46 In Vivo Soft Tissue Models
This chapter describes the use of a plethora of different animal models for the investigation of meniscal, ligament, and tendon repair and regeneration. The purpose of this review is to inform the reader of the work that has been done, how it has been done, and where appropriate, how to improve on it. We believe that the advent of international standards, which define the requirements, specifications, and guidelines needed to optimally and consistently ensure the animal model systems investigated generate results that are repeatable, reliable, and of good quality, would be extremely advantageous and would minimize the numbers of animals used in this research. International standards would allow greater and more effective comparisons between studies, thereby maximizing good-quality scientific output through fewer animals. Animal models are needed for pretreatment evaluation prior to assessment in humans; however, where possible, investigators must always endeavor to reduce, refine, and replace animal use. A power analysis should always be carried out prior to commencing an investigation. Where possible, nonexperimental models should always be used, such as naturally occurring models and preclinical animals attending veterinary clinics. This chapter is also intended to assist investigators in fulfilling their obligation to plan and conduct animal experiments in accordance with the highest scientific, humane, and ethical principles.
This chapter is written with several goals: (1) to provide a foundation of generalized principles that will guide researchers in choosing optimal animal model systems and in the design of new model systems; (2) to review the current animal models available for advancing therapeutic options for meniscal, tendon, and ligament repair, regeneration, replacement, and attachment; and (3) to describe any shortcomings within these current models in order to highlight the need for further innovation and the development of more effective clinical therapies and patient care.
A single ideal animal model in which meniscal, tendon, or ligament tissue regeneration can or should be assessed is currently not available. In vivo models form part of a range of research methods that are used to test a specific biological hypothesis. The most effective model systems should meet several research criteria. 1 While animal models do not replicate the human scenario, they should provide an environment that matches, to the greatest extent possible, the appropriate physiological environment. 2 They should provide objective and quantifiable parameters to assess the success (quantity and quality) and functional performance of the repaired, reattached, or regenerated tissue. 3 They should detect and predict clinically relevant differences in biological performance between the parameters investigated.
46.1 The Meniscus
Many in vivo animal models have been developed to investigate the injury, repair, and replacement of knee menisci. These models are reported to have menisci that are structurally and anatomically similar to humans with comparable physiological responses (injury, healing, and remodeling). However, no one animal model has established itself as the most appropriate to be used when investigating all aspects of meniscal research, and many species are currently being used to successfully test specific hypotheses related to focused areas of meniscal investigation. In general, small animal models have been used to provide an assessment of the biological healing response to new materials implanted in the knee joint. Whether damage to the meniscus occurs at the time of trauma or sometime after is not clear, and therefore the mechanics of knee joint loading are imperative to understand treatment and recovery after injury. Therefore, the main disadvantage in the use of small animal models is that the implants are not subjected to the high loads that would occur during human knee motion. In addition, the accurate placement of defects and tears in the meniscus of small animals is very challenging due to its small size, and this aspect can play a great role in determining the repair outcome. Large animal models such as pigs, goats, and sheep offer the opportunity to investigate the meniscal healing response to new materials that are subject to immediate substantial loads. However, these models are often costly and time-consuming to conduct. The use of sheep models are reported to be advantageous because they are representative of humans in terms of structural properties, vascularization patterns, cell density, the presence of an articulating lamellar collagen structure, and in terms of cross-sectional size. These factors influence meniscal repair. Canine models offer additional advantageous postoperative management options. For example, after meniscal surgery, dogs can readily be managed with slings to keep them nonweight bearing or with bandages for support and to limit range of motion. In addition, controlled physical rehabilitation regimens using underwater treadmills, range of motion exercises, and other such activities can be utilized. Prior to beginning an experimental study, it is essential that a comprehensive review of the various animal models be performed in order to ensure the use of the most appropriate species relevant to your hypothesis.
The ability of meniscal tissue to heal is closely related to its blood supply, and meniscal tears are often classified according to the location of the defect relative to the blood supply. At birth, the whole meniscus is vascularized. However, an avascular area soon develops in the inner circumference of the meniscus, and in the second decade blood vessels are seen only in the outer third. This progressive loss of vascularization within the inner two-thirds of the meniscus may be due to weight bearing and knee movement. Tears that occur within the outer vascularized region of the menisci show good healing capabilities; however, tears within the inner avascular region have a diminished natural ability to heal. The vascular anatomy of the menisci can differ among animal species. Although the vascular supply of both the medial and lateral menisci originate in the peripheral capsular attachment in both humans and animals, the extent of vascular penetration into the meniscus can vary significantly. This is one of the most critical factors to recognize and account for when studying meniscal biology, response to injury, treatment, and healing. Investigators must be careful to confirm the location of the meniscal lesion they create and whether it is in the avascular or vascular portion.
46.1.1 Meniscal Tear Models
As discussed, tears in the avascular zone of the meniscus cannot heal entirely and spontaneously. Animal models that focus on attempts to induce healing in the avascular zone have had some success. In 1983, a canine meniscal model was the first to demonstrate that rasping of the damaged meniscus in the vascularized area promoted an injury response in the avascular region. A small transverse incision was introduced that produced a bridging vascular channel that redirected blood flow from the vascular zone into a longitudinal tear in the avascular zone. Rasping increases the production of interleukin-1-alpha, transforming growth factor-beta, platelet-derived growth factor, and proliferating cell nuclear antigen. This protein network has been demonstrated to improve vascular induction and meniscal healing. Further studies have also reported a significant increase in the healing of menisci within avascular zones when treated with a form of abrasion therapy when compared with control groups. The most common abrasion therapy techniques used are rasping or trephination, in which radially orientated channels are introduced to encourage vascular and cellular migration from the peripheral vascular portion to the tear site. A study by Klompmaker et al1 used a canine vascular channel access model to investigate the use of a porous polyurethane implant in meniscal healing by creating lesions within the avascular region. In this study, the right or left lateral meniscus was approached using a lateral incision to the knee joint capsule without detachment of any ligaments. A wedge-shaped defect was created with its base at the periphery and with the top of the defect reaching inwards to at least half of the width of the meniscal body. A longitudinal lesion was created well within the avascular midsubstance and which extended into both anterior and posterior meniscal horns. The porous polyurethane implant was sutured into the defect and partial and complete healing was reported in the experimental group where both type I and type II collagen, the two major collagen types of normal meniscal fibrocartilage, could be detected in this newly formed fibrocartilage. After the fibrocartilage had formed, vascularity was seen to decrease and was reported to be completely absent in the mature tissue. Within all empty, control lesions, no regeneration of fibrocartilage was seen. This study concluded that the implant guided vascular tissue from the periphery toward the lesion, resulting in healing of the tear. Over recent years, similar tear models have been developed in rabbits, dogs, sheep, and goats, and include procedures such as a synovial flap sewn into the tear, the application of a fibrin clot attached to the exposed collagen within the tear site, the use of small intestine mucosa, and trephination. Although success has been reported, each of these procedures has disadvantages when performed clinically. For example, a concern with the vascular channel access model is that it destroys healthy meniscal tissue resulting in poor meniscal function when bearing a load, even if the tear is repaired. The trephination models also have the same disadvantages, and it is technically very difficult to raise a pedicle of synovium and suture it into the meniscal tear. Although the application of fibrin glue has been shown to induce the proliferation of fibrous connective tissue that later develops into cartilaginous tissue 12 to 24 weeks postapplication, the disadvantage with this technique is the difficulty of keeping fibrin clots on the tear without immobilization of the operated leg.
More recently, studies have focused on the use of growth factors, gene therapy, and the application of mesenchymal stem cells (MSCs) on the repair and regeneration of meniscal tissue. The use of platelet-rich plasma in a rabbit meniscal model demonstrated increased histological fibrocartilage tissue scoring 12 weeks postsurgery in defects containing platelet-rich plasma when compared with control. Vascular endothelial growth factor (VEGF)-coated sutures have been investigated in the repair of sheep menisci. Following a 6-week follow-up period, results showed that none in the VEGF-coated suture group demonstrated healing, whereas defect healing was identified in half of those in the control group. These results were in agreement with another study where VEGF coated sutures (compared with Ethibond sutures; Ethicon, Somerville, NJ) were investigated at 8 weeks follow-up, and no significant increase in angiogenesis or meniscal healing was found in the VEGF-given groups. Transplanted autologous chondrocytes harvested from articular cartilage and placed within the avascular portions of a meniscal defect in a porcine model reported the ability of seeded chondrocytes to heal the tear. The avascular meniscal tear model has also been used in rabbits, and the effect of transplanted bone marrow cells within a fibrin glue carrier investigated. Earlier histological maturation and more rapid healing was seen in the cell given group when compared to defects containing fibrin glue only. Adaptations to this model have been also been used. In 2003, Murphy et al2 resected the medial meniscus and anterior cruciate ligament (ACL) in order to investigate the effect of bone marrow–derived MSCs injected intra-articularly on the regeneration of meniscal tissue. This study identified implanted cells within the regenerated meniscus and stated that the cells stimulated meniscal regeneration. These meniscal lesions have also been created in a porcine model and results showed that both autologous and allogeneic chondrocytes delivered in a biodegradable Vicryl mesh (Ethicon) enhanced healing of the avascular lesions.
46.1.2 Meniscal Injury and Osteoarthritis
Several animal models have been employed to demonstrate the direct relationship between meniscal injury and the development of osteoarthritis. While not described in detail in this chapter, these models include the rabbit ACL tear, menisectomy models, the canine Pond-Nuki model, canine meniscectomy or meniscal release models, and sheep and monkey models. Novel and more complex models are also being developed. For example, Killian et al3 have developed a model that attempts to reproduce acute, high-impact injury patterns within the meniscus and ACL following trauma in a rabbit model. This study showed that unconstrained high-intensity impacts on the tibiofemoral joint led to meniscal damage in conjunction with rupture of the ACL. Both acute and chronic changes to the menisci were identified, and the authors proposed this model to be more realistic in the progression of osteoarthritis compared with surgically transected models. Future work is needed to investigate the meniscal healing response in this model. Despite recent advances in the materials and methods used, a large proportion of meniscal tears in patients remain irreparable, and partial or total meniscectomy is often necessary regardless of the recognized consequences of cartilage degeneration and osteoarthrosis. In these cases, there is a need to protect the articular cartilage by replacing the meniscus.
46.1.3 Partial and Total Replacement Meniscal Models
Meniscal replacement presents a useful reconstructive option for patients with loss of the meniscus due to previous meniscectomy or an irreparable meniscus tear. The possibility to entirely reproduce the meniscus structure and function either through allograft transplantation or synthetic scaffold implantation is highly attractive. In the case of extensive destruction or a complete loss of the meniscus, partial and total meniscal replacement animal models have been developed to investigate restoring knee biomechanics in order to prevent the development of early osteoarthritis. Ideally, a meniscal replacement should mimic the shape and biomechanical properties of the meniscus. An ideal scaffold should have the basic structure of the meniscus and be biodegradable and bioabsorbable in the long term. The structure should also be strong enough to withstand the load in the joint and maintain its structural integrity without damaging articular cartilage. The basic biology of allograft meniscal transplantation has been studied in various animal models, and the results have been dependent on the conservation methods: fresh tissue, cryopreserved, fresh frozen, lyophilized, and glutaraldehyde-preserved allografts. While meniscal allografts have been shown to heal the capsule and relieve pain, their use is limited due to problems with availability, size matching, cost and risk of disease transmission, as well as graft remodeling and shrinkage after implantation. Incomplete cellular repopulation of deep frozen meniscal allografts after total meniscectomy and allograft transplantation in a rabbit model has been reported. Results showed the central core of the allograft remained acellular 12 and 26 weeks post-surgery; however, those cells that did repopulate the graft demonstrated active collagen remodeling. Synthetic scaffold materials have also been investigated as meniscal alternatives. Large wedge-shaped lesions have been created in the menisci of dogs. Lesions were repaired with a meniscal replacement manufactured from carbon fiber and an organic polymer, prepared from physical mixtures of poly(L-lactide) and a segmented polyurethane. Results at 4 and 8 weeks postoperation were unconvincing and showed the reconstructed area was invaded almost completely by fibrous tissue. A large meniscal defect created in the canine model has also been used to investigate the healing capacity of small intestine submucosa, and results demonstrated success in the repair of posterior vascular defects but not for complete meniscal substitution. A polyvinyl alcohol-hydrogel meniscus composed of porous 50/50 copoly(L-lactide/epsilon-caprolactone) was shown to be chondroprotective when investigated in a rabbit model. However, problems were reported with the durability of the polymer, the fixation method, and lack of tissue adherence. The polyvinyl alcohol hydrogel implant of Kobayashi et al 4 was one of the first nonporous permanent replacement approaches to be investigated. In this study, an implant with a compressive strength and viscoelastic properties similar to the human meniscus, was investigated following a 2-year in vivo period in a rabbit model. The implant proved to be chondroprotective; however, no sham-operated controls were included to evaluate the influence of replacement surgery on the cartilage condition. A 2-year canine model was used to investigate porous polyurethane scaffolds as total meniscal substitutes. Compressive properties of the implant increased at evaluation up to 2-years postimplantation, and results showed no difference when compared with the properties of native menisci. However, the material used was not strong enough to resist shear forces within the knee joint, and the collagen type and orientation were reported to be nonmeniscus-like.
Chiari and coworkers5 developed an ovine model of total and partial meniscus replacement. The sheep model has been used in many studies because the mechanical properties of the meniscus are similar to the human menisci, and the contact stresses transmitted to the tibial plateau are within the range of that of human knees. In the total meniscus replacement animal group, surgery involved cutting the medial collateral ligament (MCL) slightly above the joint line (Fig. 46.1). This was described as necessary in order to achieve good exposure of the posterior aspect of the joint. The meniscus was circumferentially dissected from the capsule; the posterior horn of the meniscus was exposed by flexion, external rotation, and valgus stress of the joint, and the meniscus was detached at its posterior and anterior horns. Two 3 mm tunnels were drilled using a guidewire from the medial tibial condyle to the footprints of the anterior and posterior meniscal horns. The implant was then fixed with two nonresorbable anchoring sutures inserted through the implant at its horns. The sutures were pulled through the bone tunnels and tied to each other in a knot on the tibial surface. Two silk threads were tied to the polylactic acid fibers protruding from the implant at its horns and pulled into the bone tunnels. The capsule was closed. Simultaneously, several additional sutures were made between the implant and the capsule. The anterior and posterior horns of the implant were fixed to the meniscal ligaments. The MCL was reconstructed with four sutures, using resorbable sutures. In the partial replacement group, the anterior portion of the meniscus was exposed by detaching the capsule at the periphery, while the MCL was left intact. The anterior portion of the meniscus was cut out radially at the level of the MCL. The size of the implant was adjusted using a scalpel so that it corresponded to the resected portion of the meniscus. The implant was then sutured to the remaining portion of the meniscus and to the remnants of the anterior horn fixation near the ACL. The capsule was closed. Simultaneously, several additional sutures were made between the implant and the capsule.

Surgeons are often confronted with loss of the inner portions of the meniscus in partial meniscus defects and only a peripheral rim remains. The ovine model developed by Chiari et al5 created a different type of defect (anterior partial resection with the MCL left untouched), and a limitation of this model is that the defect does not reflect the clinical situation. However, this type of partial resection was used to successfully study the integration of an implant to the residual meniscus throughout its radial diameter. Blunn et al also developed a similar ovine partial meniscectomy model to investigate the use of xenograft (Fig. 46.2).

Tissue engineering has recently been proposed as a possible solution for meniscal regeneration. This model has been used further to investigate the use of a porous scaffold based on a hyaluronic acid and polycaprolactone matrix augmented with circumferential lactic acid fibers. Cultured autologous chondrocytes were seeded onto the scaffold and results demonstrated improved fibrocartilaginous tissue deposition compared with cell-free constructs; however, the cell-seeded scaffolds did not show better cartilage conditions compared with control. Kang et al 6 demonstrated the feasibility of regenerating whole-meniscal cartilage in a rabbit total meniscectomy model using allogeneic meniscal cells seeded onto a mechanically stabilized polymer (polygylcolic-polylactic acid) scaffold. Neomenisci engineered without cell seeding showed significant failure of maintenance of the original scaffold shape and size. When total meniscal substitution was investigated in the ovine model at 4 months postoperation, stem cells seeded into a resorbable scaffold also showed promising results. A collagenous sponge loaded with MSCs and placed within a partial meniscus defect in rabbits also reported stem cells to augment the repair process.
In terms of microstructure, physiology, and material properties, there is no evidence to suggest that one animal model is best for the study of meniscal biology. No matter the animal model used, it is the responsibility of the investigators to carefully define the valid levels of comparison for each model and to interpret the results within these established confines.
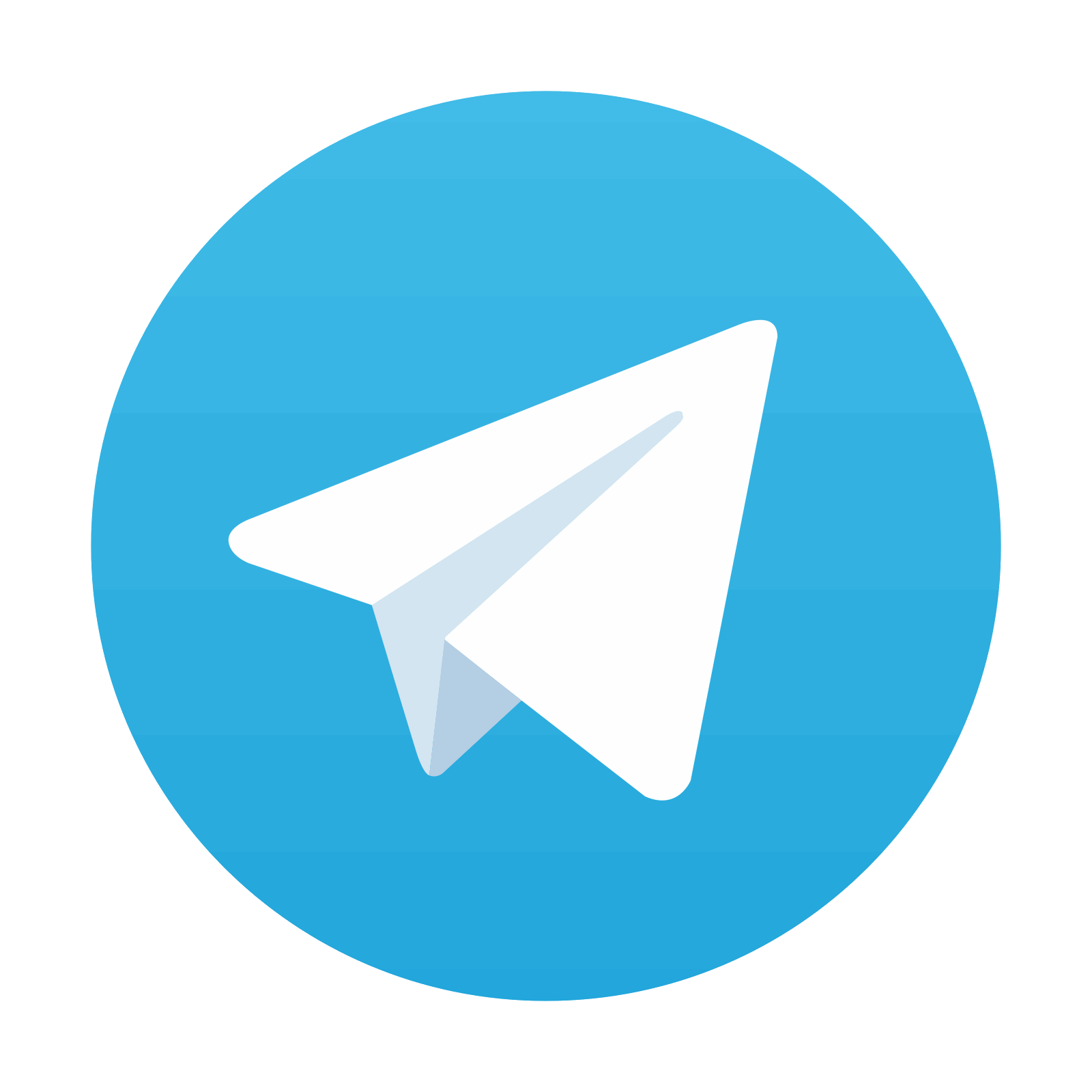
Stay updated, free articles. Join our Telegram channel

Full access? Get Clinical Tree
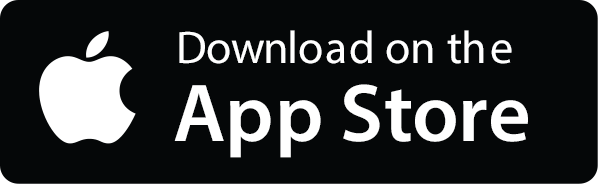
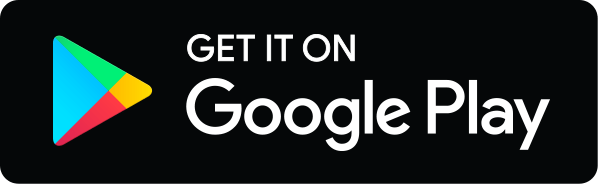
