Abstract
Proprioception is defined as the conscious and unconscious sensations regulating posture, motion, and joint control. With regard to joint control, proprioception includes the senses of joint position and motion (kinesthesia) as well as feed-forward control. The foundation for a normal joint proprioception includes an intact mechanoreceptor innervation and afferent feedback to the spinal cord and the muscles controlling a joint. In the past decade, ample studies have detailed the mechanoreceptor innervation of the wrist, basal thumb and distal radioulnar joints. In addition, studies on joint reflexes have highlighted the existence of ligamento-muscular reflex arcs to provide both immediate, joint protective, control, as well as slower reflex response for muscle coordination.
In patients with advanced osteoarthritis, however, the innervation pattern in ligamens and synovium is altered, indicating a change in proprioceptive ability and a pattern of pain and inflammation rather than proprioception.
As clinicians, we are of course faced with a challenge when it comes to preserving innervation, but at the same time provide adequate pain relief to our patients. In this chapter, the foundation for hand and wrist proprioception is outlined, with a discussion on the considerations for our patients in need of joint arthroplasty.
Key words
Joint position sense – kinesthesia – ligament – neuromuscular control – proprioception – sensory nerve endings3 Proprioception and Neural Feedback in Thumb and Wrist Arthroplasty
Essential to a great discoverer, in any field of Nature, would seem an intuitive flair for raising the right question. To ask something which the time is not ripe to answer is of small avail. There must be the means for reply and enough collateral knowledge to make the answer worthwhile.
― Sir Charles Scott Sherrington
3.1 Basis of Proprioception for Joint Control
The quote by Sir Charles Scott Sherrington reflects the groundbreaking work that he produced in the early 1900s regarding neuroscience and proprioception; work which led to his Nobel Prize Award in Physiology or Medicine in 1932. His definition of proprioception in 1906 was: “sensations arising in the deep areas of the body, contributing to conscious sensations (muscle sense), total posture (postural equilibrium), and segmental posture (joint stability).” 1 Sherrington went on to further describe these senses as arising from “receptors in skin, joints and muscles.” 1 Based on his work more than a hundred years ago, the study of proprioception with regard to joint control has come to fruition in the past two to three decades. 2 As Sherrington said above, there must be “the means for reply and enough collateral knowledge to make the answer worthwhile.” What we know today, through global collateral research efforts, is that the proprioception senses may be divided into conscious and unconscious senses (Fig. 3.1), and that these both work in an integrated manner to provide joint control and neuromuscular stability.

3.1.1 Conscious Proprioception Senses
The conscious senses include somatosensory appreciation of touch and motion. For the former, the senses included are primarily tactility, vibration, and pain as arising from receptors in the skin. The latter include kinesthesia (the sense of joint motion), joint position sense, and the sense of muscle force and tension. The origin of the conscious joint proprioception senses is found in skin, muscle, and joint receptors. Skin receptors are primarily of importance in the kinesthesia of finger joints, 3 since the muscles controlling the fingers are located at a distance, in the forearm and hand. Joint receptors are similarly thought to be of importance whenever a muscle traverses more than one major joint, 4 thus limiting the sensitivity of the muscle spindle in detecting motion.
3.1.2 Unconscious Proprioception Senses
The unconscious proprioception senses are the senses related to neuromuscular control of posture, joint stability, and complex-coordinated muscle action, so called feed-forward control. 5 , 6 Unconscious joint proprioception is primarily influenced by receptors in ligaments and joint capsule, where afferent information from these highly specialized nerve-endings create spinal reflexes for immediate joint control as well as signals to the cerebellum for advanced motor control and planning/execution of joint stability and neuromuscular control. 7 , 8 To understand the complexity of wrist-and-hand joint proprioception, a description of sensory nerve endings and their distribution in the wrist and hand will follow.
3.1.3 Sensory Nerve Endings
The innervation of ligaments is characterized by specific sensory nerve endings, which can be classified due to their typical shape (Fig. 3.2) and neurophysiological traits (Table 3.1) according to Freeman and Wyke, 9 modified by Hagert. 10 Sensory nerve endings react to mechanical stimuli, e.g., extremes of ranges of motion, transform them into neural excitations, send this information from the joint via afferent nerves and dorsal root ganglia to the spinal cord, where one part of the information is send to the cerebellum and the cortex, and the other part of the information is used for local or segmental polysynaptic interactions. 6

According to the classification by Erlanger and Gasser 11 as well as Lloyd 12 and Hunt 13 for afferent and efferent nerve fibers (Table 3.1), the afferents, Ruffini endings, and Pacini corpuscles are myelinated group II and Aβ fibers with nerve conduction velocities of 36 to 72 m/s. Golgi-like endings of the periarticular tissue belong to group II, whereas the originally described Golgi tendon organs of the myotendinous junction are group Ib fibers. The afferents of free nerve endings are either thin myelinated group III and Aδ fibers with a conduction velocity between 4 and 36 m/s or unmyelinated group IV and C fibers with conduction velocities of 0.4 to 2 m/s. 14
Ruffini Endings
In the late 19th century the Italian histologist Angelo Ruffini 15 described this nerve ending in the skin, which is named after him. Synonyms for this corpuscle are dendritic or spray ending.
Microneurographic recordings from Ruffini endings in cat knee intracapsular ligaments have been shown to be slowly adapting low-threshold receptors that constantly respond during joint movement. 16 Ruffini endings detect static joint position, intra-articular and atmospheric pressure changes, as well as the direction, amplitude, and speed of active and passive joint movement. They respond to axial load and tension, but not to vertical compressive joint forces, highlighting their importance in specifying joint position and rotation. This is required for the regulation of periarticular muscle contracture and neuromuscular joint control. 17
Pacini Corpuscles
Although Pacini corpuscles are mentioned for the first time by Johannes Gottlieb Lehmann 18 in his doctoral thesis in 1741 and in the same year by the German anatomist and botanist Abraham Vater, 19 this discovery was forgotten for nearly a century. 20 The Italian anatomist Filippo Pacini 21 rediscovered this corpuscle in 1835, which was subsequently named after him. Pacinian corpuscles are fast adapting receptors with a low excitation threshold, which respond to joint accelerations and decelerations. 22
Golgi-like Endings
The Italian anatomist Camillo Golgi 23 discovered this sensory nerve ending initially as the “Golgi tendon organ” in the myotendinous junction in 1878. Named after him, the Golgi-like ending, which is found in ligaments, is a type of spray ending, which originates from the same family as Ruffini endings. Golgi-like endings are slow-adapting receptors, which, in contrast to Ruffini endings, have a high threshold for mechanical stress, making them completely inactive in immobile joints, but important for the detection of extremes of ranges of motion. 24
Free Nerve Endings
Free nerve endings are nonadaptive high-threshold nociceptors, which respond to different stimuli, e.g., chemical, mechanical, harmful, or inflammatory stimuli. They are mainly located in the interstitium of the densely packed collagen fibers, in the epiligament layer, and in the vicinity of blood vessels. 25 , 26
Unclassifiable Corpuscles
Corpuscles, which cannot be classified as Ruffini, Pacini, Golgi-like endings, or free nerve endings, are regarded as unclassifiable corpuscles, type V. 10 The function and physiological properties of nonclassifiable corpuscles are currently unknown.
3.2 Innervation Patterns (Fig. 3.3)

3.2.1 Wrist
Articular branches of the radial, ulnar (UN), median, and musculocutaneous nerves are the main contributors to wrist joint innervation. 27 The radial aspect of the radiocarpal joint is innervated by the lateral antebrachial cutaneous nerve (LACN), which is a terminal sensory branch of the musculocutaneous nerve. 28 The central two-thirds of the volar wrist capsule and the volar parts of the scapholunate interosseous ligament (SLIL) are innervated by the anterior interosseous nerve (AIN). 29 , 30 The palmar cutaneous branch (PCB) of the median nerve innervates the volar midcarpal area. 28 The triangular fibrocartilage complex (TFCC) is mainly innervated by the UN, including branches from the dorsal sensory branch of the UN. 31 The posterior interosseous nerve (PIN) innervates the dorsal wrist capsule, the dorsal aspects of the SLIL, the lunotriquetral interosseous ligament, and the dorsal radiocarpal and intercarpal ligaments. 28 , 29 Finally, the superficial sensory branch of the radial nerve (SBRN) provides few articular branches to the dorsal and volar radial wrist capsule. 28
3.2.2 Trapeziometacarpal Joint
Cadaveric dissections of trapeziometacarpal joint (TMJ) have shown that nerve branches to the TMJ arise from the LACN in 100% of specimens, the PCB of the median nerve in 70% of specimens, and the SBRN in 40% of specimens. 32 Furthermore, the dorsoradial, the dorsocentral, and the posterior oblique ligaments of the TMJ have an abundance of sensory nerve endings. 33 In contrast, little to no innervation have been seen in the anterior oblique ligament. Ruffini endings, which mediate joint position sense, are the predominant mechanoreceptor type with a greater density at the metacarpal insertion of the ligaments. 33 This indicates that joint position sense is an important proprioceptive quality in the highly mobile TMJ.
3.2.3 Distal Radioulnar Joint (DRUJ)
The TFCC is the main stabilizer of the distal radioulnar joint (DRUJ). 34 The seven parts of the TFCC, namely the subsheath of the extensor carpi ulnaris tendon sheath, the ulnocarpal meniscoid, the articular disk, as well as the dorsal and volar radioulnar, ulnolunate, and ulnotriquetral ligaments, are predominantly innervated by free nerve endings. 35
Since the articular disk and ulnolunate ligament are rarely innervated, both these structures primarily have mechanical functions. Both radioulnar ligaments are richly innervated by all types of sensory nerve endings. Furthermore, the volar radioulnar ligament has the highest number of Ruffini endings, indicating its role in mediating joint position sense. This indicates that both ligaments are important in proprioceptive control of the DRUJ during forearm rotation. The ulnotriquetral ligament, the subsheath of the extensor carpi ulnaris tendon sheath, and the ulnocarpal meniscoid have all types of sensory nerve endings and therefore distinct proprioceptive qualities. 35
3.3 Innervation Patterns in Osteoarthritis
Proprioception in hand osteoarthritis (OA) has primarily been studied in the TMJ. In an analysis of mechanoreceptor innervation in patients with painful TMJ OA, the innervation patterns were altered as compared to the innervation found in nonarthritic specimens. 36 Where Ruffini corpuscles dominate in the normal joint, the arthritic joint had a marked innervation of unclassifiable corpuscles and free nerve endings, indicating that the nerve endings that normally signal for joint stability and control are diminished and pain receptors dominate. 37 The lack of Ruffini and Pacini corpuscles in TMJ OA may explain why joint position sense has been shown to be impaired in patients with TMJ OA compared to healthy participants. 38
In a recent publication it was reported that the free nerve endings in tissues around TMJ OA are immunoreactive to markers of the autonomic, sensory, and glutamatergic pathways, which implies that pain in OA is caused by a polymodal neurogenic inflammation. 25 These different nociceptive pathways may explain why anti-inflammatory analgesics or intra-articular corticosteroid injections often help reduce OA-related pain. 39
3.4 Neural Feedback of the Wrist and Thumb
The intricate joint and mechanoreceptor innervation found in the wrist and TMJ supports the theory that the ligaments of the hand and wrist have an important sensory function in allowing dynamic neuromuscular control of joint stability. In studies on the ligamento-muscular reflexes of the wrist, it has been found that electrical stimulation of the dorsal SLIL elicits immediate, monosynaptic reflexes that serve as joint-protective reflexes. 40 Similarly, electrical stimulation of the dorsoradial ligament of the TMJ elicits joint-protective reflexes that serve to stabilize the TMJ and avoid subluxation of the joint. 41 These reflexes are akin to the anterior cruciate ligament and hamstring ligamento-muscular reflexes around the knee joint 42 that have been shown to have an important role in the proprioception and neuromuscular stability of the knee. 43
The joint-protective reflexes elicited in the SLIL were found to be entirely eliminated in healthy controls after desensitization of the PIN, 44 suggesting that denervation of a joint will have a major impact on the proprioception of the joint. However a recent publication investigating the effect of complete wrist denervation on wrist proprioception was not able to conclude a difference in proprioception between healthy individuals and patients that had undergone Wilhelm’s denervation procedure, assessed by wrist reflex time, wrist joint position sense, and force sense. 45 The conclusion in this study was that the tests used also stimulated skin receptors and Golgi endings in the musculotendinous unit, both of which are unaffected by wrist denervation.
3.5 Clinical Implications
The indication for thumb or wrist arthroplasty is, in general, to replace a painful/degenerated arthritic joint with an artificial implant that will eliminate/reduce pain while providing a high degree of mobility. The joint to be replaced is likely to have an altered innervation pattern with activated free nerve endings rather than specialized proprioceptors, 36 , 37 i.e., Ruffini and Pacini corpuscles, meaning reduced proprioception, while the goal of the postoperative rehabilitation is to regain neuromuscular control and proprioceptive function. 46 How should we handle this obvious dilemma; to denervate or reinnervate, that is the question!
3.5.1 The Case for Nerve-Sparing Surgery
A primary goal for arthroplasty surgery must be to cause as little harm as possible and to restore thumb/wrist function. A surgical approach aiming to preserve joint innervation will aid in both postoperative healing of tissues 47 , 48 and promoting restoration of proprioceptive function through guided rehabilitation. 46 In studies on arthroplasty of the knee it has been found that the arthroplasty itself promoted an improvement in knee proprioception—joint position sense, kinesthesia, and balance—as the arthroplasty surgery both reduced pain and realigned joint capsules and ligaments into more anatomical positions. 49
Similar findings may be anticipated for wrist and thumb arthroplasties. For instance, a retained innervation appears essential for optimal healing of vascularized joint transfers performed for proximal interphalangeal or metacarpophalangeal joint reconstruction. 50 In arthroplasty where the majority of the ligaments and joint capsule are retained, e.g., an ulnar head replacement, the preserved innervation of the TFCC should assist both healing and postoperative proprioceptive rehabilitation. Nerve-sparing surgical approaches have previously been described in detail for the wrist and DRUJ, 51 , 52 where the principle is easily understood: approach the joint with care and consideration for the nerve(s) innervating the joint. As in all of medicine, primum non nocere (cause no harm).
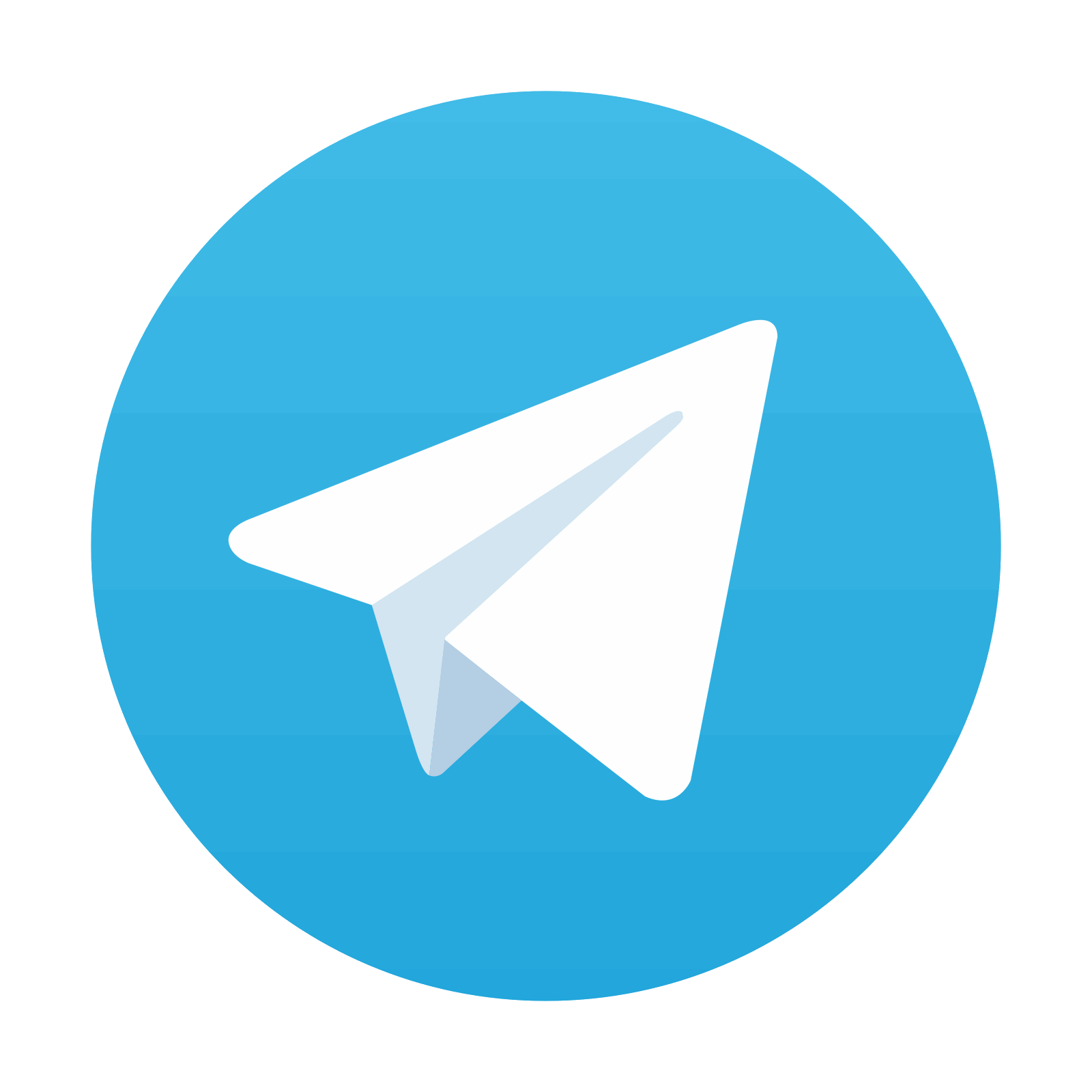
Stay updated, free articles. Join our Telegram channel

Full access? Get Clinical Tree
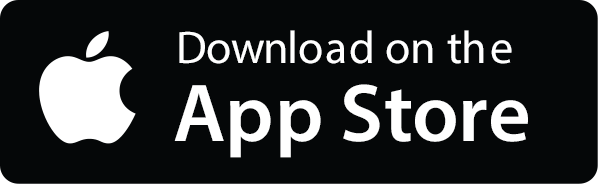
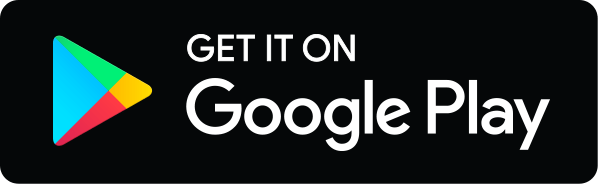
