Summary
Anterior growth modulation for progressive idiopathic scoliosis (IS) has shown efficacy in animal models and recently in its clinical application. Anterior vertebral body tethering (AVBT) has shown to be effective in this setting with promising early results; however, the determination of ideal candidates and indications for AVBT continue to evolve. Very young, immature patients (open triradiate cartilage, age < 10 years) can be at risk of overcorrection, and patients with very large curves (≥65 degrees) may experience insufficient correction. AVBT is currently gaining momentum in its clinical application by attempting to control the patient’s remaining spinal growth to prevent further progression and achieve curve correction by exploiting the Hueter–Volkmann principle. However, data regarding its true effectiveness and long-term results remain outstanding. Further research and development will be required to enhance the reliable utilization of this fusionless solution for progressive juvenile and adolescent IS.
Key words
anterior growth modulation – spinal growth modulation – vertebral body stapling – anterior vertebral body tethering – fusionless scoliosis treatment – adolescent idiopathic scoliosis treatment29 Anterior Growth Modulation
29.1 Introduction
Spinal arthrodesis has remained the gold standard in the management of progressive adolescent idiopathic scoliosis (AIS) since introduced more than a century ago; however, patients and caregivers alike have concerns about the long-term effect of spinal fusion and the resultant decrease in spinal mobility. 1 These concerns have led to the development of growth modulation techniques that may allow correction of spinal deformity without the need for an instrumented fusion. Specific concerns with arthrodesis include halting vertebral growth, loss of functional motion within the fused segments, and the potential for disk degeneration of adjacent segments due to the altered biomechanical stresses of the unfused regions. Anterior vertebral body tethering (AVBT) has been investigated for nearly two decades as a potential fusionless treatment method to manage progressive curves in skeletally immature patients by harnessing their remaining spinal growth to not only prevent further progression but also, importantly, achieve curve correction by exploiting the Hueter–Volkmann principle (Fig. 29‑1). The convex growth plates are compressed under the tension of the cordlike device, limiting convex vertebral growth, whereas the concave growth plates continue to grow, hence correcting/straightening the spine.

The Hueter–Volkmann principle is thought to be intimately related to scoliosis progression during growth. Compressive loads on the concavity decrease the physeal growth leading to vertebral wedging, which induces more compressive inhibition of growth and curve progression. Distractive forces are felt to accelerate growth. This principle has been the impetus for a number of more recent animal studies showing the efficacy of anterior spinal growth modulation. Stokes et al 2 , 3 and Mente et al 4 are credited with their classic rat tail animal model that demonstrated that the Hueter-Volkmann principle could predict vertebral body growth through mechanical modulation. Using external fixators, compression reduced rat tail vertebral growth to 68% of normal, and distraction increased growth to 114%. These authors subsequently demonstrated that asymmetric loading of rat tail vertebrae resulted in differential growth on the compression and tension sides, enabling both the production of deformity and its subsequent correction. Others have also demonstrated spine deformity creation and subsequent control of its progression in experimental animal models using a variety of mechanical implants, consisting of a shape memory alloy staple, a staple/screw device, or a flexible tether (Fig. 29‑2). 5 , 6 , 7 , 8 , 9 , 10 , 11 Braun et al 5 , 6 reported creation of a significant lordoscoliotic deformity in a goat spine using staples. Wall et al 7 showed that with a staple/screw device in a porcine model, an average 22.4-degree curve was noted at 8 weeks, and Newton et al 9 demonstrated a mean 30-degree coronal deformity in 12 months in a porcine model. Hunt et al 11 compared staples to tethers in deformity creation in their caprine model and noted that the tether construct was far superior.

Additional studies investigated the ability of anterior spinal growth modulation to correct the experimental deformity. Braun et al 12 noted tethering to be superior to staples in deformity correction in their caprine model, which was also demonstrated by Moal et al, 13 especially in larger curves. Chay et al 14 reported a more favorable three-dimensional correction of their scoliosis porcine model using an anterior-based tether compared to a control group.
The authors of these experimental studies also reported on the health of the disks in the tethered segments and found no evidence of irreversible growth cartilage or disk injury. 11 , 15 , 16 , 17 Tethering caused a shift in the nucleus pulposus toward the tether, amplified with pretensioning of the tether (Fig. 29‑3). 16 , 17 A decreased disk thickness and increased proteoglycan synthesis was also noted. A change in collagen distribution between the concave and convex sides was reported; however, there was no change in water and glycosaminoglycan content within the tethered disks. 15 The clinical implications and reversibility of these changes remain unknown.

Historically, growth modulation of skeletally immature long bones for angular deformity of the lower extremities has been quite successful in deformity correction. Epiphysiodesis using staples or a plate/screw construct has been very effective in this setting. In the setting of spinal deformity, Nachlas and Borden 18 in 1951 introduced the concept of epiphysiodesis using staples to modulate growth. Their canine model showed promise in being able to create and correct lumbar scoliosis using a staple that spanned several vertebral levels. Unfortunately, early clinical application of this concept yielded poor results in three children, and the method was abandoned. Smith et al 19 in 1954 also reported disappointing results in a cohort of patients with congenital scoliosis whose curves were stapled. The authors had a significant number of dislodgement of staples and curve progression. With advances in surgical techniques and implant technology, along with experimental studies showing the efficacy of anterior spinal growth modulation, more recently there has been a renewed interest in fusionless techniques for the treatment of scoliosis.
Betz et al 20 , 21 popularized vertebral body stapling (VBS), reporting on their initial clinical experience. Although the authors conclude that VBS can be considered for patients with progressive scoliosis, a number of authors have noted limited, if any, significant efficacy of VBS in controlling curves. Betz et al 21 found VBS to be 78% effective in thoracic curves ≤35 degrees and in 87% of thoracolumbar curves less than 35 degrees. Others have also noted VBS to have a potential role in minor curves of ≤35 degrees. 22 , 23 , 24 Most treating surgeons, however, would consider skeletally immature patients with curves ≤35 degrees to be candidates for bracing; therefore, enthusiasm for VBS has remained very limited.
Clinical proof of concept of AVBT was first reported in 2010 by Crawford and Lenke. 25 The authors highlighted the efficacy of growth modulation using an anterior-based tether in an 8-year-old boy with a 40-degree right main thoracic curve. AVBT in this case overcorrected the deformity over a 4-year period, subsequently requiring the treating surgeons to remove the tether. The case undeniably demonstrated AVBT to have the potential to correct spinal deformity using growth modulation, sparking renewed clinical interest in this concept.
29.2 Clinical Application and Potential Complications
AVBT has shown to be more effective than VBS in this setting; however, ideal candidates and indications for AVBT continue to evolve. Currently, skeletally immature patients (Risser grade 0–2, Sanders score <5, premenarchal females) with isolated thoracic curves, thoracolumbar curves, or a double major pattern (Lenke type 3 or 6) with Cobb angles between 40 and 65 degrees may be considered (Fig. 29‑4a). Preoperative flexibility of the curve is also important, and the lack of a structural proximal thoracic curve is also an important consideration for this procedure. Caution should be exercised at the extremes of skeletal maturity and curve magnitude to minimize the risks of overcorrection (Fig. 29‑4b), tether breakage (Fig. 29‑4c), adding-on, or ineffectiveness in deformity correction and/or preventing curve progression. In extremely young, immature patients (open triradiate cartilage, age < 10 years) with small curves, the risk of overcorrection has been well documented, 25 , 26 , 27 , 28 and in very large curves (≥65 degrees), some have noted tether failure and continued curve progression requiring conversion to fusion surgery. 29 The response of coronal correction may not equate to axial plane correction, and patients with a significant rib deformity should also be warned of the likelihood of residual deformity. Patients with a preoperative rib rotation less than 20 degrees may be better suited for this technique. Finally, although thoracolumbar and lumbar curves can be considered, careful evaluation of the sagittal plane should be monitored, as anterior instrumentation is known to be kyphogenic.

29.3 Surgical Technique
The procedure is performed under general anesthesia with a double-lumen tube intubation to allow for single-lung ventilation for thoracic curves being treated by a thoracoscopic approach. Thoracolumbar or lumbar curves are approached through a standard open procedure and do not require lung isolation. The patient is positioned in the lateral decubitus position with the convex side facing upward and is maintained perfectly lateral with the use of tape or a pelvic pad. Generally, four to five thoracoscopic ports are used, one to two being anterolateral working ports (along the anterior axillary line) and three posterolateral instrumentation ports (along the posterior axillary line). Using fluoroscopy prior to skin prepping and draping aids in identifying the skin incisions for the instrumentation ports (Fig. 29‑5).

The proximal anterolateral skin incision serves as the site of the first port through which a 10-mm/45-degree scope is introduced once the lung is deflated. Some surgeons use CO2 insufflation to maintain lung deflation. Care should be taken not to injure the lung parenchyma, as the trocar for this first port is introduced in a blind fashion. The second port is introduced distally along the anterior axillary line and also serves as a working port. Once the lung is fully deflated and the spine is adequately visualized, a harmonic scalpel is introduced through this port to begin dividing the parietal pleura over the spine and also ligate and divide the segmental vessels over each vertebra to be instrumented. The pleura should be reflected posterior to the rib head and anterior to the anterior longitudinal ligament to give adequate exposure of each vertebra (Fig. 29‑6).

Following the exposure, the posterolateral ports are made along the posterior axillary line to begin instrumentation. A staple is impacted within the lateral vertebral body using the rib head as a reference to gauge the anterior/posterior position of the staple so as not to encroach on the neural foramen/spinal canal. Intraoperative fluoroscopy will help confirm the adequate placement of the staple. The appropriate length and diameter screw is then introduced once the vertebral body has been tapped. Screw placement should be parallel to the endplate of the vertebra, which is done under fluoroscopic guidance. Once the safe placement of all screws is confirmed with image guidance, the polyethylene cord, which serves as the tether, is introduced, the proximal set screw is locked down, and each segment is sequentially tensioned. Using fluoroscopy, enough tension is applied to correct the disk wedging at each level sequentially to ensure that the endplates are at least parallel. This must be weighed against the possibility of screw plowing as well as the amount of initial correction the surgeon wishes to obtain on the operating room (OR) table given the preoperative curve magnitude and the skeletal age of the child. The tether is then cut at the proximal and distal ends and the thoracic cavity is thoroughly irrigated. The lung is inflated under direct visualization and the incisions are closed in a standard layered fashion. A chest tube is placed through the most distal posterior port.
Patients are encouraged to ambulate immediately postoperatively, and the chest tube is removed 2 days following surgery. Return to full activity is encouraged at 3 months following surgery.
Several technical considerations to be evaluated include the following: (1) timing of intervention with regard to the remaining growth and size of the deformity; (2) the number of levels to include in the instrumentation construct; (3) the tension (or slack) and degree of initial correction at each instrumented segment based on the segmental deformity/growth remaining; and (4) the position within the anterior vertebral body and the desired sagittal effect of the device. These are very likely critical variables to the success of AVBT procedures for a given patient. Too much tension over too many levels with too much growth for a relatively small deformity is likely to result in overcorrection and the need to release the device. Not enough growth remaining is likely to have limited benefit from the standpoint of altering the vertebral shape due to growth. Understanding that the regional differences in the deformity (more at the apex, less at the ends) must be managed together with a single surgery very likely means that the tension of the implant must be adjusted at each level to more quickly begin growth modulation at the apex and more slowly apply correcting forces at the ends. Predicting the instrumentation requirements for an ideal correction with growth will require knowledge and planning that is yet to be developed. Finite element analysis that combines growth predictions have been created and show promise in aiding the decision-making process. 30
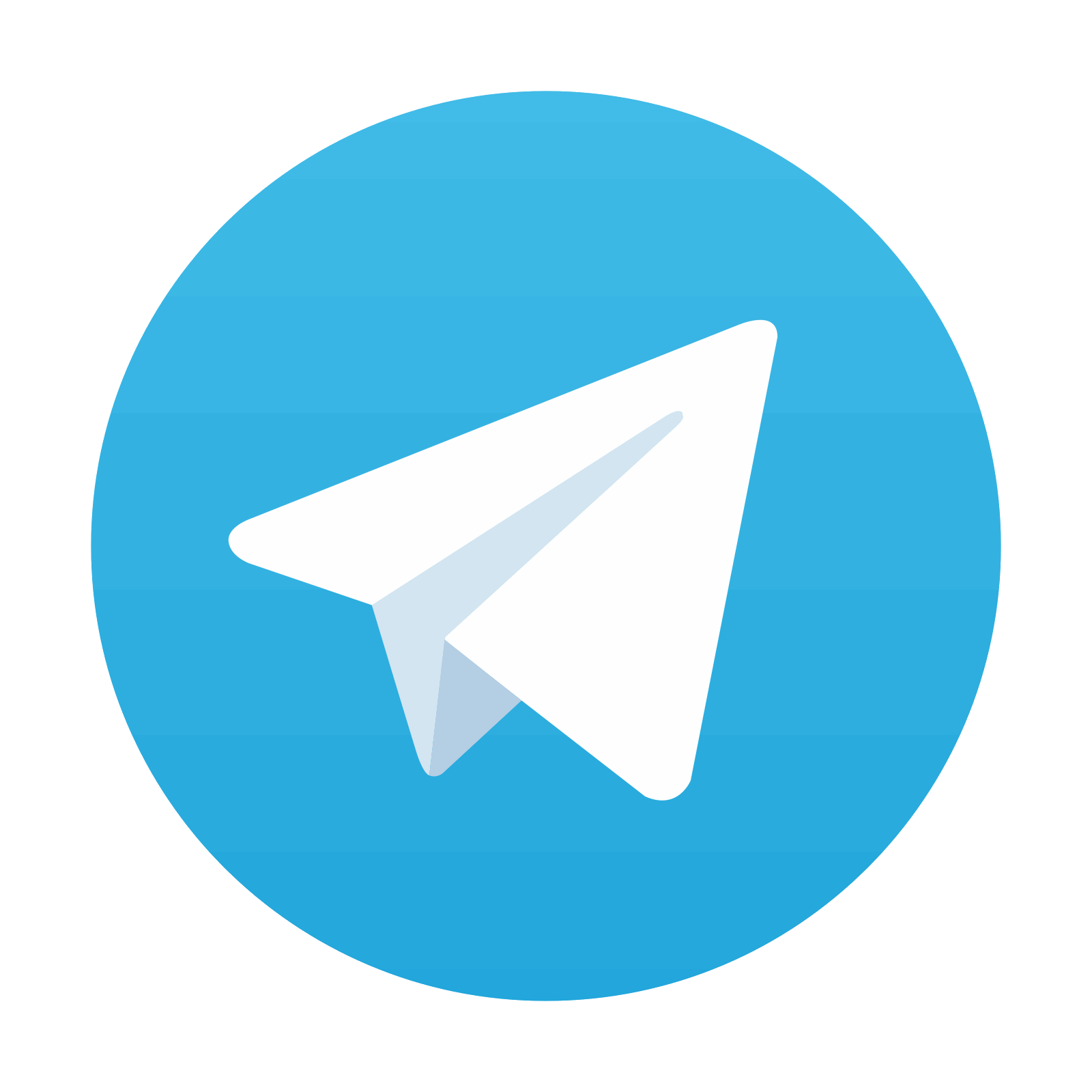
Stay updated, free articles. Join our Telegram channel

Full access? Get Clinical Tree
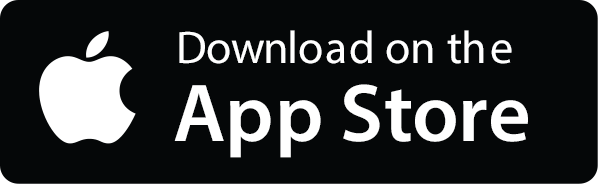
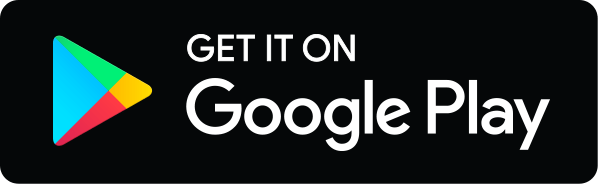