3 The Genetics of Congenital Scoliosis and Abnormal Vertebral Segmentation
Before the modern molecular era, genetic analyses were often undertaken as epidemiologic studies, leading to the detailed assembly of empiric data that would allow analysis of possible inheritance patterns and recurrence risks for the purpose of genetic counseling. In the field of infantile idiopathic scoliosis, Wynne-Davies examined 134 infants and their first-degree relatives and found that approximately 3% of parents and 3% of siblings had the same, or a similar, deformity. 1 Congenital heart disease was present in 2.5% (the general population incidence is ~6 per 1,000 live births) and mental retardation in 13%, suggesting that a significant proportion of children had a syndromic form of congenital scoliosis (CS). Erol et al studied 81 patients with different forms of CS and segmentation defects of the vertebrae (SDV); of 39 patients prospectively recruited into study, 15 (38%) were found to have multiple-organ/syndromic associations, many of which fit into the oculo-auriculo-vertebral (OAV) (or Goldenhar) spectrum. 2 Purkiss et al studied 237 patients with CS and identified 49 patients who had two or more family members with either congenital or idiopathic scoliosis, suggesting a much higher recurrence rate of 20.7%. 3 There was also a history of idiopathic scoliosis in 17.3% of the families. Maisenbacher et al reported that 10% of patients with CS reported having first-degree relatives with idiopathic scoliosis. 4 These risk data are diverse, and there is a need for more studies with clearer phenotypic stratification.
Table 3.1 lists rare syndromes that may include CS and/or SDV, along with the genetic basis, if known. Most are very rare, and those most commonly encountered in clinical practice are OAV/Goldenhar spectrum, VATER or VACTERL (vertebral, anal, cardiac, tracheo-esophageal, renal, and limb) association, MURCS (müllerian duct, renal aplasia, cervicothoracic somite dysplasia) association, and maternal diabetes syndrome. The pathogenesis of these broad clinical groups is poorly understood. Any case series presenting to the spinal surgeon, pediatrician, or geneticist will demonstrate enormous radiologic and structural heterogeneity, and a syndromic or genetic diagnosis will be at best vague (including the OAV/VACTERL/MURCS associations because the cause[s] of these groups is unknown) and at worst completely elusive. Table 3.1 is a reminder that young (and not so young) patients presenting with CS/SDV should be examined and investigated very thoroughly for additional anomalies and a syndrome diagnosis considered. Referral to a clinical geneticist should therefore be part of the patient care pathway.
Syndrome/disorder | OMIM | Gene |
Acrofacial dysostosis | 263750 | DHODH |
Alagille syndrome | 118450 | JAG1, NOTCH2 |
Anhalt syndrome* | 601344 | |
Atelosteogenesis type III | 108721 | FLNB |
Campomelic dysplasia | 211970 | SOX9 |
Casamassima–Morton–Nance syndrome* | 271520 | |
Caudal regression* | 182940 | |
Cerebro-facio-thoracic dysplasia* | 213980 | TMC01 |
CHARGE syndrome | 214800 | CHD7 |
“Chromosomal” | ||
Currarino syndrome | 176450 | HLXB9 |
Atelosteogenesis type II (de la Chapelle syndrome) | 256050 | SLC26A2 |
DiGeorge syndrome / deletion 22q11.2 / velocardiofacial syndrome | 188400 | |
Dysspondylochondromatosis* | ||
Femoral hypoplasia–unusual facies* | 134780 | |
Fibrodysplasia ossificans progressiva | 135100 | ACVR1 |
Fryns–Moerman syndrome* | ||
Goldenhar / OAV spectrum* | 164210 | |
Holmes–Schimke* | ||
Incontinentia pigmenti | 308310 | IKBKG |
Kabuki syndrome* | 147920 | MLL2 |
McKusick–Kaufman syndrome | 236700 | MKKS |
KBG syndrome* | 148050 | ANKRD11 |
Klippel–Feil anomaly* | 148900 | GDF6, PAX1 † |
Larsen syndrome | 150250 | FLNB |
Lower mesodermal agenesis* | ||
Maternal diabetes mellitus* | ||
MURCS association* | 601076 | |
Multiple pterygium syndrome | 265000 | CHRNG |
OEIS syndrome* | 258040 | |
PHAVER syndrome* | 261575 | |
RAPADILINO syndrome (RECQL4-related disorders) | 266280 | RECQL4 |
Robinow syndrome (ROR2-related disorders) | 180700 | ROR2 |
Rolland–Desbuquois type* | 224400 | |
Rokitansky sequence* | 277000 | WNT4 † |
Silverman–Handmaker type of dyssegmental dysplasia (DDSH) | 224410 | HSPG2 |
Simpson–Golabi–Behmel syndrome | 312870 | GPC3 |
Sirenomelia* | 182940 | |
Spondylo-carpo-tarsal synostosis | 272460 | FLNB |
Thakker–Donnai syndrome* | 227255 | |
Toriello syndrome* | ||
Urioste syndrome* | ||
VATER / VACTERL association* | 192350 | |
Verloove–Vanhorick syndrome* | 215850 | |
Wildervanck syndrome* | 314600 | |
Zimmer syndrome* | 301090 | |
Abbreviations: CHARGE, coloboma, heart disease, atresia choanae, retarded growth, genital hypoplasia, ear anomalies; MURCS, müllerian duct, renal aplasia, cervicothoracic somite dysplasia; OAV, oculo-auriculo-vertebral; OEIS, omphalocele, exstrophy, imperforate anus, spinal defects; OMIM, Online Mendelian Inheritance in Man; PHAVER, pterygia, heart defects, autosomal recessive inheritance, vertebral defects, ear anomalies, radial defects; RAPADILINO, radial ray defect, patellae hypoplasia or aplasia and cleft or highly arched palate, diarrhea and dislocated joints, little size and limb malformations, long slender nose and normal intelligence; VACTERL, vertebral, anal, cardiac, tracheo-esophageal, renal, and limb. * Underlying cause not known. |
Although CS is frequently associated with SDV, this is not always so, and it may occur in severe forms of some syndromes in which segmentation anomalies are absent, although abnormalities of vertebral formation may be present. A presentation of this kind should prompt consideration of a diagnosis of one of the skeletal dysplasias, although a precise radiologic diagnosis may require follow-up skeletal surveys as the child grows and generalized bone growth evolves. A clinical genetics opinion with a view to genetic testing may be very helpful, and examples include the following: congenital contractural arachnodactyly (Beals syndrome), which is autosomal dominant and due to mutations in FBN2; chondrodysplasia punctata, Conradi–Hünermann type (Happle syndrome), which is X-linked and due to mutations in the EBP gene; diastrophic dysplasia, which is autosomal recessive and due to mutations in the sulfate transporter gene SLC26A2 (also known as DTDST); and spondylometaphyseal dysplasia, Kozlowski type, which is autosomal dominant and due to mutations in TRPV4.
3.1 The Spondylocostal Dysostoses and Somitogenesis
The main progress in understanding the genetic basis of SDV has come through the study of somitogenesis in animal models, particularly the mouse but also chick. Animals with specific gene knockouts are generated and multiple gene expression assays undertaken to help elucidate the developmental pathways. Somitogenesis is the sequential process whereby paired blocks of paraxial mesoderm are patterned from the presomitic mesoderm to form somites. It takes place between 20 and 32 days of human embryonic development, and in this process, pairs of somites are laid down on either side of the midline in a rostrocaudal direction. In mice, a pair of somites is formed every 1 to 3 hours, whereas in humans, the process is estimated to have a periodicity of 6 to 12 hours based on cell culture models and analysis of staged anatomical collections. 5 , 6 Somites ultimately give rise to four substructures: sclerotome, which forms the axial skeleton and ribs; dermotome, which forms the dermis; myotome, which forms the axial musculature; and syndetome, which forms the tendons. 7 , 8 Somitogenesis begins shortly after gastrulation and continues until the pre-programmed number of somite blocks is formed. In humans, 31 blocks of paired tissue are formed, but the number is specific for each species. The formation of somite boundaries is precisely timed and begins with the most rostral (head) somite, with the progressive laying down of more caudal somites. The establishment of boundaries takes place as a result of very finely tuned molecular processes determined by activation and negative feedback interactions between components of the Notch, Wnt and FGF signaling pathways. 9 , 10 In the rostral third of the presomitic mesoderm, the formation of segmental boundaries is subject to levels of the factor FGF8; this is produced in the caudal region of the embryo 11 and probably maintains cells in an immature state until levels fall below a threshold, allowing boundary formation. Somites already harbor specification toward their eventual vertebral identity, a process regulated by the Hox family of transcription factors, 12 which also display oscillatory expression in the mouse during somitogenesis. 13
The Wnt signaling pathway also displays oscillatory expression in a temporal phase different from that of Notch pathway genes, and it plays a key role in the segmentation clock. 14 , 15 , 16 The mediators of the determination front and the segmentation clock (Notch, FGF, Wnt) are required for forming the somite boundary and specify the rostrocaudal patterning of presumptive somites, for which Mesp2 is crucial. 17 Mesp2 is expressed caudal to the somite that is in the process of forming, and this domain is set where Notch signaling is active, FGF signaling is absent, and the transcription factor Tbx6 is expressed. Precise periodicity in the establishment of somite blocks is mediated by several so-called cycling, or oscillatory, genes, two of which, LFNG and HES7, have been implicated in humans as well as animals.
Somites themselves, having formed, are subsequently partitioned into rostral and caudal compartments, with vertebrae formed from the caudal compartment of one somite and the adjacent rostral compartment of the next, a phenomenon known as resegmentation. 18 , 19 , 20 , 21 An understanding of the molecular biology of somitogenesis in animal models, in combination with finding patients and families with specific forms, or patterns, of segmentation anomalies, has led to the most definitive progress in understanding the causes of CS/SDV, albeit in a rare group of disorders. Ongoing research is identifying more cycling genes and pathways involved in the regulation of somitogenesis.
3.2 Terminology
At this point, it is necessary to explain the use of terms, which in clinical practice is very inconsistent and confusing. The term spondylocostal dysostosis (SCD) has been, and continues to be, applied to a wide variety of radiologic phenotypes in which abnormal segmentation is evident, together with rib involvement. For the purposes of this review, however, the definition given in Table 3.2 is used. A number of attempts have been made to classify SDV. The scheme proposed by Mortier et al combines phenotype and inheritance pattern (Table 3.3). 22 The scheme proposed by Takikawa et al allows a very broad definition of SCD (Table 3.4), 23 but both these schemes identify Jarcho–Levin syndrome 24 with a crablike chest, which on more detailed scrutiny is misapplied. The surgical approach to classification of McMaster and Singh distinguishes between formation and segmentation errors (Table 3.5). 25 Like the scheme of McMaster and Singh, the classification scheme for vertebral abnormalities of Aburakawa et al, 23 , 26 which includes vertebral morphology (Table 3.6), does not attempt to identify phenotypic patterns of malformation based on assessment of the spine as a whole. Vertebral fusion or segmentation errors involving the cervical region, Klippel–Feil anomaly, has been subclassified (Table 3.7), 27 , 28 and Clarke et al (Table 3.8) proposed a further, detailed classification combining modes of inheritance. 29 The use of a limited number of terms in these classification schemes fails to reflect the great diversity of radiologic SDV phenotypes seen in clinical practice, and the schemes do not incorporate knowledge from molecular genetics. Furthermore, the diversity of SDV is not captured within the classification of osteochondrodysplasias. 30 , 31 A new scheme for classification and reporting from the International Consortium for Vertebral Anomalies and Scoliosis (ICVAS) is described later.
Features | Spondylocostal dysostosis | Spondylothoracic dysostosis |
General | No major asymmetry to chest shape Mild, nonprogressive scoliosis Multiple SDV (M-SDV; >10 contiguous segments) Absence of a bar Malaligned ribs with intercostal points of fusion | Chest shape symmetric, with ribs fanning out in a “crablike” appearance Mild, nonprogressive scoliosis or no scoliosis Generalized SDV (G-SDV) Regularly aligned ribs, fused posteriorly at the costovertebral origins, but no points of intercostal fusion |
Specific, descriptive | “Pebble beach” appearance of the vertebrae in early childhood radiographs (Fig. 3.1) | “Tramline” appearance of prominent vertebral pedicles in early childhood radiographs, not seen in SCD (Fig. 3.2) “Sickle cell” appearance of vertebrae on transverse imaging 40 |
Abbreviations: SCD, spondylocostal dysostosis; SDV, segmentation defects of the vertebrae. |


Nomenclature | Definition |
Jarcho–Levin syndrome | Autosomal recessive Symmetric crablike chest Lethal |
Spondylothoracic dysostosis | Autosomal recessive Intrafamilial variability, severe/lethal Associated anomalies uncommon |
Spondylocostal dysostosis | Autosomal dominant Benign |
Heterogeneous group | Sporadic Associated anomalies common |
Nomenclature | Definition |
Jarcho–Levin syndrome | Symmetric crablike chest |
Spondylocostal dysostosis | More than two vertebral anomalies associated with rib anomalies (fusion and/or absence) |
Type | Anatomical deformity | Anomalies |
I | Anterior failure of vertebral body formation | Posterolateral quadrant vertebrae
Posterior hemivertebrae
Butterfly (sagittal cleft) vertebrae Anterior or anterolateral wedged vertebrae
|
II | Anterior failure of vertebral body segmentation | Anterior unsegmented bar Anterolateral unsegmented bar |
III | Mixed | Anterolateral unsegmented bar Contralateral posterolateral quadrant vertebrae |
IV | Unclassifiable |
Failure of formation |
Type I |
A. Double pedicle |
B. Semisegmented |
C. Incarcerated |
Type II |
D. Not incarcerated, no lateral shift |
E. Not incarcerated, plus lateral shift |
Type III |
F. Multiple |
Type IV |
G. Wedge |
H. Butterfly |
Failure of segmentation |
I. Unilateral bar |
J. Complete block |
K. Wedge (plus narrow disk) |
Mixed |
L. Unilateral bar plus hemivertebra |
M. Unclassifiable |
Note: Hemivertebrae are seen in types B through F and in type L. |
Type | Site | Anomaly |
I | Cervical and upper thoracic vertebrae | Massive fusion with synostosis |
II | Cervical vertebrae | One or two interspaces only, hemivertebrae, occipito-atlantoid fusion |
III | Cervical and lower thoracic or lumbar vertebrae | Fusion |
Class | Vertebral fusions | Inheritance | Possible anomalies |
KF1 | Only class with C1 fusions C1 fusion not dominant Variable expression of other fusions | Recessive | Very short neck; heart, urogenital, craniofacial, hearing, limb, digital, ocular defects Variable expression |
KF2 | C2-C3 fusion dominant C2-C3 most rostral fusion Cervical, thoracic, and lumbar fusion variable within a family | Dominant | Craniofacial, hearing, otolaryngeal, skeletal, and limb defects Variable expression |
KF3 | Isolated cervical fusions Variable position Any cervical fusion except C1 | Recessive or reduced penetrance | Craniofacial, facial dysmorphology Variable expression |
KF4 | Fusion of cervical vertebrae, data limited | Possibly X-linked Predominantly females affected | Hearing and ocular anomalies: abducens palsy with retraction bulbi, also called Wildervanck syndrome |
According to the definition used here, SCD is a condition characterized by a short trunk and short stature due to multiple or generalized SDV (M-SDV or G-SDV), usually accompanied by rib fusions and/or malalignment. A mild, nonprogressive kyphoscoliosis is present, usually without additional organ abnormalities. Five Notch signaling pathway genes are now linked to this group, four demonstrating autosomal recessive inheritance and one autosomal dominant inheritance. These are now described in more detail, and Table 3.9 summarizes the conditions and their genes.
Gene symbol | Chromosomal locus | Protein name |
DLL3 | 19q13.1 | Delta-like protein 3 |
MESP2 | 15q26.1 | Mesoderm posterior protein 2 |
LFNG | 7p22 | Beta-1,3-N-acetylglucosaminyltransferase lunatic fringe |
HES7 | 17p13.2 | Transcription factor HES-7 |
TBX6 | 16p11.2 | DNA-binding protein T-BOX-6 |
In clinical practice, patients with abnormal vertebral segmentation, usually with only regional or very limited involvement of the spine, are much more commonly seen than patients with the rare monogenic forms, which perhaps affect 1 in 1,000 newborns. They are likely to occur sporadically, demonstrate more asymmetry, and be associated with other organ malformations.
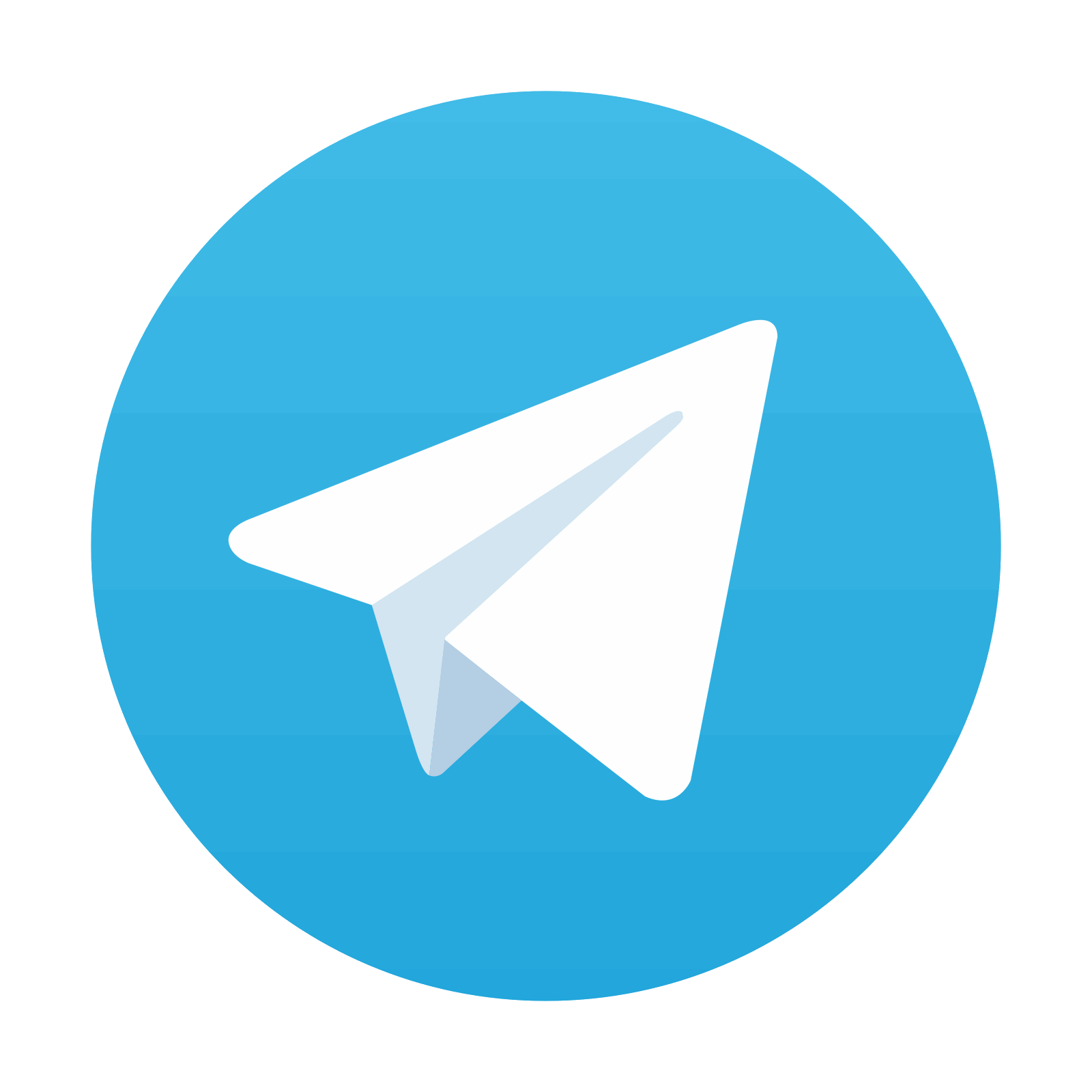
Stay updated, free articles. Join our Telegram channel

Full access? Get Clinical Tree
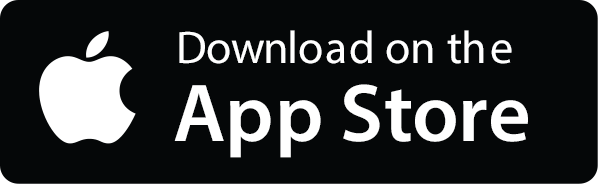
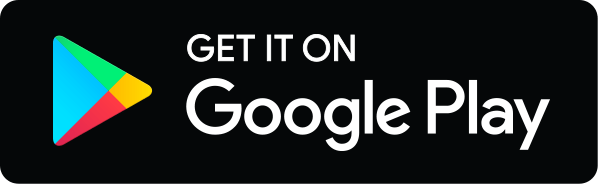