Summary
Intraoperative neuromonitoring (IONM) techniques facilitate the detection and prevention of potentially devastating iatrogenic neurologic injury. Somatosensory evoked potential (SSEP) monitoring for detecting impending neurologic injury during scoliosis surgery was first reported in 1977 by Nash et al. 1 However, limitations of SSEPs, including an inability to provide a real-time assessment of the ventral motor pathways, led to the development of more sensitive and instantaneous markers of spinal cord integrity with transcranial motor evoked potentials (tcMEPs). TcMEPs are mediated by synapses in the ventral spinal cord and are highly sensitive to localized ischemic changes affecting the anterior horn of the spinal cord. SSEP monitoring is still helpful for its confirmatory role in the setting of negative tcMEPs, for providing information on the dorsal sensory cord, and for its ability to monitor the brachial plexus during prone positioning of the patient. Combined SSEP and MEP monitoring has been shown to have high rates of sensitivity and specificity, with rates as high as 100 and 98.5%, respectively, in one series. An intraoperative neurophysiologic alert may indicate a true physiologic event affecting the spinal cord, which has the potential to become a neurologic deficit if not addressed acutely. Return of IONM data after an alert may help guide the surgeon to safely complete spinal deformity surgery.
Key words
adolescent idiopathic scoliosis – intraoperative neuromonitoring – somatosensory evoked potentials – transcranial motor evoked potential – triggered electromyographic stimulation – delayed postoperative neurologic deficit28 Intraoperative Neuromonitoring
28.1 Introduction
Progress in operative techniques has allowed the spine surgeon to safely treat patients with increasingly complex spinal deformities. Intraoperative neuromonitoring (IONM) techniques facilitate the detection and prevention of potentially devastating iatrogenic neurologic injury. Over the last decade, there has been an increase in the use of IONM in spinal surgery by nearly 300%. 2 This chapter discusses the advantages and limitations of the various IONM tools available to the spinal deformity surgeon.
28.2 History
28.2.1 Stagnara Wake-up Test
In 1973, Vauzelle et al first introduced the Stagnara wake-up test, which has been widely used for assessing neurologic function intraoperatively. 3 The test involves a temporary reduction in anesthesia during which the patient is instructed to move the extremities, starting with the upper extremities and progressing to the lower extremities. Failure to move the lower extremities symmetrically indicates a neurologic injury. The benefit of the test is that it is a simple, cost-effective procedure that can be performed in the operating room and does not require the participation of a specialized team of trained neurophysiologists. Furthermore, it provides a direct measure of global motor function. Although some still consider the wake-up test the “gold standard” for intraoperative assessment of neurologic function, it has significant shortcomings. Potential complications related to the wake-up test include patient recall, accidental extubation, air embolism, pain, construct-rod dislocation, disruption of intravenous and intra-arterial lines, added surgical time, and false-negative and false-positive results. 4 , 5
28.2.2 Ankle Clonus Test
In 1997, Hoppenfeld et al described the ankle clonus test as a predictor of neurologic compromise following scoliosis surgery. 6 They reported that the absence of bilateral ankle clonus on the emergence of the patient from anesthesia is abnormal and indicates neurologic injury. The ankle clonus test is predicated on the normal presence of bilateral ankle clonus upon recovery from general anesthesia as a result of the return of lower-motor-neuron function before the return of inhibitory upper-motor-neuron impulses, leading to a temporary excitatory state. In Hoppenfeld et al’s review of 1,006 patients who underwent spinal arthrodesis and instrumentation for scoliosis, 6 patients had new neurologic deficits postoperatively, and all of them had a “positive” ankle clonus test. There were three false-positive findings but no false negatives. 6 Although this method of monitoring spinal cord function is cheap, sensitive, and easily performed, some have questioned its specificity, especially with inhalational anesthesia. 7
The major drawback of both the wake-up test and ankle clonus test is their failure to provide a real-time, continuous assessment of spinal cord integrity. Both tests reflect global spinal integrity and do not provide a real-time assessment of dorsal sensory or ventral motor cord tracts. The lag between injury and detection may jeopardize the small window of opportunity for intervention, resulting in a transient deficit becoming permanent. Such limitations have served as the impetus for the development of IONM, which provides a real-time indication of spinal cord integrity in patients undergoing corrective scoliosis surgery. Advances in neurophysiologic monitoring of the spinal cord complement improvements in corrective spinal instrumentation and design, facilitating improved care and safety that can be offered to patients with more complex spinal deformities.
28.3 Neuromonitoring
28.3.1 Somatosensory Evoked Potentials
Somatosensory evoked potential (SSEP) monitoring was first described over 70 years ago; however, its use in clinical practice for detecting impending neurologic injury during scoliosis surgery was not reported until 1977 by Nash et al. 1 , 8 SSEP monitoring represents the average of electrical responses to repetitive electrical or mechanical stimulation of a peripheral nerve. Signal transduction is mediated by large-diameter myelinated sensory fibers that traverse the peripheral nerve and then enter the spinal cord and ascend through the dorsal columns. After synapsing in the medullary nuclei of the brainstem, the neural signal crosses the brainstem and enters the medial lemniscal pathway. After another synapse in the thalamic nuclei, the signal proceeds to the parietal (sensorimotor) cortex. The afferent signal is then recorded at the level of either the spinal cord or, more commonly, the scalp (Fig. 28‑1). Monitoring of the upper extremities at the brachial plexus is best done by stimulating SSEPs in the ulnar nerve (Fig. 28‑2). 9 , 10 Monitoring of SSEPs in the lower extremities is typically done by stimulating the posterior tibial or peroneal nerves.


SSEP monitoring has several advantages over the ankle clonus and wake-up tests for spinal cord integrity. It is effective at reducing the rate of neurologic injuries below the rate found without IONM, it may detect injuries at the time of occurrence, and it may be performed on patients who are neurologically intact and those who are compromised. 11 , 12 Numerous studies have shown the efficacy of SSEP monitoring in reducing the rate of new neurologic deficits when used during surgery for scoliosis. 12 , 13 , 14 , 15 Nuwer et al found that experienced SSEP monitoring teams had fewer than one-half as many neurologic deficits per 100 cases compared to teams with little monitoring experience. 11 In their survey of 51,263 scoliosis procedures, the investigators reported that SSEP monitoring had a sensitivity of 92%, although it also had a relatively high rate of false-positive results. Overall, the rate of false-positive results with the use of SSEP monitoring averages 2%, with a reported range of 0 to 7%. 16 In 1992, the Scoliosis Research Society (SRS) issued a position statement advocating SSEP monitoring as the standard of care for scoliosis surgery (Scoliosis Research Society Position Statement. Somatosensory evoked potential monitoring of neurologic spinal cord function during spinal surgery. Scoliosis Research Society, Milwaukee, WI, September, 1992).
Despite its excellent clinical track record, SSEP monitoring has some limitations that are of concern among spinal deformity surgeons, including latency, high false-negative rates, and low sensitivity. 17 , 18 , 19 , 20 Because SSEP monitoring assesses the integrity of the dorsal columns, primary motor injuries of the ventral motor tracts of the spinal cord may go undetected. Pelosi et al reported a false-negative rate of 2.4% using SSEPs, as compared with no false negatives in the monitoring of transcranial motor evoked potentials (tcMEPs). 21 In the largest series to date comparing SSEP monitoring with tcMEP monitoring in adolescent idiopathic scoliosis (AIS), Schwartz et al found that SSEP monitoring failed to detect four of seven cases (57%) of new-onset motor deficits, whereas tcMEP monitoring detected all seven cases (Case Example 1). 20
In addition to its weaker sensitivity in detecting impending neurologic injury, it has been well documented that there is a delay in detecting ischemic injury with SSEPs as compared with tcMEPs (Case Example 2). Schwartz et al showed that the average time to detection of an intraoperative insult with SSEP monitoring lagged behind tcMEP monitoring by an average of 5 minutes, with the lag ranging from 0 to 10 minutes. 20 This relative delay in detecting ischemia with SSEP monitoring has also been reported in the literature with thoracoabdominal aneurysm (TAA) repair and, as a result, SSEP monitoring has been abandoned for the prevention of neurologic injury in surgery for TAA. 22 There are two potential explanations for the delay in detecting ischemia with SSEP monitoring: (1) SSEPs primarily monitor the dorsal sensory columns, and ischemia of the ventral cord and motor tract may only be inferred rather than measured directly; this is in contrast to the monitoring of tcMEPs, which correlate directly with the blood supply to the ventral cord and (2) unlike tcMEPs, SSEP impulses relay afferent sensory signals nonsynaptically, which may result in a lag between the onset of an ischemic injury and detection of the causative ischemic event because of the relative resistance of axonal conduction to ischemia. 22 , 23 In addition, Chen et al showed that there may be latency in obtaining baseline SSEPs, which may not be obtained until after incision. 24 When these factors are taken together, they show that SSEP monitoring alone is incapable of direct monitoring of vascular blood flow to the ventral cord in real-time and therefore at best serves as an indirect measure of the global ventral cord blood supply.
With the improved sensitivity afforded by the monitoring of tcMEPs, a residual role of SSEP monitoring of the spinal cord during AIS surgery may be to confirm the integrity of the cord in the setting of unchanged tcMEPs. 20 , 21 One important and unique contribution of SSEP monitoring is the monitoring of blood flow to the brachial plexus during the prone positioning of patients in scoliosis surgery. 15 Prolonged or inappropriate prone positioning is an increasingly recognized source of iatrogenic neurologic injury to the extremities in scoliosis surgery. 9 With the arm in abduction and with increasing axillary pressure, brachial plexopathy can occur. Typically, ulnar nerve SSEPs are recorded immediately after positioning of the arm in the flexed, abducted position. The point prevalence of positional brachial plexopathy reported in recent studies has ranged from 3.6 to 15%, depending on the criteria used for a significant change. 9 , 10 , 15 A reduction in SSEP amplitude of 30% indicates an impending injury and should prompt the surgical team to reposition the patient’s arm. 9 , 10 In 18 arms for which there was an intraoperative SSEP alert, repositioning resulted in a nearly immediate return of ulnar nerve SSEP tracings to baseline and in normal neurologic function upon awakening of the patient (Case Example 3). 10 Another study demonstrated that SSEP monitoring was 78% sensitive in detecting sensory deficits in the upper extremity, 100% sensitive in detecting combined sensory and motor deficits, and 98.5% specific in predicting a normal neurologic status postoperatively. 15 As a result, for routine AIS surgery, SSEP monitoring is recommended for its confirmatory role in the setting of negative tcMEPs, for providing information on the dorsal sensory cord, and for its ability to monitor the brachial plexus during prone positioning of the patient.
28.3.2 Transcranial Motor Evoked Potentials
Motor evoked potentials from transcranial stimulation of the motor cortex in humans was first described in the 1980s. 25 , 26 , 27 Transcranial electrical stimulation of the motor cortex generates an electrical impulse that descends the corticospinal tract (CST) and enters the peripheral muscle where the electrical impulse is recorded. Beginning with a transcranial electrical stimulus that delivers a brief (50 μs), high-voltage (250–500 V) train of anodal pulses (2–7 pulses, with an interstimulus interval of 1–5 ms), a neuroelectric signal is generated (Fig. 28‑3). Axons in the CST mediate the electrical impulse, which travels from the cortex through the internal capsule to the caudal medulla, where the fibers of the CST decussate and form the lateral CST. The signal then descends the CST in the lateral and anterior funiculi of the spinal cord. Upon entering the gray matter of the spinal cord, the axons of the CST interact with the interneurons of the spinal cord and synapse with α-motor neurons. The subsequent electrical responses are recorded at peripheral muscles, most commonly the anterior tibialis, although recording at the abductor hallucis, iliopsoas, quadriceps, and foot flexors has been described. 28 The first dorsal interosseous muscle is also monitored as a control to assess whether or not an intraoperative tcMEP alert represents global spinal cord dysfunction (reflected by reductions in the amplitude of tcMEPs in both the upper and lower extremities) or localized injury (in which only tcMEPs in the lower extremity are affected).

The neurophysiologic mechanism of signal transduction is most likely responsible for the preferential sensitivity of tcMEP over SSEP monitoring in the detection of motor deficits during scoliosis surgery. Although SSEPs in the lower extremity provide information on the dorsal columns, they do not reflect the integrity of the CSTs. As discussed previously, the dorsal columns consist of nonsynaptic axonal white matter, which is inherently more resistant to ischemia than is gray matter. 23 , 29 On the other hand, motor neurons in the anterior horn of the gray matter of the spinal cord have a high metabolic rate and are more vulnerable to ischemic changes. 22 , 23 TcMEPs, which are mediated by synapses in the ventral spinal cord, are thus highly sensitive to localized ischemic changes affecting the motor cells and interneurons of the anterior horn of the spinal cord. This is of particular clinical relevance because the vascular supply to the ventral motor pathways is less redundant than that to the dorsal columns, making the ventral motor tracts susceptible to cord ischemia (Case Example 4). During scoliosis surgery, hypotension and corrective maneuvers such as distraction and derotation may further compromise the local blood supply to the spinal cord. 21 On the basis of its sensitivity to ischemic changes affecting the ventral cord, tcMEP monitoring serves as a real-time neurophysiologic indicator of impending injury to the ventral motor tract. 18 , 20 , 22 , 30
TcMEP monitoring raises several unique safety concerns because it involves repetitive electrical stimulation of the brain. Some of the potential complications of tcMEP monitoring include brain damage, seizure activity, bite injuries, scalp burns, adverse cognitive or affective disorders, cardiac arrhythmias, and intraoperative awareness. In an analysis of the safety profile of tcMEP monitoring in more than 15,000 cases, Macdonald reported an exceedingly low complication rate. 31 He found the most common complication was tongue or lip laceration in 29 patients, which could be prevented with a properly inserted bite block. Other adverse events included pulse train or coincidental seizures (5 patients), mandibular fracture (1 patient), cardiac arrhythmia (5 patients), and minor scalp burns (2 patients). 31 To the best of our knowledge, the few reports of seizure as a consequence of tcMEP monitoring have involved unanesthetized patients with predisposing brain lesions. 31 , 32 In addition, tcMEP monitoring does not appear to increase the risk of intraoperative or postoperative seizures in patients with epilepsy following spinal deformity surgery. 33 Relative contraindications to tcMEP monitoring include epilepsy, cortical lesions, cardiac disease, vascular clips or shunts, and implanted cardiac pacemakers. 31
Numerous studies document the efficacy of tcMEP monitoring for detecting impending neurologic injury during scoliosis surgery. 20 , 21 , 22 , 30 , 34 Schwartz et al reported the largest series of scoliosis surgeries in which tcMEP monitoring was used in 1,121 patients with AIS. 20 Seventeen patients experienced depression of tcMEPs > 65% (alert level) without any changes in SSEPs. Seven patients (0.6%) experienced transient motor deficits, all of which were identified during surgery and addressed. All neurologic deficits resolved within 90 days, and the majority (six of nine cases) resolved within 7 days. Muchow et al compared 38 patients with spinal cord pathology (15 Chiari malformations, 12 syrinx, 7 tethered cords, and 4 spinal cord tumors) with 184 patients with AIS and showed that tcMEPs can be safely obtained in patients with neuraxis abnormalities and can be used to accurately identify impending neurologic deficits with high sensitivity in this patient population. 35 However, in 2017, Mo et al demonstrated that in patients with cerebral palsy, neuroanatomic findings of periventricular leukomalacia, hydrocephalus, and encephalomalacia are significant predictors of limited IONM signals, especially tcMEPs. 36
Traditionally, the outcome that is assessed in evaluating the efficacy of spinal cord monitoring is whether or not the patient awakens with a new neurologic deficit (a true positive). A false-positive alert is therefore defined as an intraoperative alert in which a patient has a normal neurologic examination upon awakening. However, an intraoperative neurophysiologic alert, especially one involving tcMEP monitoring, may indicate a true physiologic event affecting the spinal cord (i.e., ischemia), which has the potential to become a neurologic deficit if not addressed acutely. This is based on numerous animal and clinical studies demonstrating the relationship between spinal cord ischemia (a true physiologic event), a significant tcMEP alert, and the onset of new neurologic injuries. 37 In 2002, Noonan et al showed that lability in mean arterial pressure (MAP) may lead to higher false-positive rates. 38 Schwartz et al showed that up to 74% of tcMEP alerts may be attributable to a mechanism of spinal cord ischemia arising from systemic hypotension, distraction, or derotation maneuvers, in which 32% of patients awakened with a neurologic injury of new onset. 20 It can therefore be concluded that the ischemic cord is actually “at risk” of permanent injury and that a tcMEP alert provides an opportunity to correct a threatening neurologic deficit. Although this is difficult to prove in a controlled study, false-positive tcMEP readings are likely to represent a true positive made “false” by appropriate and timely intervention.
28.3.3 Multimodality
Combined SSEP and MEP monitoring has been shown to have high rates of sensitivity and specificity, as high as 100 and 98.5%, respectively, in one series. 21 , 30 , 39 , 40 Zuccaro et al reported a true-positive rate of 0.9% in 7 of 806 pediatric patients undergoing spinal deformity surgery at a single institution with no false-negative or false-positive outcomes. 40 As a result, many authors consider this to be the standard of care in scoliosis surgery. 40 In 2010, Thuet et al reviewed 3,436 consecutive pediatric orthopaedic spinal surgery patients receiving multimodal neuromonitoring and noted that it allowed accurate detection of permanent neurologic status in 99.6% of patients and reduced the total number of permanent neurologic injuries to 6 patients (0.17%) after intraoperative intervention. 41 However, in a larger study by Raynor et al, multimodal intraoperative monitoring failed to identify 45 patients (0.36%) with false-negative outcomes out of 12,375 patients. 42 Thus, multimodal IONM is currently the most sensitive and specific tool the spinal surgeon has to identify pending neurologic injuries, but it is not absolute, and there continues to be variation in use.
28.3.4 Triggered Electromyography
Intraoperative evaluation of the integrity of the pedicle and pedicle screw position before and after screw placement is a three-step procedure involving manual palpation of the ventral, medial, lateral, superior, and inferior pedicle walls with a pedicle probe or feeler; intraoperative imaging 43 ; and electromyographic (EMG) stimulation. Pedicle screw constructs are more common today as numerous clinical and biomechanical studies have shown improved rotational correction, improved fixation, and reduced loss of correction with their use as compared with hook or sublaminar wire constructs. 44 , 45 , 46 , 47 , 48 Given the smaller pedicle diameter in the thoracic spine, variable pedicle angle, the proximity of the great vessels, thoracic spinal nerve roots, and spinal cord, the risk of a pedicle breach and injury of adjacent structures is greater than that in the lumbar spine. 49 , 50 , 51 EMG monitoring has been used as a means of improving patient safety during the placement of both thoracic and lumbar pedicle screws. 49 , 50
Early cadaveric studies revealed a rate of thoracic cortical violation of 12.5 to 54.7% with thoracic pedicle screw placement. 52 , 53 , 54 Lehman et al used postoperative computed tomography (CT) to evaluate the thoracic positioning of 1,023 pedicle screws placed in 60 patients with spinal deformity using the freehand technique and reported an 89.5% rate of success without any neurologic, vascular, or visceral complications. 55 Samdani et al demonstrated a similar rate of successful screw placement at 87.9%; however, they also noted that surgeons with more than 5 years of experience had the lowest rate of medial wall breaches. 56 Reported clinical sequelae of erroneous pedicle screw placement include incidental durotomies, nerve root irritation, pedicle fracture, and leakage of cerebrospinal fluid (CSF). 49 , 50
EMG monitoring is done by placing subdermal needle electrodes into the muscle groups innervated by the spinal nerves relevant to the spinal level. The regimen in EMG monitoring consists of two components: (1) monitoring of spontaneous EMGs (spEMGs) and (2) monitoring of stimulated EMGs (stEMGs). Monitoring of spEMGs involves the continuous acquisition of data from spontaneous muscle activity at rest. With chronic nerve root compression, impulse train activity is the most common pattern seen in spEMG recordings. More acute changes in spEMG activity may manifest in the form of burst or train activity resulting from mechanical stretching, retraction, or sudden compression of a nerve root. The major contribution of spEMG monitoring is its ability to instantly notify the surgical team about a nerve root insult. It does not, however, provide information about the conductive capacity of the nerve root after insult or injury. The frequency of postoperative neurologic injuries following spEMG alerts is low, indicating a low specificity for this technique. 57 , 58
stEMG monitoring involves the electrical stimulation of a pedicle channel or screw and the recording of the stimulation threshold needed to trigger a compound muscle action potential (CMAP). 58 The stimulating probe is placed into the pedicle screw channel or on the surface of the pedicle screw, and a threshold potential for stimulation of a CMAP is recorded. The rationale behind stEMG monitoring is that the electrical stimulus required to activate the neighboring nerve root is proportional to the electrical impedance characteristics of the pedicle bone. If the pedicle is intact, there is greater resistance to current flow resulting in a high threshold for screw stimulation. If there is violation of the pedicle, the resistance to current flow is reduced and the screw stimulation threshold will be lower. 49 , 50 , 59 Several studies have confirmed a significant correlation between low pedicle screw stimulation thresholds and misdirected placement of lumbar pedicle screws. 60 , 61
It is important to maintain proper technique when performing stEMG to avoid false-recorded values. Steps should be taken in every case to ensure that no soft tissue is in contact with the EMG probe, which can artificially increase the stimulation threshold; to stimulate circumferentially inside the pedicle and vertebral body, being aware that in patients with osteoporosis or poor bone quality a low impedance value can be seen despite the pedicle being intact; and apply the cathode stimulator probe to the hexagonal screw port or directly to the shank of the pedicle screw, and not to the mobile crown, to avoid a false-negative EMG result. 62 , 63
Studies suggest the negative predictive value of lumbar pedicle screw monitoring with stEMG has been 98% when the impedance values have been greater than 11 mA. 50 , 64 If the threshold of stimulation is low (<6 mA), a pedicle breach is more likely. Impedance values between 7 and 11 mA represent a possible pedicle breach. 60 , 61 , 65 In 2002, Raynor et al reported that all screws with stimulation thresholds greater than 6 mA were safely implanted, without breaching the medial pedicle wall. For screws with a stimulation threshold of less than 6 mA, they recommended concomitant evaluation of the “average” of all other screw stimulation thresholds in a given patient and suspicion of a pedicle wall breach if the threshold is 60 to 65% below the patient’s average threshold. 49 In a later study, Raynor et al analyzed the EMG results for the placement of 4,857 lumbar pedicle screws and established 8.0 mA as a threshold above which the stimulated screw was very likely to be present in an intact pedicle (a 0.33% false-negative rate). 66 At lower thresholds of stimulation, they recommended removal of the relevant screw and repeated palpation to evaluate for a pedicle breach. 66 There needs to be a balance as the higher the threshold of EMG stimulation, the less likely a pedicle is to have been breached, but using a high threshold of stimulation as the criterion for repeat palpation carries the risk of increasing the frequency of false-positive results and unnecessary repeat palpations.
In 2016, Mikula et al performed a meta-analysis of 18 studies in which EMG was used during pedicle screw placement of 15,065 screws in 2,932 patients. They found an overall sensitivity for detecting misplaced pedicle screws of 0.78 and a specificity of 0.94, with a threshold of 10 to 12 mA. 67 However, Samdani et al retrospectively reviewed 50 patients (937 pedicle screws) undergoing posterior spinal fusion (PSF) for AIS and found 47 breaches with a mean threshold stimulus of 10.2 mA. After subgroup analysis, they concluded that EMG may not be reliable in detecting medial breaches from T2 to T9 but may have some utility from T10 to T12. 68 Thus, it is important to not always rely on impedance values and use good clinical judgment as well as intraoperative imaging when deciding if a screw has been breached.
28.3.5 Descending Neurogenic Evoked Potentials
Descending neurogenic evoked potentials (dNEPs) involve recording motor evoked potentials from peripheral nerves or muscles via stimulation of the spinal cord. 69 The stimulus can be either indirect with the placement of needles into spinous processes or direct with insertion of an epidural catheter onto the dura. 70 Initially, dNEPs were thought to monitor motor activity, but Minahan et al showed that they actually record dorsal column activity. 71 As a result, they may be useful in patients with cerebral palsy who have poor motor activity at baseline and may be used to identify an area of spinal cord deficit by stimulating multiple points along the spinal cord.
28.3.6 H-Reflex
The H-reflex is a monosynaptic reflex produced by stimulation of the afferent fibers of the S1 nerve root (tibial nerve) in the popliteal fossa. This segmental reflex provides a measure of the excitability of the motor neuron pool within the gray matter and can be used to assess the integrity of both afferent and efferent neural connections. 72 The afferent volley traverses the mixed peripheral nerve via group Ia fibers and exits via motor neurons that transmit the efferent signal to the muscle (gastrocnemius or soleus), where the signal is detected. Defects in this reflex pattern classically correlate with S1 radiculopathies and have been shown to reflect the integrity of the motor pathways of the more rostral spinal cord. The H-reflex is mediated over a long course (peripheral nerve, spinal cord, sacral plexus, and tibial and sciatic nerves), and injury at any point along this pathway can therefore cause an abnormal H-reflex. Animal and clinical studies in humans also suggest that the H-reflex can provide immediate feedback about the status of the descending motor tracts of the ventral spinal cord. 73 , 74 , 75 , 76 A transient change in the H-reflex warns of an impending neurologic injury, and permanent suppression of the H-reflex predicts postoperative neurologic deficits. 75
Leis et al investigated a group of 31 patients undergoing spinal cord surgery and found that 4 of 6 patients who had temporary changes in the H-reflex amplitude of less than 50% (which resolved with intraoperative measures) were neurologically normal upon awakening from anesthesia. The two patients in whom the amplitude of the H-reflex was permanently suppressed by greater than 90% were noted to have severe postoperative neurologic deficits. 75
Mechanical perturbation of the spinal cord produces nearly instantaneous changes in the H-reflex, corroborating the findings in animal studies of rapid changes in the H-reflex with spinal cord insults. 73 , 74 , 76
Some authors have proposed that by providing almost instantaneous warning of potential motor tract injury in the spinal cord, and with changes in amplitude corresponding with neurologic outcome, changes in the H-reflex may reflect the severity of spinal cord injury. Other potential advantages of H-reflex monitoring include a relative resistance of H-reflex wave recording to suppression by general anesthesia and the ability to detect subtle sensory deficits in the S1 nerve root. 75
Although there have been reports of the successful use of H-reflex monitoring in scoliosis surgery, 77 , 78 , 79 several limitations have precluded its use in common practice in such surgery. H-reflexes are commonly absent in patients older than 60 years or who have had a prior laminectomy. In a patient with a baseline deficit of the S1 nerve root, monitoring of the reflex will not be capable of detecting new spinal cord injury. As with other monitoring techniques, the use and interpretation of the H-reflex is highly operator and technique dependent, and its results may therefore be ambiguous in inexperienced hands. Consequently, monitoring of the H-reflex is currently limited to a role complementary to that of EMG monitoring with the use of more established modalities for spinal cord monitoring in AIS surgery.
28.3.7 D-Wave Monitoring
The D-wave is evoked by direct activation of the pyramidal axons and measured with an epidural recording catheter and has been considered another means of monitoring the functional integrity of the CST (Fig. 28‑4). Variations greater than 20% in D-wave amplitude are evidence of impending neurologic injury. 80 In patients undergoing surgery for intramedullary spinal cord tumors, variations greater than 50% in D-wave amplitude correlate with poor outcome.

Reports of the efficacy of D-wave monitoring of the spinal cord in scoliosis surgery have described mixed results. 81 Ulkatan et al recently reported that 27% of 93 patients with scoliosis who underwent D-wave and tcMEP monitoring showed significant alterations in D-wave activity without any changes in tcMEP or SSEP tracings or changes on postoperative neurologic examination. The changes in D-wave activity occurred immediately after correction of the scoliosis. On the basis of findings with magnetic resonance imaging (MRI) that revealed displacement of the spinal cord toward the curve concavity in scoliosis, they postulated that the reason for D-wave variability in scoliosis surgery is the new spatial relationship between the spinal cord and spinal canal that occurs with curve correction. 80 In addition to the difficulty in achieving the requisite midline position of the epidural recording catheter used in D-wave monitoring, the technique also carries the risk of causing epidural complications due to placement of the catheter with a laminectomy or laminotomy. Continued research is needed to further define the role of D-wave monitoring in monitoring spinal cord function during surgery for scoliosis.
28.4 Anesthesia and Other Agents
28.4.1 Inhalational Agents
Several significant considerations relating to the operating room environment and anesthesia can affect the stability and clarity of both SSEP and tcMEP monitoring. Inhalational anesthetic agents such as desflurane, sevoflurane, isoflurane, and nitrous oxide have a dose-response effect on SSEP monitoring. 82 , 83 The depressant effect of inhalational anesthetic agents is manifested both by a reduction in the latency of SSEP signals and, more importantly, by degradation of the cortical SSEP wave, with one study reporting a 50% reduction in SSEP amplitude with nitrous oxide. 22 , 84 , 85 In 2010, Sloan et al showed there was a synergistic effect of isoflurane and nitrous oxide causing more amplitude and latency changes than expected from a proportionate mixture of the individual agents during SSEP neuromonitoring. 86 Similarly, tcMEP signals are highly suppressed by inhalational anesthetics. 22 , 87 , 88 Volatile inhalational anesthetics decrease the excitability of both cortical and spinal cord motor neurons in tcMEP monitoring. With increased variability and reduced reliability in both SSEP and tcMEP signal tracing, the risk of ambiguity in signal interpretation with inhalational agents is significantly increased.
28.4.2 Total Intravenous Anesthesia
Efforts to reduce the risk of false-positive alerts and unreliable signal baselines in surgery for AIS commonly consist of using total intravenous anesthesia (TIVA) and avoiding the use of paralytic agents. In our institution, a standardized anesthesia protocol is strictly followed. After induction and intubation, all inhalational agents and muscle relaxants are discontinued. Throughout surgery, general anesthesia is maintained by the pump-controlled intravenous infusion of propofol and remifentanil and the maintenance of an MAP ≥65 mm Hg especially during curve correction maneuvers. Remifentanil has an extremely short half-life, and when infused at a constant rate produces constant plasma concentrations and minimal variability in the tcMEP response, although in high doses it has been shown to affect SSEP amplitude. 87 , 89 Occasional boluses of narcotic agents are provided as needed. Although the specifics of an anesthesia regimen for AIS surgery may vary, adhering to these principles will minimize signal variability and maximize the neurophysiologist’s ability to provide reliable IONM.
28.5 Other Considerations
Elements of the operating room environment and physiologic considerations that might influence the intraoperative quality of IONM signals include external 60-Hz noise and artifacts from electronic beds, warming blankets, poor electrical grounding, surgical headlights, microscopes, blood warmers, and electric drills. 90 Additionally, hypothermia has been shown to delay the rate of synaptic transmission in SSEP monitoring, which may affect SSEP latency but not amplitude. 91 As discussed earlier, hypotension may have an additive effect on iatrogenic spinal cord ischemia produced by distraction, compression, derotation, or vessel ligation. 22
28.5.1 Steroids
The decision to administer steroids according to the protocol for spinal cord injury as well as the threshold required to initiate the protocol is determined on a case-by-case basis and remains controversial. The use of steroids has not been extensively studied in the intraoperative setting. Previously, it was thought to have some benefit in acute spinal cord injury if administered within 8 hours of injury. 92 , 93 , 94 Methylprednisolone has been shown to reduce inflammation and stabilize cell membranes after spinal cord injury by inhibiting lipid peroxidation and calcium influx. 95 , 96 More recent literature suggests that steroids may cause gastrointestinal hemorrhage and increase the risk of infection. 97 As a result, in 2013, the American Association of Neurological Surgeons/Congress of Neurological Surgeons Joint Section on Disorders of the Spine and Peripheral Nerves Guidelines Committee recommended against the use of steroids in patients with acute spinal cord injuries due to potentially harmful side effects (including death) that outweigh the clinical benefits. 94 , 98 , 99
28.6 Change in Intraoperative Neuromonitoring
28.6.1 Significant Change
Once baseline signals are obtained, there are no absolute standards about what constitutes an alert. Traditionally, a loss of 75% in signal amplitude suggests a transient injury that in most cases will resolve. 100 However, some authors advocate greater than 50% decrease in unilateral or bilateral tcMEP amplitude as the criterion for an alert, whereas others use a decrease in amplitude of 65, 70, or 80% as a cutoff of significant change. 21 In 2003, Langeloo et al studied tcMEP monitoring in 145 patients and showed that an amplitude decrease of greater than 80% achieved a sensitivity of 1.0% and a specificity of 0.91%. 34 More recently, the Japanese Society for Spine Surgery and Related Research recommended a 70% decrease in amplitude of tcMEPs as an alarm point by studying a prospective multicenter group of 959 cases of spinal deformity, spinal cord tumor, and ossification of the posterior longitudinal ligament. 101 With this cutoff, the group was able to provide a high sensitivity (95%) and specificity (91%). 101 Our institutional guidelines allow a procedure to continue with caution upon a return of 50% in the amplitude of either SSEP or tcMEP signals. It should be noted that although a lower cutoff may produce fewer false negatives, it might also create extra stress and instigate additional steps to identify and correct the cause of the alert.
28.6.2 Team Response to Intraoperative Neuromonitoring Alert
As a result of an IONM alert, the team response may involve a pause in surgery, raising the patient’s MAPs to greater than 85 mm Hg, 102 reduction in the degree of spinal correction, administration of steroids, or removal of instrumentation. A review of 38 intraoperative IONM alerts revealed that the most common team response was to correct hypotension to at least 85 mm Hg, followed by a pause in surgery (45% of cases and averaging 8.7 minutes in duration), steroid administration, the removal of spinal instrumentation, reduction of spinal correction, and administration of the wake-up test. 20 More recently, Ferguson et al reported an incidence of significant neuromonitoring changes of 9.1% in 47 of 519 pediatric patients undergoing spinal deformity correction. 103 In response to these changes, wake-up tests were performed in 79% (37/47) of cases, instrumentation was adjusted in 32% (15/47), anesthesia was adjusted in 11% (5/47), implants were removed in 11% (5/47), the patient was repositioned in 6% (3/47), and the procedure was aborted in 28% (13/47). 103
Although a detailed description of the treatment algorithms used in response to an IONM alert is beyond the scope of this chapter, we present several guidelines used in our institution. Fig. 28‑5 depicts the IONM protocol used for AIS patients instrumented with pedicle screw systems. In 2014, Vitale et al introduced a consensus-based checklist to guide surgeon responses to IONM changes in patients undergoing spine surgery, which has been instituted in many centers and is considered best practice. 104 Fig. 28‑6 shows the checklist that has been modified slightly for our institution.


At our institution, the team response to an alert is a surgical pause and confirmation that the reduced IONM amplitude is not being caused by an anesthetic agent or technical factors in monitoring. Simultaneously, the patient’s mean arterial pressure (MAP) is raised to at least 80 mm Hg through volume repletion, possibly accompanied by packed red blood cell transfusion to raise the hematocrit and, if necessary, by pharmacologic modulation. 91 Patient body temperature should also be elevated to greater than 36.5°C (97.7°F) to help optimize neuromonitoring. 91
The institutional protocol and practitioner response to an IONM alert may vary if the amplitude of the signal continues to be decreased. If changes in neuromonitoring are concerning for a persistent neurologic deficit, a confirmatory wake-up test should be considered in a patient who is capable of following directions. If the wake-up test is positive and/or the alert coincided with curve correction or with the placement of an implant, some of the surgical corrections may be released or the relevant anchor may be removed, respectively. 91 Should the IONM tracings still suggest an impending neurologic injury, placement of all pedicle screws should be examined. Fluoroscopy may be used to evaluate screw position with a pedicle screw tip past the midline of the vertebral body on posteroanterior (PA) radiographs suggestive of a medial breach. 105 A triggered EMG threshold less than 60% is also suggestive of a possible pedicle breech. 49 In the presence of any of these signs, the screw should be removed to reassess the tract with direct palpation; in addition, a small laminotomy may be performed to evaluate the integrity of the medial pedicle cortex. 105
If signals still do not return, removal of all instrumentation with abortion of the surgery should be considered. In the event that removal of instrumentation is indicated, introducing the potential for spinal instability, the patient should be put in a brace postoperatively. Removal of implants may decrease artifact when obtaining postoperative CT or MRI scans to evaluate implant positioning or cord compression but is not required. Return to the operating room for reinstrumentation is based on the results of the postoperative neurologic examination and imaging and may even be delayed for as long as 2 or 3 weeks if there is no identifiable site of compression. The case examples given at the end of the chapter are useful for discussing the management of several of the more commonly encountered situations in which IONM alerts may occur.
28.6.3 Timing of Change and Time to Return
Recently, Phillips et al showed that in patients with early-onset scoliosis undergoing spinal deformity surgery there is a 12% rate of a significant neuromonitoring change which may occur at any stage of surgical management including prone positioning and may actually be under-reported. 106 In 2017, Zuccaro et al reviewed 806 patients undergoing pediatric spinal deformity surgery with IONM, noting that alerts occurred during rod correction (34% of cases), thoracic screw placement (25%), osteotomies (20%), resections (17%), cage insertion (3%), and sublaminar wiring procedures (2%). 40 Skaggs et al performed a retrospective review of patients with severe scoliosis over 80 degrees treated with posterior-only spinal fusion with temporary distraction rods and found that 9 of 22 patients (41%) had IONM changes that were reversed after releasing distraction on the temporary rod, except in one case. 107
28.6.4 Rate of Injury in Adolescent Idiopathic Scoliosis
In 2017, Bartley et al reviewed a prospectively collected multicenter registry of patients who underwent surgical treatment of AIS to identify perioperative surgical complication rates and found that although neurologic complications were rare (0.5%), they were still the second most common perioperative complication following wound-related complications (1%). 108 The reported incidence of spinal cord injury in scoliosis surgery varies from 0.3 to 1.4%. 64 , 109 , 110 , 111 Hamilton et al 64 studied the SRS morbidity and mortality (SRS M&M) database, which showed a significantly higher rate of new neurologic deficits in revision cases (1.25%) compared with primary cases (0.89%). In 2016, Burton et al studied the SRS M&M database and reported an increase in neurologic deficit rates from 2009 (0.44%) to 2012 (0.79%), with the highest rates in dysplastic spondylolisthesis and congenital kyphosis. 109 They concluded that this could be due to a number of factors, including more rigorous reporting. 64
28.6.5 Risk Factors for Changes in Intraoperative Neuromonitoring
A variety of mechanisms may account for spinal cord injury during corrective scoliosis surgery including misdirected wires, hooks, or pedicle screws that may cause direct trauma to the cord. 100 In 2006, Coe et al published a report showing that combined procedures (anterior and posterior) had statistically significant higher neurologic complication rates compared to anterior- or posterior-only approaches. 110 In addition, occlusion of segmental vessels during anterior procedures may cause ischemia of the cord, 112 , 113 which may be compounded by the correction of scoliosis that distracts the spinal cord and compromises local blood supply. 114 In 2010, Vitale et al reviewed the records of 162 consecutive patients who underwent surgery for the treatment of spinal deformity and found that 12 of 151 patients with successful monitoring had a true electrophysiologic event, with curve correction being the most common cause in 8 of the patients. 100 A decreased MAP (<60 mm Hg) and a low hemoglobin concentration may also exacerbate perfusion to the spinal cord leading to higher false-positive rates with multimodal IONM. 38
In 2010, Kamerlink et al assessed the preoperative neurologic risk in a consecutive series of 301 spinal deformity patients undergoing corrective surgery and found that patients with neuromuscular scoliosis and revision sagittal plane deformities had the highest incidence of neuromonitoring changes, at 10 and 9.8%, respectively. 115 Others have shown that osteotomies, major coronal curve angles greater than 90 degrees, and preoperative kyphosis were correlated with a higher incidence of neuromonitoring changes. Buckwalter et al analyzed a prospectively collected multicenter database of patients with scoliosis treated with spine surgery with IONM and found a 3.6% rate of significant neuromonitoring changes (due to low blood pressure, misplaced screws, and traction) with no postoperative neurologic deficits when a cause was identified. 116 Recently, we studied a similar cohort of 676 patients with AIS who underwent spinal arthrodesis with the use of IONM and found significant alerts (a ≥50% drop in SSEPs and/or tcMEPs) occurred in 5.3% of the patients. The patients with significant alerts had larger preoperative deformity, longer operative duration, a greater number of levels fused, a higher estimated blood loss, and a greater volume of autologous blood transfused. 117 Interestingly, we found that return of IONM data guided the surgeon to safely complete the procedure in 34 of 36 patients, with correction similar to that of patients who did not experience an alert. 117
28.6.6 Delayed Postoperative Neurologic Deficit
A delayed postoperative neurologic deficit (DPND) is one of the most feared complications following surgery for AIS. 118 In 2016, Auerbach et al surveyed members of the SRS and found that a DPND occurs at an estimated incidence of 0.01%. 119 Despite an uneventful surgery, unremarkable intraoperative monitoring profile, and normal postoperative neurologic examination, a DPND is characterized by the development of postoperative paresis within hours or days after a surgical procedure. 120 , 121 , 122 Auerbach et al found that 63% of cases occurred within the first 24 hours and 90% within 48 hours of surgery. 119 Complete (41%) or partial (26%) neurologic recovery may be expected, especially in compression-related DPND emphasizing the need for vigilant postoperative examination and early intervention. 119
Possible reasons for a DPND include postoperative spinal cord swelling and vascular spasm with resultant ischemia. In such cases, IONM shows no changes in tcMEPs. Progressive neurologic decline often prompts emergent return to the operating room for exploration and the removal of corrective implants. In such situations, a loss of tcMEP signals may corroborate the change in neurologic status during revision surgery. A second potential mechanism for DPND is delayed ischemia of the spinal cord following distractive and derotational forces applied to the spine. 123 Similarly, prolonged intraoperative hypotension during instrumentation and curve correction can predispose to a later ischemic event. For these reasons, we routinely recommend that the patient’s MAP remain above 65 mm Hg, and patients who are dehydrated should be delayed tetraparesis in a patient after PSF for AIS due to venous congestive myelopathy following an arteriovenous shunt of an unrecognized syrinx, which may have been discovered with a preoperative MRI. 124 A further mechanism for DPND is an epidural hematoma that can create mechanical compression of the cord or nerve roots, resulting in neural ischemia. 120
Management of a suspected DPND should begin with a careful neurovascular examination of the patient. This should include a detailed motor and sensory examination of the lower extremities with evaluation of bowel and bladder function, peroneal and perirectal sensation, and reflex function. If implants containing titanium alloys are used, MRI is a feasible imaging option for detecting problems as the decreased ferromagnetic properties of titanium reduce scatter and distortion of the image. 125 Alternatively, CT myelography can be used to evaluate whether or not an epidural hematoma is present or if there is compression of neurologic structures adjacent to an instrumentation component. In some instances, the treatment plan may warrant an urgent return to the operating room before imaging is done. The treatment plan must be individualized and devised on a case-by-case basis. 120 , 126 Treatment by partial removal of implants and staged correction has been shown to be effective for facilitating full neurologic recovery (Case Example 5). 12 , 120 , 122 , 127 When DPND is attributable to an epidural hematoma, we recommend reinstrumentation with pedicle screws instead of sublaminar wires or hooks to minimize the risk of recurrent bleeding.
Measures that can be taken to maximize postoperative spinal cord perfusion and reduce the risk of DPND include the following: (1) aggressive preoperative hydration and volume repletion of the patient to keep the MAP > 65 mm Hg during instrumentation; (2) transfusion with packed red blood cells to maintain the hematocrit at a physiologic level; (3) postoperative care in the intensive care unit with monitoring through an arterial line for maintenance of the MAP; (4) regular neurologic examination; and (5) limiting the use of patient-controlled analgesia to optimize patient cooperation with the examination. 20 , 120
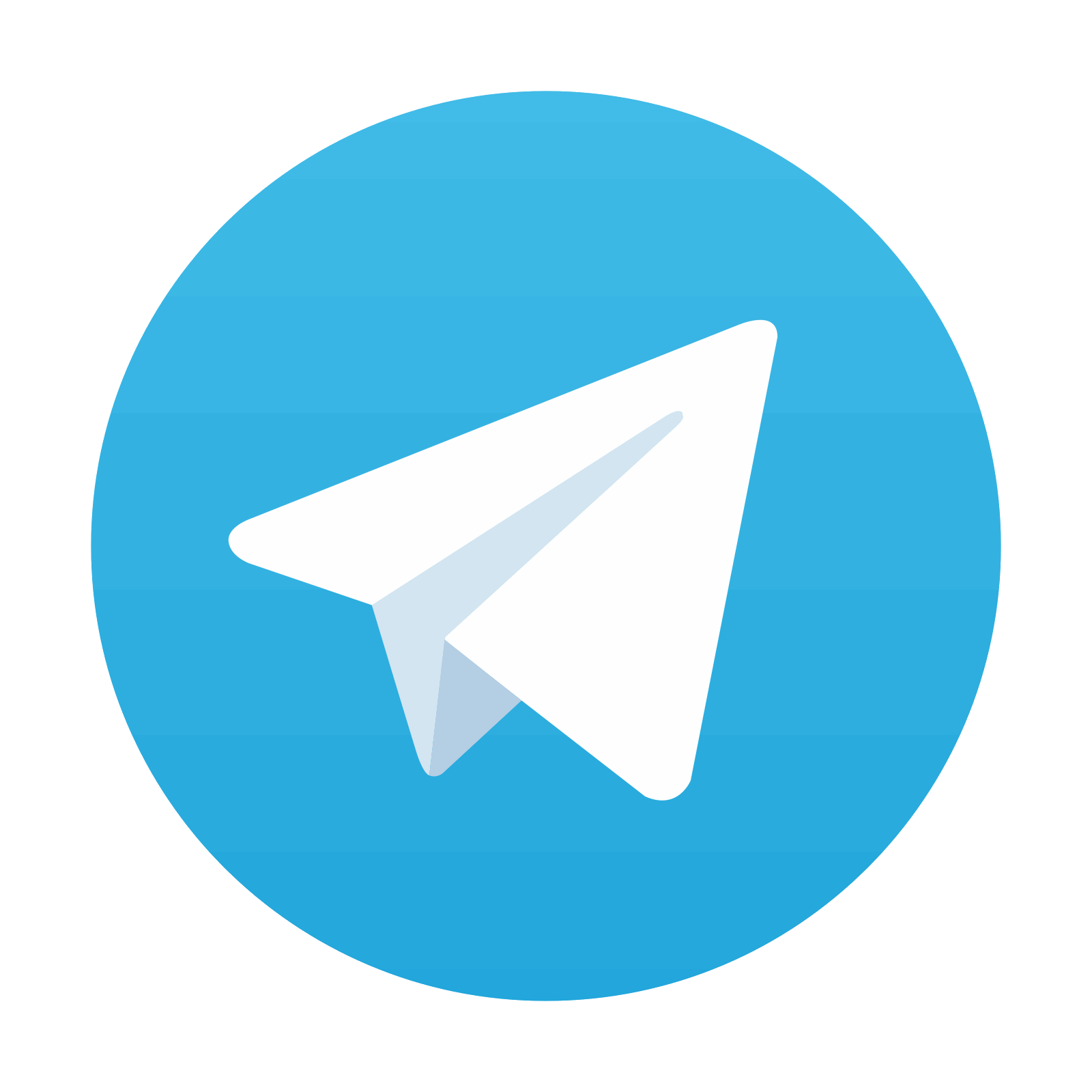
Stay updated, free articles. Join our Telegram channel

Full access? Get Clinical Tree
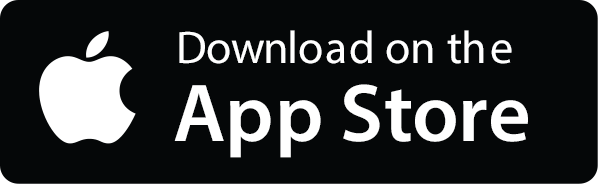
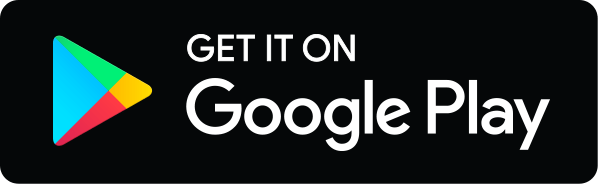
