Adaptation in ROM Loss and Recovery
The capacity of the musculoskeletal system to undergo range of movement (ROM) adaptation can be seen in a number of human experiences, from the dramatic elongation of the anterior abdominal wall in pregnancy and the gains of flexibility in yoga and dance to ROM losses during immobilization and ROM recovery during rehabilitation. These adaptive processes are whole-person changes that can be observed in the tissue, neurological and psychological dimensions (Fig. 4.1).
FIGURE 4.1 Adaptation in range of movement (ROM) loss and recovery is multidimensional. Adaptation in the neurological and psychological dimensions is discussed in Chapters 8, 9 and 11.
This chapter will explore the following topics:
What is the physiology of adaptation?
What happens to tissues during ROM loss?
How do tissues adapt in ROM recovery?
The focus in this chapter will be primarily on adaptation in the tissue dimension, using immobilization and post-injury and post-surgery conditions as a model to explore ROM loss and recovery.
The Wonders of Mechanotransduction
Underlying the body’s adaptive capacity is a physiological mechanism called mechanotransduction. It is a mechanism whereby the body converts mechanical signals into biological processes.1–18 A notable example of mechanotransduction is the musculoskeletal adaptation seen in response to sports training. Mechanotransduction is also the process underlying the tissue changes observed during ROM loss and recovery.
Within the musculoskeletal system the masters of mechanotransduction are the myocytes and fibroblasts (often called mechanocytes). They produce the various biological materials that compose the tissues in which they are found.19,20 These physiological functions are modulated by the physical stress that is imposed on the tissues. Recurrent, habitual physical loading results in higher synthesis and turnover of these biological materials and lowered turnover during inactivity.21 Generally, mechanotransduction is more readily stimulated when connective tissue and muscle are preloaded in tension – the essence of many ROM challenges.22,23
More recently the interface at which mechanotransduction occurs has been identified as the attachment points between the fibroblasts’ and myocytes’ cell surfaces to the extra-cellular matrix.20,24,25 At these sites there are specialized proteins that act as mechanical sensors. When a stretch is applied, the deformation of the tissue activates membrane channels that convert the mechanical signals into chemical signals within the cells (Fig. 4.2).20,25
Mechanotransduction can be activated by mechanical signal alone in the absence of blood or nerve supply. This phenomenon can be demonstrated in denervated muscle and laboratory samples of muscle or isolated fibroblast/connective tissue.26,27 This highlights the significance of physical stress in shaping adaptation; a salient message for ROM rehabilitation.
The mechanical signals that stimulate mechanotransduction activate a cascade of cellular events that continue long after the cessation of stimulation. This phenomenon can be observed following resistance training.18 Muscle hypertrophy (biological dimension) does not occur during the training session (biomechanical dimension), but later, long after the cessation of exercise and including times when the person is resting. For example, a peak release of mechanogrowth factor can be observed in the human forearm muscles 2 hours after eccentric exercise.15 A similar phenomenon can be observed in connective tissue after the cessation of stretching or exercise. Fibroblast activity and reorientation of collagen fibres can be observed after several hours; it peaks at 24 hours and can remain elevated for up to 3 days.21,28
Dysfunctional Adaptation and ROM Losses
ROM loss is a form of dysfunctional adaptive reorganization that takes place when loading forces change or drop below a functional threshold (Fig. 4.3). The deleterious influences of underloading/immobilization are pervasive, affecting all musculoskeletal tissues, including the muscle–tendon unit, fascia, ligaments, joint capsule and intracapsular structures such as articular surfaces and synovium (Fig. 4.4).29–34
Connective tissue and intracapsular structures
There are several mechanisms associated with the loss of ROM during immobilization. The loss of extensibility is partly due to collagen fibres adhering to each other by chemical bonds called cross-linking.35 Under normal circumstances, the collagen fibres are intermingled with a gel, containing glycosaminoglycans, that has a high water content.36 This gel provides volume to the collagen matrix, helping to keep the collagen fibres apart, similar to dipping a cotton-bud in water – it tends to swell as the fibres separate. The gel also acts as a lubricant that allows the fibres to glide over each other. During immobilization the gel content tends to decline, resulting in approximation of the fibres and loss of lubrication.37 As a consequence, the fibres tend to “stick” to each other, and, like glue, will “set” and become progressively strong over several days.38 This process is more marked during the first 2 weeks after an injury or immobilization, when there is a high turnover of collagen in the tissues.
A similar process underlies adhesion formation. Here, the abnormal deposition of collagen and cross-linking is between gliding surfaces, such as tendons within their sheaths or synovial villi. In animal studies, adhesion of the synovial villi was found to be a major cause of ROM loss during immobilization (Fig. 4.4).39–43 Longer durations of immobilization result in stronger and more numerous cross-links. The consequence is formation of adhesions that have greater tensile strength than the tissues to which they are attached. When this occurs, vigorous stretching may fail to break up the adhesions but may damage the tissues to which they are attached.
In addition to cross-linking and adhesion tissue extensibility may be reduced by shortening and reorientation of the collagen fibres (Fig. 4.5).44,45 Normally, collagen fibres have a crimp-like structure organized along the lines of tension in the tissue. During tension loading, some elongation occurs by flattening of this crimp structure. In immobilization, this crimp architecture is lost. The normal longitudinal arrangement of the fibres becomes disorganized, forming a random criss-cross pattern. The outcome is stiffening and weakening of the tissue (Fig. 4.5).
Not all tissues respond equally to immobilization. Ligaments demonstrate reduced cross-section, weakening and an increase in compliance (less stiff), whereas tendons increase in stiffness.38,46–50 In animals, 8 weeks of immobilization resulted in a 40% decrease in tensile strength and a 55% increase in ligament compliance.51 However, this may vary between conditions; for example, in osteoarthritis of the knee there is an increase in stiffness of the medial, lateral and collateral ligaments.52,53
Clinically, it is difficult to assess tissue strength. There is always an uncertainty as to how much force we should use during stretching. This uncertainty can be overcome by introducing tissue loading in a graded manner over several sessions (see graded challenge, Ch. 6).
Muscle changes
Muscle–tendon adaptation is largely related to the efficiency and effectiveness of force production. Optimal force production is observed when the muscle length corresponds to the resting position of the muscle or neutral position of the joint. Force production is diminished if the muscle has to contract while overstretched or in a shortened position (plotted as the active force–angle curve; Fig. 4.6). This change in force (and velocity) generation is related to the overlap of the myofilaments. In the neutral position the cross-bridges are optimally overlapped for force generation, whereas they are excessively overlapped in the shortened position and insufficiently overlapped in the lengthened position.
During immobilization, the neutral position of the joint is displaced and, as a consequence, the muscle has to adapt around this new position.54 However, the sarcomeres have only a limited capacity to change their length. This is overcome by the muscle’s capacity to add or remove sarcomeres to preserve the optimal overlap (Fig. 4.7). This is akin to making a chain longer or shorter by adding or removing links rather than changing the size of the individual links. In a similar manner, sarcomeres are removed when the muscle is immobilized or forced to function in a shortened position, and added in the lengthened positions.54–64 The loss of sarcomeres in series can be as high as 40% in the shortened muscle, and there may be a 20% gain in the lengthened muscle.56 This adaptive process was shown to start within hours of immobilization or when the muscle is stimulated to contract in a shortened position/range.57 The deposition of new sarcomeres can be observed within 4 days of stimulating the muscle.65
During the first 2 weeks of immobilization muscle shortening/stiffening plays a larger role in loss of ROM than other joint structures. In animal studies, 2 weeks of joint immobilization resulted in a 20° loss as a result of muscle adaptation compared with a 6.5° loss attributed to joint structures. After that adjustment period, the muscle seems to complete the process of adaptation and “settle” around the angle of immobilization. At that stage it contributes marginally to the contracture. For example, at 32 weeks only a 1.0° loss is attributed to muscle tissue versus a 51.5° loss attributed to connective tissue structures.39–41
The reorganization of the muscle length around the position of immobilization is also associated with loss of parallel sarcomeres. The outcome is muscle atrophy and force loss, the essential components of the active ROM. This highlights the importance of introducing active challenges early in the ROM rehabilitation programme.
Connective tissue change in muscle
Normal mechanical links between the muscle cells and their final insertions are essential for optimal transmission of contraction forces.16,66 This link originates from the attachment of the myofilaments to the connective tissue in the extra-cellular matrix, to the perimysium and subsequently to the tendon, aponeuroses and bone. Transmission of the contraction force can be impeded by damage or dysfunctional adaptive changes in any part of this connective tissue link (Fig. 4.8).16,66,67
Changes in the muscle’s connective tissue can be observed during immobilization or partial mobility.16,66–68 Within the first 2 days after immobilization the ratio of collagen in muscle increases as a result of a rapid loss of muscle fibre (in the shortened but not in the lengthened muscle).66 Within 4 weeks of immobilization the muscle’s fascia can shorten by as much as 25%.59,60 Interestingly, in this particular study changes in the fascia were also observed in the non-immobilized, control side (15% decrease in the connective tissue length and reorientation of the fibres). This adaptation was associated with the abnormal movement patterns imposed on the animal by the one-sided immobilization. Furthermore, in immobilized muscle, collagen fibres were found to be arranged at a more acute angle to the axis of the muscle fibres than in controls.66
Adaptation in ROM Recovery
The adaptive recovery of ROM is the story of ROM loss but in reverse (Fig. 4.9).
Recovery in connective tissue
There are two potential mechanisms that could account for ROM recovery: normalization of the tissue’s stiffness/compliance or gradual adaptive elongation of the fibres.
During remobilization there are several factors which account for recovery the tissues’ extensibility: normalization of the gel content,37 reduction of abnormal collagen cross-bridges, recovery of the crimp structure, normalizing of fibre reorientation,28,35,69 and reduced intra-articular adhesions.70 In ligaments, reorganization of collagen fibres can take place in as little as 6 weeks of remobilization.
How length changes occur in connective tissue is not entirely clear. One potential mechanism is by assembly of collagen fibres in series, similar to the deposition and removal of sarcomeres in muscle. The fibroblasts lead a double life: they construct the tissue’s matrix but at the same time produce the enzymes that degrade it. This turnover of collagen is elevated during tensional loading and may account for a length adaptation. A similar length remodelling process is seen in connective tissue during the repair process.71–76
Remobilization has the added benefit of improving the tissue’s tensile strength by recovering its unique architecture and also by compacting collagen fibrils into thicker and denser bundles.34,35,77–82 In laboratory samples, stretched collagen was shown to be 10 times stronger, eight-fold denser, and eight times thinner than non-stretched samples.83
Generally, connective tissues tend to adapt over long time periods. After immobilization it can take connective tissues several months or longer to regain their mechanical properties.37,84
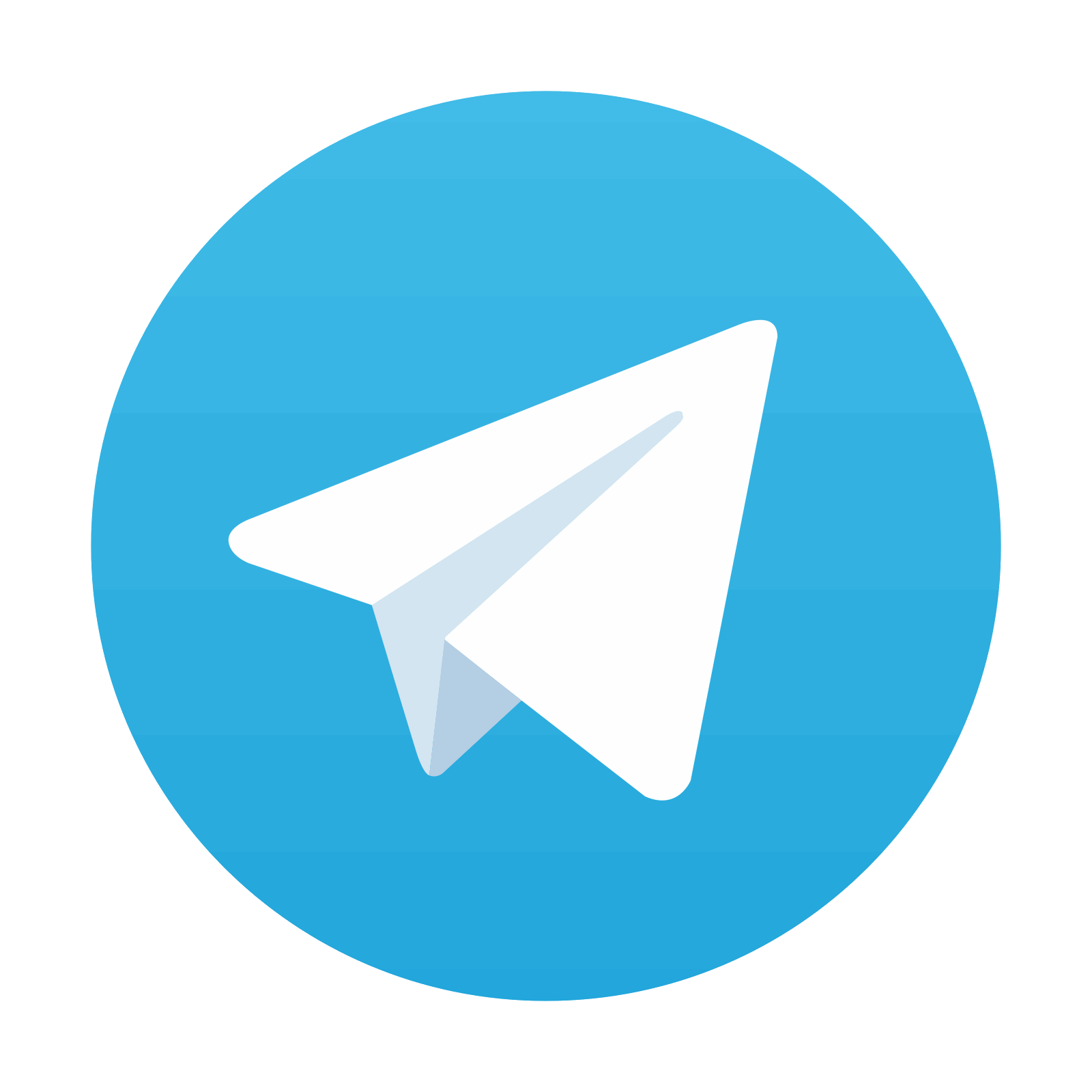
Stay updated, free articles. Join our Telegram channel

Full access? Get Clinical Tree
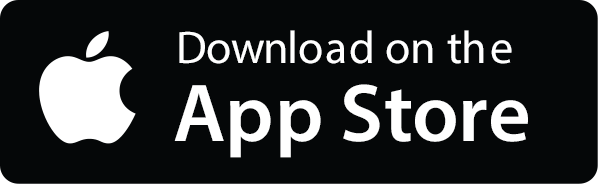
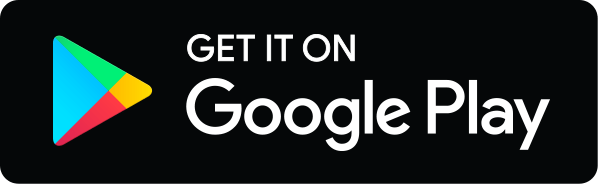