1 Frontier of the Impossible
When you have excluded the impossible, whatever remains, however improbable, must be the truth. —Sir Arthur Conan Doyle
Early onset scoliosis is one of the most challenging conditions in pediatric orthopedics and an important health issue. It is a condition with the potential to cause severe adverse consequences. The pathologic changes induced on a growing organism by an early onset spinal deformity can be dramatic and can, in the most severe cases, lead to death. A vertebral column that is not permitted to grow normally will affect the growth potential of the whole upper body, resulting in a short trunk, a disproportionate body habitus, and an underdeveloped thoracic cage (Fig. 1.1).

Pediatric orthopedic surgeons, by using the option of surgically managing young and very young children with early onset spinal deformity, have opened up a new perspective for spinal surgery. They have in effect broken down barriers that were previously felt to be insurmountable. Ingenuity has meant that management strategies have changed dramatically, shifting from a defensive attitude toward an offensive one. However, this audacious surgery is not without risk.
In young children with progressive deformity, there is a decrease of longitudinal growth and a loss of the normal proportionality of trunk growth. Abnormal growth leads to a deficit that sustains the deformity. As the spinal deformity progresses, by a “domino effect,” not only spinal growth but also the size and shape of the thoracic cage are modified. This distortion of the thoracic cage ultimately impairs lung development and cardiac function. Over time, the spine disorder changes in nature from a mainly orthopedic issue to a severe, systematic pediatric disease associated with thoracic insufficiency syndrome, cor pulmonale, and, in the most severe cases, death (Fig. 1.2).

Early spine fusion is not the answer for dealing with progressive, early onset spinal deformities. Arthrodesis in the thoracic spine at an early age does not address the impact of the deformity on the shape of the thoracic cage shape and development of the lung parenchyma, and it does not address the preservation of cardiopulmonary function. Moreover, early spinal fusion, especially in the thoracic region, is a cause of respiratory insufficiency and adds a loss of pulmonary function to the preexisting spinal deformity (Fig. 1.3).

The ideal treatment of early onset scoliosis has not yet been identified; both clinicians and surgeons still face multiple challenges, including preserving the thoracic spine, thoracic cage, lung growth, and cardiac function without reducing spinal motion. However, patients with early onset scoliosis are a heterogeneous population, so that it is difficult to compare the outcomes of different management strategies in meaningful numbers of patients; in addition, there is a lack of outcome assessment tools for this complex group of patients.
Management strategies must consider the complete life span of the patient, and the effects of treatment on distorted spinal and chest growth must be considered. Before any treatment is started, a convincing answer to these three questions must be given:
What is the functional benefit of the treatment?
What is the potential morbidity of the treatment?
What quality of life can be anticipated?
1.1 The Rib–Vertebral–Sternal Complex
In the concept of the rib–vertebral–sternal complex, a normal interaction occurs among the organic components of the spine, thoracic cage, and cardiorespiratory system. 1 , 2 , 3 This complex encloses the three-dimensional thoracic cavity and tends to be an elastic structural model similar to a cube in shape. However, in the presence of scoliosis, the complex becomes flat, rigid, and elliptical and prevents the lungs from expanding. These deformities can be lethal in the most severe cases as a result of reciprocal interactions and influences among the various skeletal and organic components of the thoracic cage and cavity that are not well understood. The development of the thoracic cage and lungs is a complex process that requires perfect synergy among the various components of the rib–vertebral–sternal complex. Alterations in any of these elements affect and change the development and growth of the others. It must be remembered that the thoracic cage volume represents about 6% of its definitive volume at birth, about 30% by the age of 5 years, and about 50% by the age of 10 years (Fig. 1.4). Moreover, between the age of 10 years and skeletal maturity, the thoracic cage volume doubles, and its volumetric growth ends on an upward trend.

To preserve thoracic motility and permit normal development of the respiratory tree, treatment should not focus only on the spine but should also consider the rib–vertebral–sternal complex as a whole.
1.2 Growth Holds the Basics
Sitting height correlates strictly with trunk height and is the best indicator for monitoring thoracic cage and spinal growth. In children with severe spinal deformities, the loss of sitting height is related to the severity of the deformity. For this reason, it is important to monitor changes in sitting height rather than in standing height in children with progressive spinal deformities. Standing height does not always exactly correlate with the loss of trunk height in children with severe spinal deformities because it includes subischial height.
It is important to follow the stages of growth. Three periods can be identified: (1) between birth and 5 years of age, characterized by a significant spinal growth; (2) between 5 years of age and the beginning of puberty, characterized by a reduction in spinal growth (also known as the quiescent phase); (3) the pubertal growth spurt, characterized by a new increase of spinal growth (Table 1.1).
Spinal segment | Age (years) | ||||||
1 | 3 | 5 | 7 | 9 | 11 | Pubertal spurt | |
T1-S1 | 2 | 1 | 1.8 | ||||
T1-T12 | 1.3 | 0.7 | 1.1 | ||||
L1-L5 | 0.7 | 0.3 | 0.7 | ||||
Note: Values are expressed in centimeters and are average values. A perivertebral arthrodesis in the T1-S1 segment at 5 years of age results in a sitting height of 15 cm (10 cm in the thoracic spine and 5 cm in the lumbar spine). |
Trunk growth is crucial between birth and 5 years of age because sitting height increases by 28 cm, whereas between 5 years of age and skeletal maturity the remaining trunk growth is 30 cm (Fig. 1.5). Moreover, the T1-S1 segment increases by 10 cm between birth and 5 years of age and almost doubles in length between 5 years of age and skeletal maturity (Table 1.2). It is therefore necessary to act fast, before pulmonary and cardiac alterations induced by a distorted spinal growth become irreversible. It is important to adapt management to growth rates. After the age of 5 years, the growth of the trunk decreases. Height increases by 2.5 cm and weight by 2.5 kg per year. This quiescent phase has to be used to stabilize the clinical situation because at the time of the pubertal growth spurt, the resumption of growth is going to worsen the spinal deformity. It is necessary to get ready for it and possibly to anticipate the need for a definitive surgery.

Developmental stage | Spinal segment | |||
Males | Females | |||
T1-T12 | L1-L5 | T1-T12 | L1-L5 | |
Newborn | 11 | 7.5 | 11 | 7.5 |
Child | 18 | 10.5 | 18 | 10.5 |
Pre-Adolescent | 22 | 12.5 | 22 | 12.5 |
Adult | 28 | 16 | 26 | 15.5 |
Note: Values are expressed in centimeters and are average values. |
All growths are interrelated. Any abnormal growth, by a “domino effect,” leads to another abnormal growth. The irregular growth of vertebral bodies is the basis of a distorted development. Severe, progressive early onset spinal deformities lead to abnormal spine growth that alters thoracic and lung growth, which finally affects the cardiopulmonary system. 1 , 4
The goal of any treatment is to break this vicious cycle; it is necessary to correct all distortions secondary to distorted spinal growth as soon as possible: short height and disproportionate body habitus, underdeveloped thoracic cage and inability to breathe normally, low weight and cardiac dysfunction. Tachypnea, ventricular tachycardia, dyspnea, tracheomalacia, weight loss, and chronic obstructive pulmonary diseases are often more worrisome elements than the distortion of the vertebral column itself.
1.3 Alveolarization: When Is It Complete?
Pediatric respiratory physiology is not well understood. It is difficult to investigate children younger than 5 years of age. Every day, a child exchanges 12,000 L of air. At birth, the lung parenchyma volume is 400 cc3; it is approximately 900 and 1,500 cc3 at ages 5 and 10 years, respectively, and about 4,500 cc3 for boys and 3,500 cc3 for girls at skeletal maturity. 1 , 2
Lung growth is a complex phenomenon in which different pulmonary structures and regions grow at different rates. At birth, the newborn has the same number of conducting airways as an adult. Tracheal caliber increases two- to threefold between birth and skeletal maturity. In contrast, the peripheral regions of the lung containing alveoli and pulmonary capillaries, also known as the acinar regions, undergo substantial postnatal growth and development.
Alveolarization is the process in which lung alveoli develop by multiplication. Once alveolar cell multiplication ceases, lung growth continues by alveolar cell expansion. It is estimated that about 30 to 50% of alveoli are present at birth; from the late fetal stage to 4 years of age, the number of alveoli grows by a factor of 10.
However, there is no agreement on when alveolarization stops. Some studies suggest that alveolar multiplication is completed by age 3 years, whereas others point out that alveolarization stops at age 8 years. Postmortem studies have shown that patients with early-onset deformities have fewer alveoli than expected, and that emphysematous changes in existing alveoli are present. These studies suggest that mechanical compression is not a factor in reducing the number of alveoli, and that this reduction is probably due to a premature cessation of alveolar proliferation. These data are currently being questioned as new findings are discovered with innovative study techniques such as electric impedance tomography, helium magnetic resonance imaging, and high resolution computed tomography.
The average number of alveoli reaches about 90 million at age 3 years and about 300 million during adulthood. These findings suggest that alveolar multiplication continues beyond early childhood. Moreover, experimental studies have shown that alveolarization continues beyond early life and into maturity in some mammals, and that it can be initiated by experimental pneumonectomy in mature animals. In humans, compensatory lung growth has been reported over a 15-year follow-up period after pneumonectomy in a 33-year-old woman for lung cancer. Moreover, it has been shown that Cobb angle correction does not correlate with either an increase in vital capacity or with a decrease in the spinal penetration index.
Evidence is beginning to accumulate that alveolarization may not be confined to early childhood, as previously thought, and may actually continue into maturity and beyond. Therefore, alveolarization during late childhood or adolescence may provide a repair mechanism for early insults to lung growth. 5 , 6
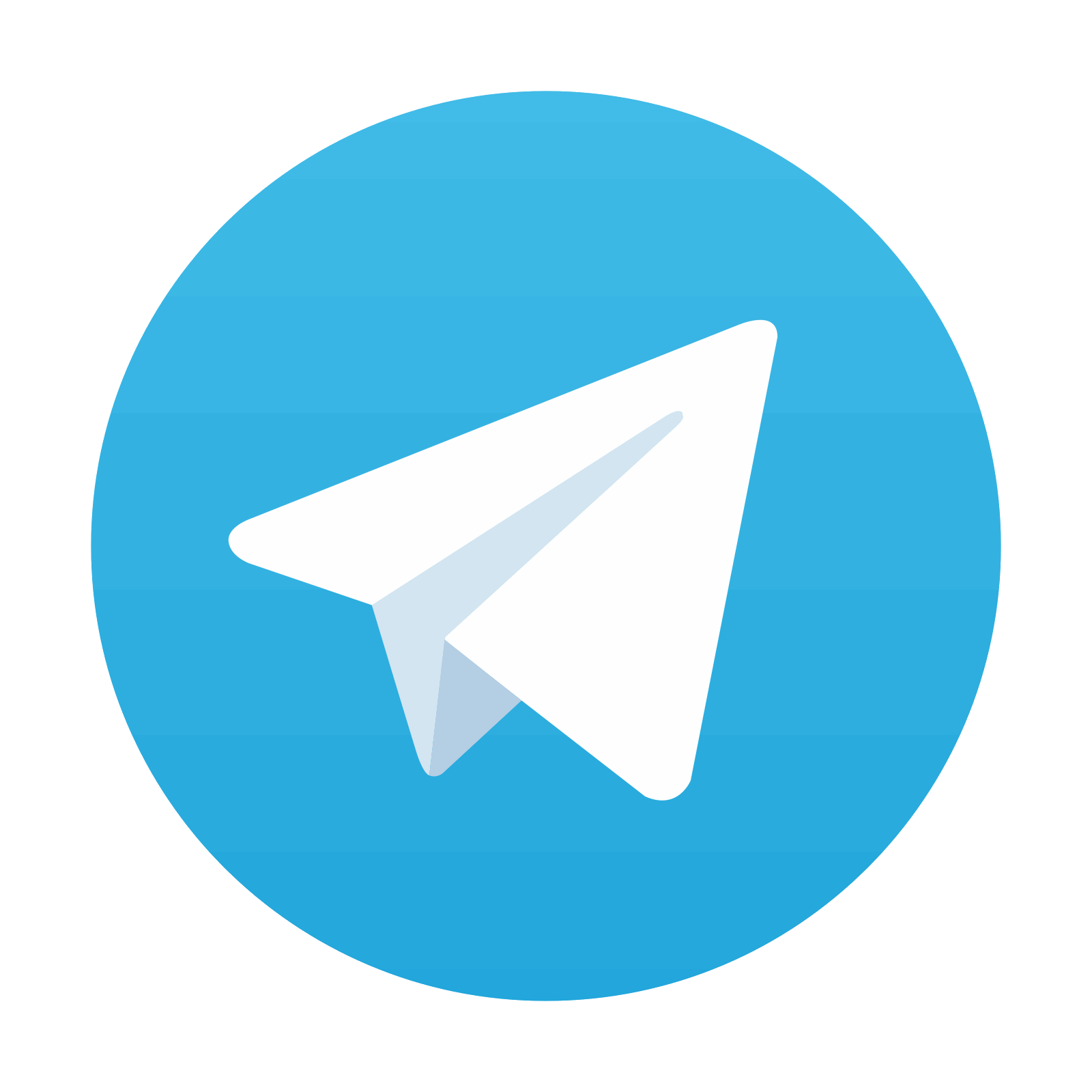
Stay updated, free articles. Join our Telegram channel

Full access? Get Clinical Tree
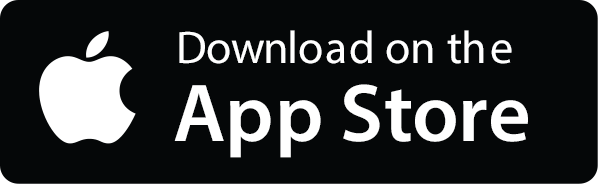
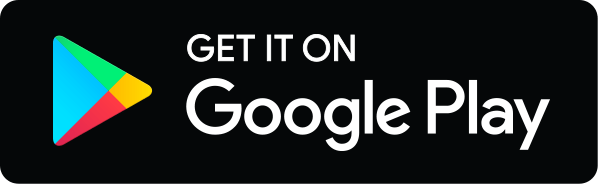