43 Models for Impaired Healing
Fracture healing occurs by one of two well-described ways. Direct fracture healing, which is believed to be uncommon in nature, involves the direct remodeling of lamellar bone, haversian canals, and blood vessels. Indirect bone healing occurs much more commonly in nature and combines endochondral and intramembranous bone healing in the overall process.
43.1 Indirect Bone Healing
This occurs when some instability remains between the fracture ends when healing begins. This process is seen when long bone fractures are treated nonoperatively, with intramedullary nailing or with external fixation, and also with plate fixation in the setting of a comminuted fracture. The initial phase of this process is the formation of a hematoma as blood seeps into the area from the surrounding soft tissues as a result of the concomitant injury and from the intramedullary canal. The clot that results from bleeding of the bone at the time of fracture ultimately acts as the template for subsequent callus formation. A highly regulated inflammatory phase generally follows within the next 24 hours. This inflammatory phase involves many known inflammatory cytokines and many still yet undiscovered compounds that will subsequently lead to tissue (in this case bone) regeneration. Proinflammatory factors include TNK-alpha, interleukin (IL)-1, -6, -11, and -18, with IL-1 and IL-6 of extreme importance in this process. These inflammatory factors serve to recruit additional cells into the area and promote angiogenesis. For further bone regeneration to occur mesenchymal stem cells must be recruited into the area. Mesenchymal stem cells are recruited from the surrounding soft tissues, bone marrow, and possibly from the systemic circulation in response to bone morphogenic proteins, stromal cell–derived factor 1, and other cytokines. Once in the area, they proliferate and differentiate into osteogenic cells. Cartilaginous callus begins to form between the fracture ends and external to the periosteum. In rats, rabbits, and mice, peak cartilage formation can be found 7 to 9 days following the fracture, providing increased stability to the fracture site. Simultaneously, intramembraneous ossification is occurring subperiosteally directly adjacent to the distal and proximal ends of the fracture. A molecular cascade that stimulates the production of collagen I and II and several signaling peptides follows. The transforming growth factor superfamily including transforming growth factor-B1, -B2, -B3, and GDF-5 are involved with chondrogenesis and endochondral ossification, while several bone morphogenetic proteins (BMPs; BMP-5 and -6) induce cell proliferation in intramembraneous ossification at periosteal sites. Revascularization of the callus occurs by the in growth of blood vessels from surrounding tissues and the new growth of blood vessels. These processes are regulated by angiopoietin and vascular endothelial growth factor, respectively. To permit revascularization of the callus, chondrocyte apoptosis and degradation of the cartilage also has to occur. Mineralization of the callus follows neovascularization and is regulated by a host of peptides including Wnt-family, macrophage–colony-stimulating factor including kappa-B ligand (RANKL), osteoprotegerin, and tumor necrosis factor-alpha. Mineralization of the soft callus and development of woven bone is then followed by a second resorptive phase with sequential removal of the hard callus and formation of lamellar bone structure with a central medullary cavity. This phase is orchestrated by cytokines including IL-1, TNK-alpha, and BMP2 as the resorption is performed by the osteoclasts and bone deposition is performed by the osteoblasts. This process may take quite some time to complete and requires the presence of a good blood supply.
43.2 Direct Fracture Healing
The process of direct fracture healing varies significantly from indirect fracture healing and occurs considerably less commonly in nature. Direct fracture healing occurs by direct remodeling of the lamellar bone, haversian canals, and local blood vessels. For direct fracture healing to occur, the fracture fragments must be brought into very close proximity and be held there rigidly during the healing process. That being said, two types of direct fracture healing are known to occur. One type of direct fracture healing is referred to as “contact healing,” which occurs when the fracture ends are no more than 0.02 mm apart and there is no more than 7% intrafragmentary strain. In this scenario, cutting cones formed by leading osteoclasts and trailing osteoblasts pass across the fracture area at a rate of 50 to 100 um/d. The osteoclasts are responsible for removing bone debris and necrotic bone, whereas the osteoblasts are responsible for laying down new bone across the fracture site. “Gap healing” occurs when a residual gap between the fracture fragments is no more than 0.8 to 1.0 mm; the gap is preliminary filled by woven bone that is oriented perpendicular to the long axis of the bone. Secondary osteonal reconstruction then takes place within this bone, which undergoes secondary remodeling resembling the contact bone healing that is occurring in other areas of the fracture as described previously.
43.3 Animal Models for Studying Fracture Healing
“Animal studies still represent an essential tool to analyze the biology of fracture healing.” 1 However, the differences in the anatomy and metabolism of animals compared to humans must be considered in the experimental setup and in the interpretation of the experimental results.1 Additionally, although there are considerable advantages (size, costs, availability, genetic modifications) to using small rodents (mice, rats) to study fracture healing, their primitive bone structure is not representative of our own. This being said, the majority of the of fracture healing studies use either a mouse tibia fracture model as originally described by Hiltunen et al or the rat femur fracture model, or a similar model modified for mice femurs. 2, 3 Because the mechanical environment of the healing fracture affects the cellular and molecular mechanisms of fracture healing, considerable thought must be given to the type of fracture model employed. Stabilization of the fractures can be performed with thin pins,3 intramedullary locking nails, 4 compression screws, external fixators, pin clip devices, and locking plates3,4, 5 (Table 43.1).
Animal models | |
Mouse tibial model | Hiltun A, 1993a |
Rat femur model | Bonnarens F, Einhorn TA, 19842 |
Mouse femur model | Manigrasso MB, 20043 |
Fixation models | |
Thin pins | Manigrasso MB, 20043 |
Intramedullary locking nails | Holstein JH, 2008a |
Compression screws | Mehta M, 2010a |
External fixators | Cheung KM, 2003a |
Pin clip devices | Garcia P, 2008a |
Locking plates | Matthys, 2008a |
Physiological compromise models | |
Diabetes | Kayal RA, 2007, 7 2009,a 2010a Albow J, 2009a |
Aging | Xing Z, 2010 |
Hypovitaminosis | Delgado-Martinez AD, 1998 14 Melhus G, 2007 15 Tanaka K, 2010 16 Holstein JH, 2010 17 Aleantara-Martos T, 2007a |
aNot referenced. |
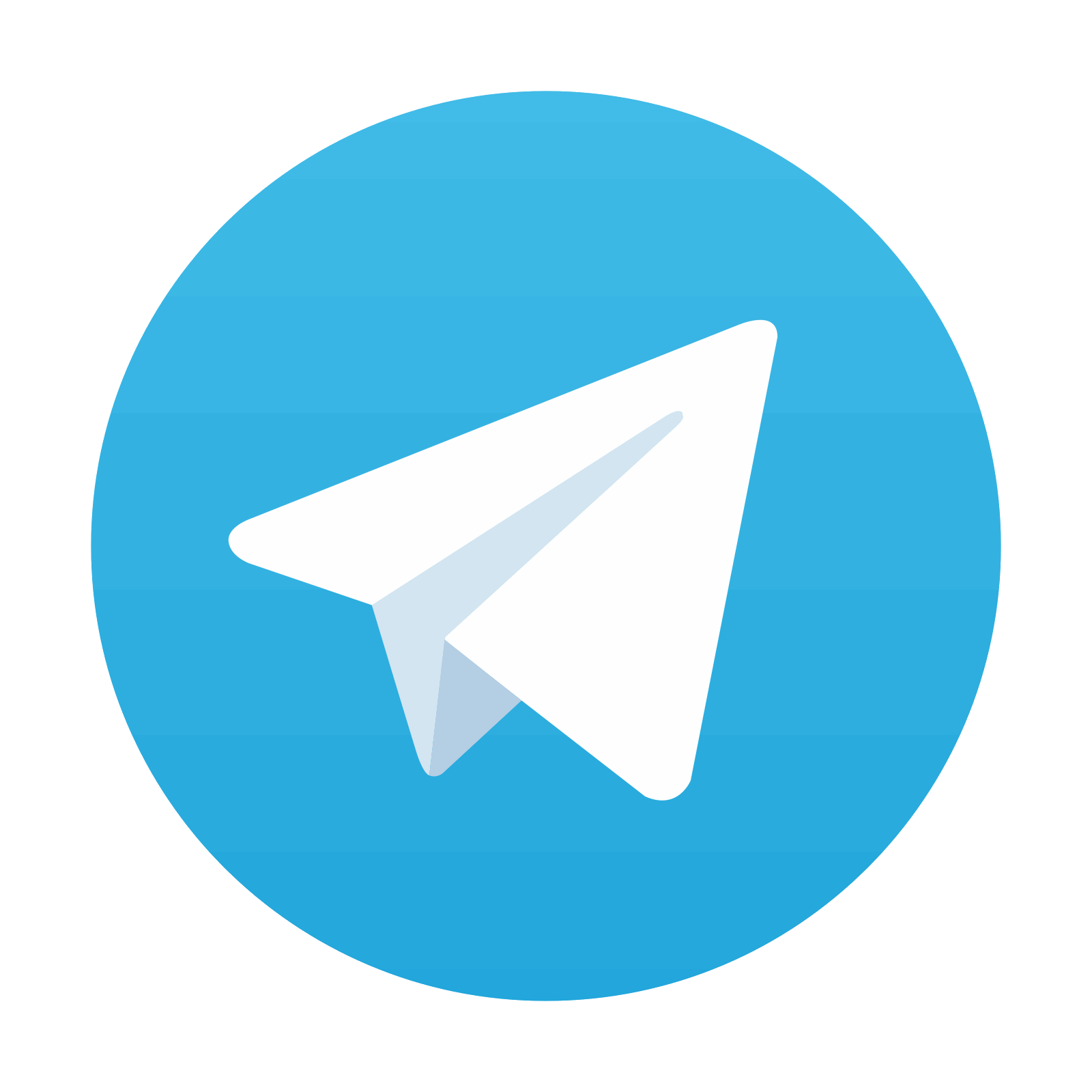
Stay updated, free articles. Join our Telegram channel

Full access? Get Clinical Tree
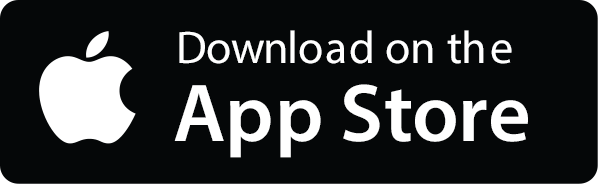
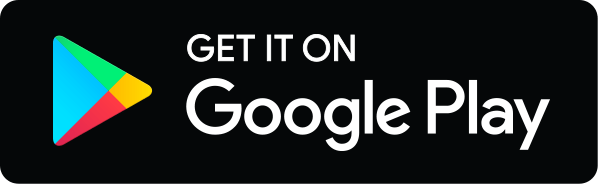