5 Physiological Boundary Conditions for Mechanical Testing
It is widely accepted that the mechanical environment within a bone plays a critical role for the progression and the final outcome of the regeneration and healing processes taking place after sustaining a fracture. However, not only the healing and adaptation processes of the biological tissues, but also the proper function of the implants and hardware used for the stabilization of a fracture depends critically upon the mechanical forces acting within the bone. Detailed knowledge of the loading conditions within the long bones is therefore an essential prerequisite for the successful development of new implants. Moreover, an understanding of how the interaction between the mechanical forces within the bone and the stability provided by an implant define the local mechanical environment at a fracture may allow the development and application of osteosynthesis devices in such a manner that the resulting mechanical environment will at least not hinder, but ideally even promote healing. It is essential that the mechanical loading conditions of a long bone are governed by the forces of the muscles and the contact forces transferred at the joints, 1, 2 and this chapter will therefore provide a review of our current understanding of the physiological loading conditions in the human lower extremity.
Key Concepts: Musculoskeletal Loading
The musculoskeletal system provides form, support, stability, and movement to the body. It consists of bones, muscles, cartilage, tendons, ligaments, and other connective tissue. The skeletal muscles are not only the essential active structures that enable us to move our body in a variety of ways and gaits, but they also provide the main contribution to the mechanical loading conditions within our limbs (Fig. 5.1). While muscle forces cannot be directly measured, the development of telemetric implants equipped with technology to measure the forces within the hip, knee, and shoulder joints has resulted in the most detailed and accurate knowledge of the forces acting across the large joints in the human body today. 3

5.1 In Vivo Loading Conditions at the Large Joints of the Lower Limbs
While previous numerical modeling approaches provided estimates of the forces at the hip as large as 10 times body weight during walking and stair climbing, the in vivo measurements of the joint forces could not confirm these predictions. According to the measurements, the forces at the large joints of the human lower extremities are rather in the range of about 2 to 3.5 times body weight during activities of daily living, with the largest forces at both the hip and knee joints determined during stair descent (Table 5.1).
Joint | Walking | Stair ascent | Stair descent | Rising from a chair |
Hip 4 | 2.38 | 2.51 | 2.60 | 1.90 |
Tibiofemoral3 | 2.61 | 3.16 | 3.46 | 2.46 |
Patellofemoral 5 | 0.76 | 2.80 | – | 3.10 |
Although the measurements indicate that there is only little variation in the force magnitudes across activities, and this observation generally holds true also for the three-dimensional orientation of the joint force vectors, there are small yet relevant differences in the quality of loading between walking and, for example, stair negotiation activities. As a result of the increased hip flexion, the muscles of the hip act more in the transverse plane of the femur and hence the anterior-posterior directed component of the hip force vector during stair negotiation can be up to twice as large as during level walking (Fig. 5.2). As a consequence, the torsional moments around the long axis of the femur are also increased during stair climbing (Fig. 5.3). The inclusion of stair climbing loads in the mechanical testing would therefore be particularly important for the development of devices that need to provide adequate stability not only during walking but throughout the spectrum of key activities of daily living. 6


5.2 Muscle Action Is a Key Modulator of the Internal Loading Conditions
The literature further suggests that specific situations such as stumbling are capable of causing excessively large internal forces. 7 Analysis of musculoskeletal interaction suggests that under these conditions, muscle activity alone is capable of generating extreme joint forces. Similarly, all other bony regions spanned by activated muscles may become excessively loaded during activities such as stumbling. If muscles are activated to their full potential, they may produce not only maximal forces at the joints and extreme compression forces in the bone, but also excessive bending and shear forces. Furthermore, experimental evidence suggests that attempts to unload the fracture site by controlling the external force might not be effective. 8 Taken together, these results suggest that in patients who received osteosynthetic treatment after bone fracture and exhibit unsecure gait patterns, it is likely that unusually large forces or relatively larger shear loading conditions exist, conditions that are particularly detrimental to the secure fixation of bone screws and can limit the longevity of the implants.
5.3 Loading Conditions within Long Bones
While telemetric implants provide excellent insight into the forces acting at the large joints, the mechanical loading conditions throughout the bones are modified by the action of the muscles and therefore differ from those observed directly at the joints. Although ethical considerations discourage the use of invasive methods for determining the coordinated action of all muscle forces in vivo by direct measurement in humans, computer models provide a powerful, alternative means to study the complex distribution of muscle forces in detail.
While early numerical analyses used to overestimate joint forces, recent modeling approaches demonstrate that analytical methods are able to predict the measured in vivo loads within an approximately 15% error in patients with telemetric hip or knee implants (Fig. 5.4). In order to obtain this high degree of accuracy, the key features of the individual musculoskeletal anatomy, accurate skeletal motion data, and information on the external loads are required.5

5.4 Organ Level Loading Conditions: Forces and Moments
As such validated models determine the muscle and joint forces throughout the entire lower limb, they are not limited to the study of the internal loading conditions at single locations such as the hip or knee, but rather provide access to the internal loading conditions throughout the entire bones. From such analyses, we understand that the internal loading of the femur is generally characterized by axial compression (Fz), with small shear forces directed mediolaterally (Fx) and anteroposteriorly (Fy) (Fig. 5.5). As a result of the activity of the abductor muscles during gait, compressive and shear forces are largest at the femoral head and decrease distally toward the diaphysis during walking. Under physiological loading, the bending moments in the femur are dominated by the frontal plane bending moment (My), whereas the torsional moment (Mz) is the smallest of all. Even though individual gait characteristics resulted in some variations of the patterns over a cycle, load magnitudes are comparable between patients during walking and stair climbing (Fig. 5.5 and Fig. 5.6).


Of note, however, are the considerable differences in the magnitude of the compressive forces acting down the femur (Fz). During stair climbing, forces peaked in the diaphysis because of contraction of the quadriceps muscles. The importance of considering the muscle contribution when analyzing long bone mechanical loading is further underlined by the fact that the bending moments determined were considerably smaller than those predicted by previous analyses, which neglected muscle forces.
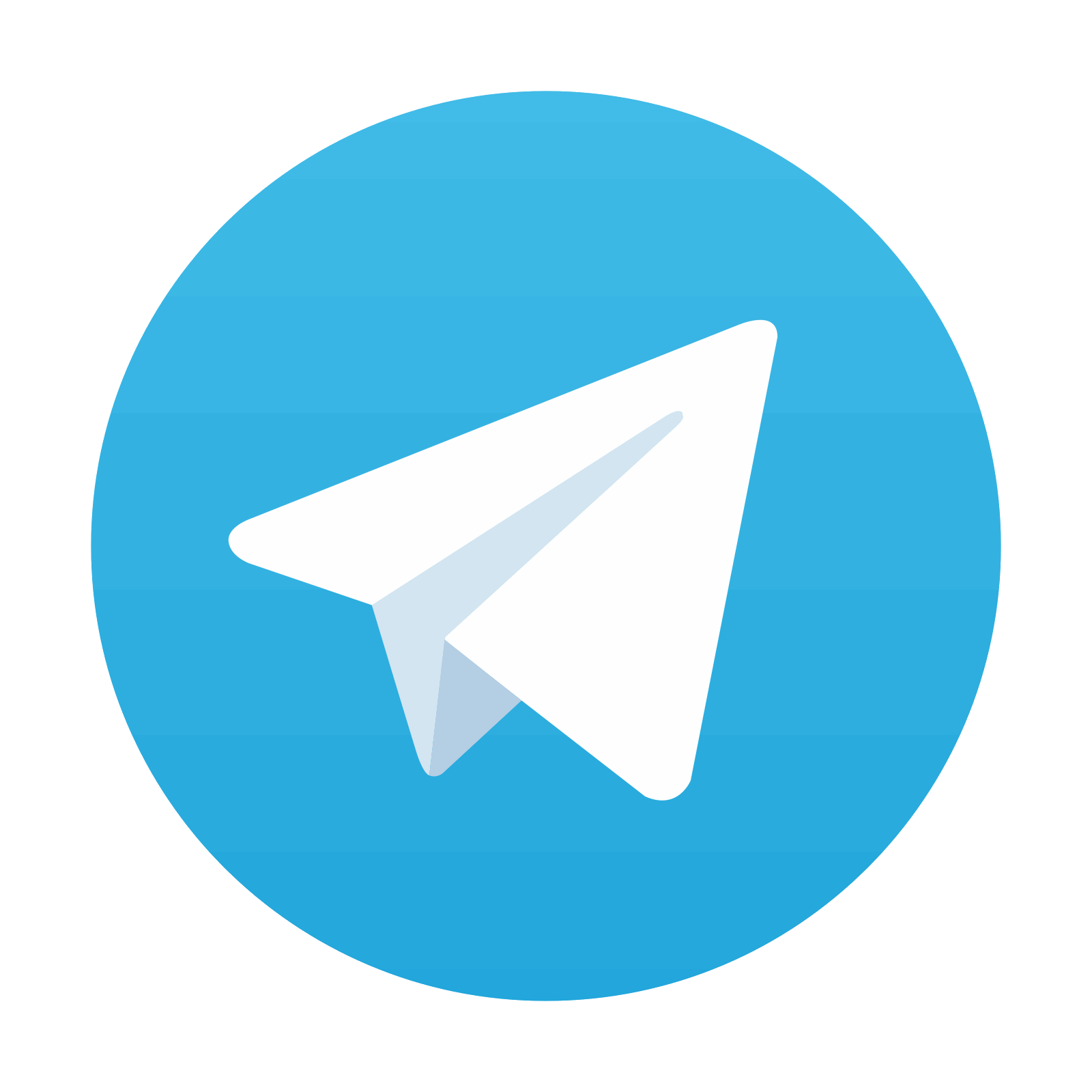
Stay updated, free articles. Join our Telegram channel

Full access? Get Clinical Tree
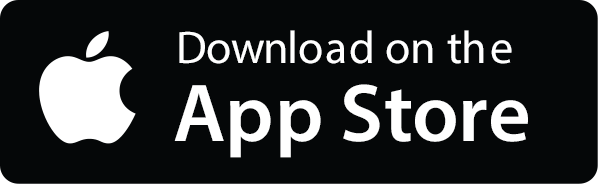
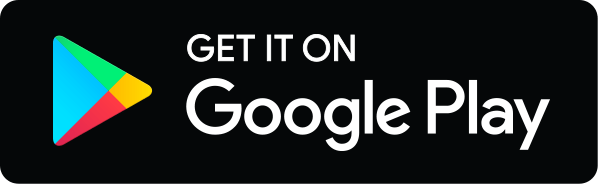