in blue
As it can be seen, medial and lateral parts of the clavicle are strengthened by many factors on both surfaces, but the middle part is vulnerable due to lack of supporting factors. This is the reason why clavicle fractures most commonly occur in the middle part (80%) [12]. The clavicle has the highest rate of fractures among other bones in the skeletal system, statistically [15]. The diagnosis of the clavicle fractures can be done by examining the AC and CC ligaments [16].
The arterial supply of the clavicle is provided by three varied arteries. The first one is a branch of the thyrocervical trunk: the suprascapular artery. The second one is the thoracoacromial artery, which is the first artery that originates from the second part of the axillary artery. The last one is the internal thoracic artery, and it originates from the subclavian artery [17].
From embryological aspect, the clavicle has notable importance. It is the first bone to ossify in the early human embryo, between the 5th and the 7th weeks. However, the medial epiphysis of the clavicle is one of the last ossification centers in body to close at early 20s [18]. The clavicle is formed via intramembranous ossification, which is one of two mechanisms that the body uses for ossification process and does not need a cartilage model [19]. Besides, the clavicle does not have a medullary cavity. Still, it is classified as a long bone [12].
The clavicle articulates with acromion of the scapula at the lateral end and with the manubrium of the sternum at the medial end. At the medial end, also called the sternal end, the manubrium of the sternum and the clavicle are connected to each other via the SC joint. This joint is surrounded by a fibrous capsule and interacts with some anatomical structures such as costoclavicular and interclavicular ligaments. At the lateral end, the clavicle and the scapula are connected to each other via the AC joint, and they form a plane type of synovial joint. Together, they make up the shoulder girdle which is a complex of anatomical and physiological joints. Movements of the shoulder girdle are restricted mostly by the clavicle in all directions, specifically in forward direction [20].
1.1.3 Scapula
The scapula is a thin triangular bone which is one of the main components of the shoulder joint. Consisting of three borders (medial, lateral, and superior), three angles (inferior, superior, and lateral), and two surfaces (anterior and posterior), it is located posterolaterally behind the ribcage and extends between the 2nd and 7th ribs. Due to its thinness, the body of scapula is translucent [21, 22].
Embryologically, the scapula starts developing at the C4–C5 level and later descends into its position; its failure to descend results in Sprengel’s deformity [23]. It contains seven or more ossification centers with one in the body, two in the coracoid process, two in the acromion, one in the vertebral border, and one in the inferior angle. The scapula starts ossifying at several of these centers during the second month of fetal life. However, the ossification of the glenoid cavity, the coracoid process, the acromion, and the lateral border are not complete until late into puberty. Failure of the numerous ossification centers to fuse may result in anatomical variances such as the formation of os acromiale [24, 25].

The scapula creates a 30–45° angle with the frontal plane in transverse cross-section and 10–20° angle with the frontal plane in sagittal cross-section

The anterior and posterior views of the scapula

The drawings of the types of acromion (described by Bigliani et al.)

The drawing of the type IV acromion (described by Gagey et al. in 1993)
Inferomedial to the acromion, the lateral border of the scapula forms the glenoid cavity. Facing anterolaterally and slightly superiorly, the glenoid cavity is surrounded by the glenoid labrum. Together, these structures form a concave fossa which articulates with the head of humerus [21, 22].
The anterosuperior portion of the glenoid cavity is indented by a notch which provides it a specific shape. According to the prominence of this notch, glenoid cavity shape variations occur. In general, there are three types of glenoid cavities: oval, pear, and inverted comma. If the glenoid notch is absent, the cavity obtains an oval shape. If the notch is present but not distinct, the cavity is pear shaped. And if the notch is distinct, the cavity forms an inverted comma shape. Although the percentages vary depending on the research conducted, the pear-shaped glenoid cavity is the most common [32, 33].
In addition to the specific orientation of the scapula in the human body, the glenoid cavity has a specific orientation in regard to the scapula itself. According to a study carried out among 344 human scapulae, the glenoid cavity has, on average, 1.23° of retroversion (angled posteriorly) and 4.2° of superior inclination. Although no significant size difference was measured between races, the study showed significant racial difference in the measurement of glenoid version; white adults had an average of 2.66° of retroversion, while black adults had 0.20. The orientation of the scapula, especially the glenoid cavity, and the angular differences between races have extreme importance in glenoid surfacing for asymmetric glenoid bone loss; surgeons may consider these data while planning certain operations [34].
Additionally, there are important muscular attachment sites inferior and superior to the glenoid cavity. The infraglenoid tubercle provides attachment for the long head of triceps muscle. Similarly, the long head of biceps muscle attaches to the supraglenoid tubercle [21, 22].
Superior to the glenoid cavity is another process called the coracoid. Running superiorly, anteriorly, and then laterally, the coracoid process does not form any articulations but serves as an important attachment site for various muscles and ligaments. The short head of the biceps brachii, coracobrachialis, and pectoralis minor muscles attach to the coracoid process. Additionally, the coracoclavicular, acromioclavicular, and coracohumeral ligaments are attached to the coracoid process [21, 22].
At the base of the coracoid process, there is a small notch on the superior border of the scapula called the suprascapular notch (Fig. 1.9). Converted into a foramen by the transverse scapular ligament, the suprascapular notch forms a pathway for the suprascapular nerve. Although variations of suprascapular notch shape occur (u- and v shaped), there has been no correlation discovered between the variations in shape and suprascapular impingement/entrapment [21, 22, 35].
1.1.4 Humerus
The humerus, the bone which forms the skeleton of the arm itself, establishes the ball-and-socket-type shoulder joint and hinge-type elbow joint by articulating proximally with the glenoid cavity of the scapula and distally with the radius and ulna, respectively [36, 37]. It is the bone that helps us to position our upper extremity in space [38, 39].
Embryologically, the humerus is first visible as mesenchymal humerus at Carnegie stage 16, and the cartilaginous humerus begins to form during stages 16–17. The ossification of the humerus starts from the midshaft. The primary ossification center can be seen histologically by week 7 and the first bony collar appears at stage 21 (week 8) in the middle of the bone. By the 6th month, it extends proximally to the anatomical neck and distally to the olecranon fossa and the epicondyles. The secondary ossification centers are seen on the proximal and distal epiphyses. Both the proximal and the distal epiphyses also develop from separate ossification centers, and each of those ossification centers starts to be seen at different times in utero. These proximal and distal epiphyses become the future sides for the proximal and distal ends of the humerus. They complete their formation in the early childhood [40].
Based on our knowledge of embryology and anatomy, we can divide and study the humerus in three parts. These are the proximal end, the shaft, and the distal end of the humerus.
1.1.4.1 The Proximal Humerus

The picture showing the anterior and posterior surfaces of the proximal humerus
The head forms one-third of a sphere [40], and it projects medially, posteriorly, and superiorly [38, 40] to articulate with the glenoid cavity of the scapula. Covered by a hyaline cartilage, the head is the main part of the humerus that contributes to the formation of the shoulder joint. In a study made by Boileau and Walch, the diameter of the articular surface of the humeral head has been reported as 43.3 mm [41].
The anatomical neck is the obliquely directed, shallow, and constricted region between the head and the greater and lesser tubercles laterally and between the head and the shaft medially [38]. It represents the closed epiphyseal plate [37]. It provides attachment to the capsule of the glenohumeral joint, except in the superior part where there’s an area without capsular ligament for the passage of the long head of the biceps brachii muscle [36].
The greater and lesser tubercles are the two prominences found on the lateral side of the proximal humerus. The greater tubercle is located superiorly, and it provides attachments to the supraspinatus, the infraspinatus, and the teres minor muscles. The lesser tubercle is located more anteriorly and inferiorly, and it provides attachment to the subscapularis muscle. These four muscles form the “rotator cuff” muscles, which help to position the arm, and they are also the main providers of the stability and the integrity of the shoulder joint [38, 40]. In 1928, Meyer described the supratubercular ridge found on 17.5% of humeri and stated that it may help to prevent the medial displacement of the tendon of the long head of biceps brachii muscle by forcing it laterally [42]. Separating the greater and lesser tubercles, there is the intertubercular sulcus (bicipital groove) through which the long head of the biceps brachii muscle passes. The intertubercular sulcus continues distally to the shaft of humerus; the lateral lip, the medial lip, and the floor of it provide attachments to the pectoralis major, the teres major, and the latissimus dorsi muscles, respectively [36, 38, 40].
The surgical neck is the weak, horizontally oriented [38] region of the proximal humerus which is inferior to the greater and lesser tubercles and superior to the shaft of the humerus. The anterior circumflex humeral artery passes anteriorly, and the posterior circumflex humeral artery and the axillary nerve pass posteriorly to the surgical neck.
The proximal humerus is the third most commonly fractured bone in the body following distal radius and the proximal femur fractures. They are especially seen in elderly women as osteoporotic fractures following low-energy traumas [43]. They can also be seen in younger patients, but in those cases, it’s most commonly associated with high-energy traumas or sports injuries [44].
To understand the importance of the proximal humeral fractures, to decide the treatment options, and to predict the possible complications (such as avascular necrosis of the humeral head) and outcomes of the patients, scientists tried to define several types of classification systems. Those classifications were usually based on the levels of the fractures, the mechanisms of injuries, etc. In 1970, Charles Neer defined his own four-segment classification system based on the fractured segments of the proximal humerus and whether they are displaced or not [45]. He thought that the existing classifications were inadequate in evaluating the proximal humeral fractures. It has been more than 40 years, but surgeons still use Neer’s classification system widely because it is useful in understanding the pathological features of the fractures, deciding the possible outcomes and treatment options, and grouping for research purposes.
According to his classification system, Group 1 includes the fractures with displacements of less than 1.0 cm or angulations of less than 45°. This group constitutes more than 85% of all proximal humeral fractures, and they usually do not require surgery. Group 2 includes the fractures with pure displacement at the anatomical neck. Malunion or avascular necrosis can be seen in this type of fractures. Group 3 includes the fractures with displacement at the surgical neck. They can be either angulated, separated, or comminuted fractures. The fractures of the surgical neck are important because they may result in axillary nerve injury and they may also damage the arterial structures. These complications are especially seen in separated type of Group 3 fractures. Group 4 includes the greater tuberosity displacements and is pathognomonic for longitudinal rotator cuff tears. They can be either two-, three-, or four-part fractures. The prognosis gets worse when the displaced parts increase in number. Group 5 includes the lesser tuberosity displacements and can also be in the form of two-, three-, or four-part fractures. Finally, Group 6 includes the fractures with dislocations. In two- and three-part dislocations, the blood supply is usually maintained. In four-part fracture dislocations, the head is detached, and neurovascular symptoms are seen more commonly.
In 2004, Hertel et al. defined the binary description system by slightly modifying the Codman’s classification for proximal humeral fractures [46]. They stated that Neer’s classification system was unclear because there were several types of fracture planes that are not considered and there were some overlappings of the defined subgroups. Their aim was to assess the predictors of ischemia of the humeral head after fractures. They defined 12 fracture types based on 5 fracture planes. Those five fractures planes were between the head and the greater tuberosity, the greater tuberosity and the shaft, the greater tuberosity and the lesser tuberosity, the lesser tuberosity and the head, and the lesser tuberosity and the shaft. They also added criteria related to medial metaphyseal head extension and the integrity of the medial hinge. They stated that humeral head perfusion is important to decide the treatment options, and the most important predictors of this are the fracture types and the two additional criteria.

Anterior view of the vessels that supply blood to the proximal humerus
1.1.4.2 The Shaft of the Humerus
The shaft is the twisted portion of the humerus between the proximal and distal ends. It’s cylindrical in shape in the cross-sections of the upper half, whereas it’s triangular in the cross-sections of the lower half [40]. It has two important features: the deltoid tuberosity on the lateral side for the attachment of the deltoid muscle and the radial (spiral) groove that goes diagonally on the posterior side through which the radial nerve and the profunda brachii vessels pass [36, 52]. The fractures of the shaft in this area can damage these structures. Injury to the radial nerve may result in paralysis of the supinators of the forearm as well as the extensors of the wrist and the metacarpophalangeal joints. In this situation, the patient cannot extend the wrist and the fingers of the injured side, and with the unopposed force of the flexors, the wrist stays slightly in flexed position. This condition is called as “wrist drop,” or sometimes it’s called as “drop hand” [52].
The main nutrient foramen is the opening that is usually located on the anteromedial side of the midshaft, and it’s directed toward the distal end [53, 54]. It becomes apparent approximately at 9th to 10th weeks of embryological life [40]. The major blood supply to the humerus is via the nutrient artery that arises from the brachial artery [36], and it passes through this foramen into the medullary cavity of the humerus. There may also be some accessory foramina, but those foramina are usually located on the posterior aspect of the humeral shaft [54, 55]. The nutrient artery is particularly important during the active growth of the bone, the early phases of ossification [55], and during fracture healing [54]. The anatomy of the nutrient artery should be known and taken into consideration while performing the orthopaedical procedures like fracture reductions, bone microsurgery, bone grafting, etc.
At the lower part, the shaft widens, as the medial and lateral supracondylar ridges form on each side. The brachialis muscle attaches anteriorly, and the medial head of the triceps muscle attaches posteriorly to the distal part of the shaft [36, 52].
1.1.4.3 The Distal End of the Humerus
The distal end of the humerus is triangular in shape. It has two projections on either side called the medial and lateral epicondyles from which the flexor and extensor muscles of the forearm originate, respectively. The ulnar nerve passes posterior to the medial epicondyle and can be damaged in the fractures of the medial epicondyle. The articular surfaces of the distal humerus includes the capitulum laterally and trochlea medially to make articulations with the head of the radius and the proximal part of the ulna, respectively. Superior to them, there is the radial fossa laterally and coronoid fossa medially. The radial fossa accommodates the head of radius while the coronoid fossa accepts the coronoid process of the ulna during flexion of the elbow. On the posterior side, there is the olecranon fossa which accepts the olecranon process of the ulna during extension of the elbow [36, 40].
The blood supply of the distal humerus mainly relies on the descending branch of the main nutrient artery [47]. It gives branches to the supracondylar regions. However, the epicondylar regions are supplied mainly by the posterior ulnar recurrent artery, the recurrent interosseous artery, and the radial recurrent artery [56].
1.1.5 Sternoclavicular Joint
Sternoclavicular (SC) joint, or sternoclavicular articulation, is the connection between the manubrium of the sternum and the medial end of the clavicle. The SC joint is biaxial and classified as a saddle type of synovial joint, but despite its structural materials, it has the ability to act like a ball-and-socket type of joint functionally. Theoretically, the SC joint allows movement in three planes, as a triaxial joint. The reason it is not classified as triaxial joint is because rotation movements cannot be isolated from the body [9]. There is a fibrous joint capsule which surrounds the SC joint, and the capsule attaches to the articular disk. The articular disk lies between articular faces superiorly and inferiorly and separates the synovial cavity into two compartments [57]. The SC joint is the only true connection between the trunk and the upper limb structurally. Therefore, most shoulder movements originate from this articulation. Moreover, SC and acromioclavicular (AC) joints determine the position of the scapula; therefore, the position of the arm is strictly related to interwork of joints [9].
In the body, musculoskeletal movements are always restrained by limitative factors, specifically, by ligaments. The SC joint contains four major ligaments: anterior sternoclavicular ligament, posterior sternoclavicular ligament, interclavicular ligament, and costoclavicular ligament.

The sternoclavicular joint and associated structures, seen from anterior
As can be seen, the sternoclavicular joint performs movements under strong restraining factors. However, due to its synovial materials, it has a wide variety of motion abilities, such as protraction (30°), retraction (30°), elevation (45°), and depression (10°). In addition to these, when the arm is elevated via flexion, the clavicle rotates around its longitudinal axis [9, 57].
1.1.6 Acromioclavicular Joint
The acromioclavicular (AC) joint is a plane-type of synovial joint which connects the lateral end of the clavicle and the acromion of the scapula. It is surrounded by a thin, loose fibrous capsule, and the capsule is supported by AC ligament, superiorly. The lateral face of the clavicle and the medial face of the acromion are covered with fibrous cartilage, and between two articular faces, there is a meniscoid intraarticular disk [57]. Due to the structure of the joint, it gives rise to non-axial gliding motions [59].
The blood supply of the AC joint is provided by suprascapular and thoracoacromial arteries which are the branches of the thyrocervical trunk and the axillary artery, respectively [57].
The AC joint increases the range of motion of the scapula and implicitly of the arm. It allows extra rotation of the scapula on the scapulothoracic joint and contributes to raise the arm above the head [59].

The acromioclavicular joint, the glenohumeral joint, and associated structures seen from anterior
There are some conditions which can cause injury to the AC joint. Several of those conditions are AC joint dislocations, AC arthritis, and distal clavicle osteolysis [13, 16]. The AC joint dislocation, also called shoulder separation, is a condition when someone has a hard fall on his shoulder or has stretched his arm over limits [57]. The classification of AC joint dislocations can be done by checking the AC ligament and CC ligament. CC ligament ruptures indicate severe injuries and result with elevation of the clavicle [15].
1.1.7 Glenohumeral Joint
The glenohumeral joint, also called the shoulder joint, is the ball-and-socket type of synovial joint between the head of the humerus and the glenoid cavity of the scapula. The glenoid cavity of the scapula, in comparison to the humeral head, is so small and shallow that it only accepts little more than a third of it [60]. This articulation allows the glenohumeral joint to have greater range of motion than any other joint in the body [15, 61]. The glenohumeral joint can perform movements around the three axes so that the arm can perform flexion-extension, abduction-adduction, medial and lateral rotation, and circumduction [60].
Restraints of glenohumeral joint
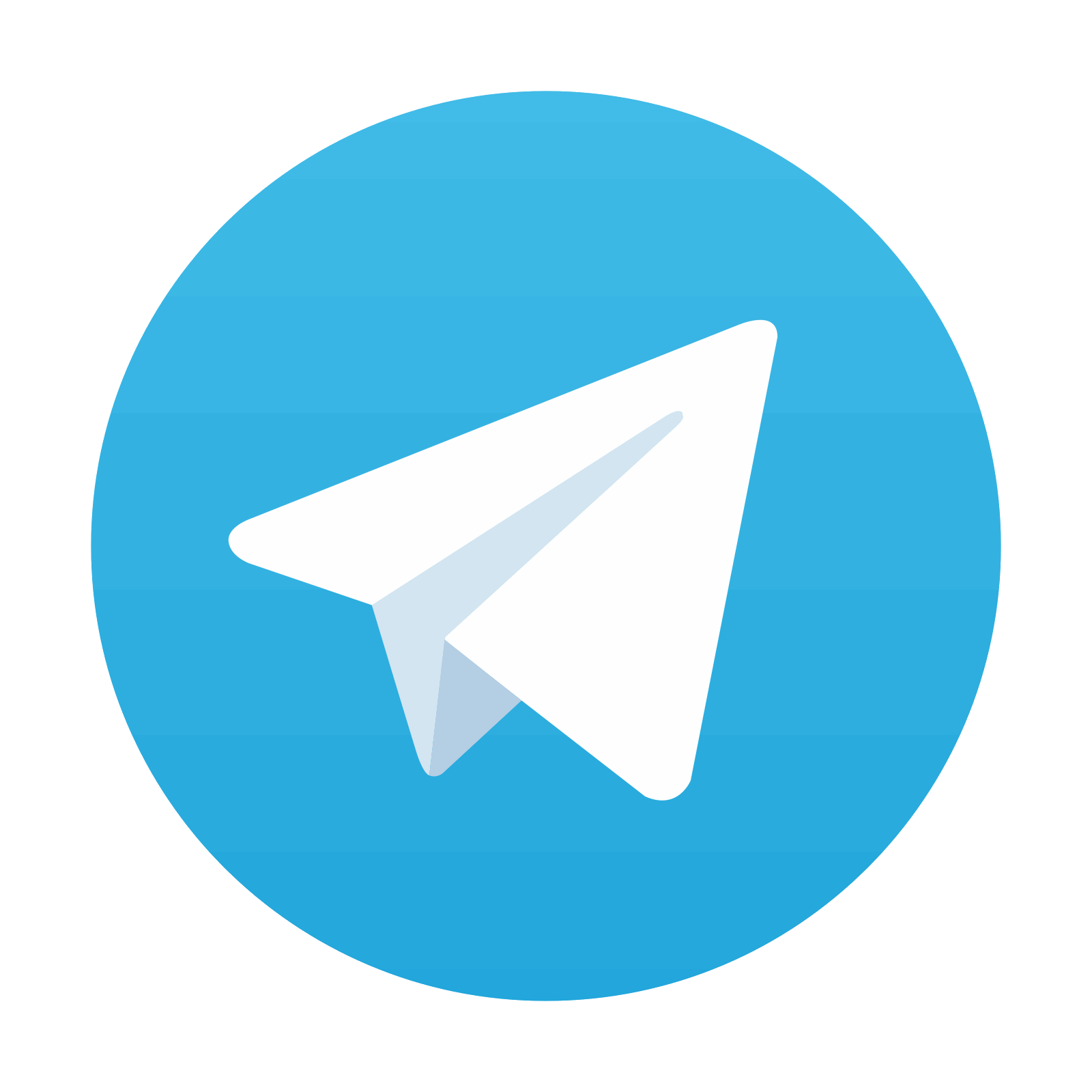
Stay updated, free articles. Join our Telegram channel

Full access? Get Clinical Tree
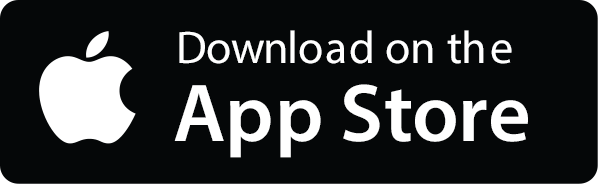
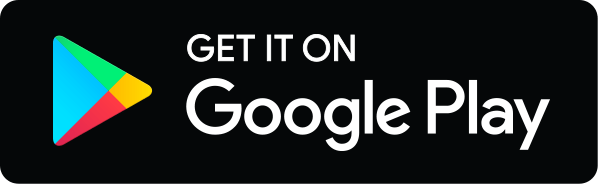