Summary
Spinal fusion is currently the gold standard surgical treatment for adolescent idiopathic scoliosis (AIS). A successful spinal fusion is predicated on several complex physiologic, biologic, and molecular processes. Historically, surgeons have modified their techniques and utilized autogenous bone graft, as well as a variety of osteobiologic agents, in an attempt to minimize the risk of pseudarthrosis and nonunion. Osteobiologic agents have demonstrated significant clinical value in the adult population; however, they come at a high economic cost. Osteobiologic agents are usually classified by their method of participation in new bone formation. The primary action of a given agent usually falls into one of the following categories: supporter (i.e., osteoconductive); recruiter (i.e., osteoinductive); or producer (i.e., osteogenic). Autogenous bone graft is unique because it assumes all three roles. Local autogenous bone graft is ideal but is sometimes considered to be limited in quantity. For many years, iliac crest bone graft offered a reasonable solution. Nevertheless, the associated morbidity of bone graft harvesting from this location limits its desirability. Fortunately, in the setting of AIS, despite varying surgical techniques and bone graft choices, the incidence of nonunion or pseudarthrosis is remarkably low. In fact, in this population, successful spinal fusion can occur without the use of bone graft. Given the high financial burden of osteobiologic agents and the paucity of evidence supporting its need in the AIS population, surgeons must be clinically and financially responsible when deciding to use these products.
Key words
spine fusion – idiopathic scoliosis – pseudarthrosis – bone graft – allograft – bone morphogenetic protein27 Osteobiologic Agents for Spinal Fusion
27.1 Introduction
A critical goal of any spine fusion surgery is to achieve a solid fusion, and surgery for AIS is no exception. Using bone graft to augment fusion rates in spine surgery has been a standard practice for decades. Autogenous bone, such as iliac crest bone graft, has long been considered the gold standard for optimizing fusion rates. A variety of allogenic options exist as well, both from biologic and synthetic sources. This chapter will review the biology of bony fusion, the differences between the various bone-grafting materials available, and the latest evidence describing their use and effectiveness. Although the problem of pseudarthrosis in adult spine surgery has been a significant issue, it is important to note that reported rates of pseudarthrosis in instrumented idiopathic scoliosis surgery have been very low 1 , 2 regardless of the type of bone graft, if any, used. Thus, care must be taken when using data from adult spinal fusion studies to guide decision-making about patients with adolescent idiopathic scoliosis (AIS). We will highlight literature directly pertaining to AIS patients when available.
27.2 The Biology of Spinal Fusion
Traditionally, obtaining solid bony fusions in adult spine surgery has been challenging, with high rates of nonunion or pseudarthrosis (we will use the terms interchangeably in this chapter). A lateral arthrodesis of lumbar intertransverse processes, one of the most common adult spinal fusion procedures, fails to achieve a solid bony union in 10 to 40% of patients with single-level uninstrumented fusions. Failure rates are even higher when multilevel fusions are attempted. 3
The success of any fusion operation depends on a complex interplay of numerous physiologic, biologic, and molecular events. Perhaps the increased utilization of more rigid segmental internal fixation systems with pedicle screws and stiffer rods over the past 10 to 15 years has decreased the number of nonunions; however, it has not eliminated the problem, with nonunions occurring in 10 to 17% of adult patients, who undergo instrumented spinal fusions for deformity or degenerative disease. 4 , 5 Studying the biologic sequence of events in spinal fusion provides key insights into devising biologic products and bone graft substitutes to enhance fusion rates. 3
The biology of spinal fusion is a multifactorial process, making it difficult to study in the clinical setting. Early animal models used to investigate the biologic events in spinal fusion had fusion rates approaching 100%, a figure much higher than is seen in most adult human clinical studies. Boden et al developed a successful rabbit model of intertransverse process arthrodesis that developed nonunions at a rate comparable with that reported in humans who had fusion without internal fixation. 6
The rabbit model of lumbar intertransverse process arthrodesis has been well characterized with the use of autogenous iliac crest as the graft material. Mechanically solid fusions, in the model, generally occur by 4 to 6 weeks, with a 30 to 40% overall nonunion rate. Radiographic analysis has shown bone graft remodeling starting at approximately 10 to 12 weeks, but as in humans, radiographs were accurate in assessing success or failure to attain solid fusion only 70% of the time. Control animals have demonstrated that surgical exposure alone does not automatically result in spinal fusion, as has also been seen in other species. 6 Vascular injection studies indicated that the primary blood supply to the fusion mass originated from the decorticated transverse processes. The failure to achieve spinal fusion in the absence of decortication emphasizes the importance of careful fusion bed preparation of the posterolateral elements of the spine for successful fusion. Proper preparation of the fusion bed is required to provide critical bone marrow, vascularization, and cellular elements to the fusion mass. 6
Three distinct and reproducible temporal phases of healing in spinal fusion have been histologically identified: an inflammatory phase, an endochondral phase, and a remodeling phase. 7 Histologic analysis has indicated that fusion mass maturation begins peripherally and progresses centrally. The maturation of a spinal fusion was most advanced at the ends of the fusion mass, near the transverse processes (outer zone). A similar histologic progression occurred in the central zone but was delayed relative to the series of events in the outer zones. A similar lag has been noted in osteoblast-related gene expression, with the peak expression of all genes occurring 1 to 2 weeks later in the central zone than in the two outer zones. This is consistent with the peripheral-to-central healing pattern observed histologically in fusions done with autogenous bone graft material. This central lag effect, with a transient cartilaginous area, was hypothesized as the reason for many nonunions in the central zones of fusion masses.
Variations were also seen in the temporal and spatial expression of the messenger ribonucleic acid (mRNA) for bone morphogenetic protein (BMP). In the peripheral zones, expression of the mRNA for BMP-2 was increased during weeks 2 through 6, with peak expression at weeks 3 and 4. BMP-6 in the outer zones had a first peak on day 2 and a second peak during week 5. BMP-6 in the central zone showed an initial peak on day 2 but did not demonstrate a later peak. This lower level of expression of BMP-6 observed in the central zone of the fusion mass may be correlated with the delayed timing and smaller amount of bone formation in the central zone. 7
27.3 Osteobiologic Products and Spinal Fusion
Over half a million bone graft procedures are performed in the United States annually. Of these, the vast majority are spinal fusions, potentially representing up to $2.5 billion spent per year on agents for enhancing bone repair and bone graft substitutes (Table 27‑1). 8 , 9 Because of the distinct biologic conditions and biomechanical forces specific to the anterior spinal column and the posterior elements, it is likely that the efficacy of each bone graft material in promoting successful fusion will also depend on the particular clinical application for which it is being used (e.g., interbody fusion vs. posterolateral fusion; Table 27‑2). 10 , 11 , 12
The processes of bone regeneration and formation during spinal fusion require three critical elements: (1) osteogenic cells that have the capacity to make new bone; (2) osteoinductive factors (i.e., growth factors and cytokines) that promote the differentiation of stem cells into an osteoblastic phenotype; and (3) an osteoconductive scaffold that facilitates neovascularization and supports the ingrowth of bone. Thus, any bone-grafting material needs to support at least one of these three elements. Bone grafts that promote stem cell differentiation are considered osteoinductive, whereas those that provide a scaffold are considered osteoconductive. Some grafts can provide more than one of these elements and, in fact, autogenous bone has all of these essential properties and is considered the “gold standard” graft material for spinal fusion.
27.3.1 Autogenous Bone Graft
Autogenous bone graft for use in spinal fusion surgery has traditionally been taken from the iliac crest, and this is the standard against which all other techniques are compared. 13 , 14 As mentioned earlier, bone from the ilium contains osteogenic cells as well as osteoinductive factors and provides a scaffolding for vascular and bony ingrowth. It also can have some structural integrity depending on how it is harvested. Although it is an excellent option on the surface, there are some significant problems with iliac crest bone graft. Procurement of iliac crest bone graft necessitates a separate fascial, if not skin, incision, and thus involves additional surgical trauma. Besides increasing the operative time and blood loss, the harvesting of bone graft material from a separate site is also associated with considerable donor site morbidity. Nearly 30% of patients who undergo such procedures experience some postoperative complication including chronic pain, infection, hematoma, nerve or vascular injury, fracture, abdominal herniation, and pelvic instability. 15 , 16 , 17 The amount of bone available for grafting may also be insufficient in some children. 18 , 19 , 20 Given these real problems with iliac crest bone grafts, along with the documented low pseudarthrosis rates, most surgeons eschew this source of bone graft for AIS in this day and age.
In response, there has been a spate of recent studies reporting on the use of local bone graft only; this is bone harvested at the fusion site through the existing incision (i.e., spinous process, facet joints, portions of the transverse process, and bone from posterior column osteotomies). 14 , 21 , 22 For completeness, it should be noted that there are other potential sources of autogenous bone graft that can be harvested through the standard midline incision including rib (from thoracoplasty) and vertebral body (from vertebrectomy), although spinal fusions for AIS rarely include these procedures anymore. 23 Although thoracoplasty has been shown to be an effective part of the deformity correction in AIS with reasonable safety, 24 , 25 other authors have raised concerns about its adverse effects on chest wall and respiratory function. 26 In summary, the literature clearly concludes that there is no benefit to iliac crest bone graft or other nonautogenous bone graft over local autogenous bone graft in AIS. 14 , 21 , 22 , 27
27.3.2 Allogenic Bone Graft
In settings where autograft is inadequate in volume or inaccessible for other reasons (e.g., revision surgery), external sources of bone graft (allogenic bone graft) need to be considered to enhance fusion rates. There are three general descriptions for nonautogenous, or allogenic, bone grafts:
Bone graft extenders are substances that add bulk to a given amount of autogenous bone that is to be used over a greater surface area with the same rate of success. These are generally osteoconductive grafts.
Bone graft enhancers are substances that increase the healing potential of autogenous bone grafts and may lessen the amount of autogenous graft required. These are the osteoinductive grafts.
Bone graft substitutes are substances that can entirely replace autogenous bone graft material with the same or better rate of successful fusion. 28
27.3.3 Cadaveric Allograft
Allograft bone obtained from cadaveric sources has been the most widely used substitute for autogenous bone graft material. 29 , 30 , 31 Allografts are osteoconductive, with minimal or no osteoinductive potential, primarily because the donor’s cells are intentionally eradicated during tissue processing. Allografts are prepared by either freezing or lyophilization (i.e., freeze-drying) to decrease their antigenicity and permit their storage for extended periods. Frozen allografts may be kept for up to 1 year at minus 20°C without a change in their structural properties. Lyophilized allografts are dehydrated and vacuum packed, which allows their storage at room temperature. Freeze drying reduces the immunogenicity of allografts more than does freezing, but upon rehydration and reconstitution, freeze-dried grafts may lose up to 50% of their mechanical strength. Allografts may also be treated with ethylene oxide or radiation, although these methods may further compromise their material properties. 29
A common patient concern with the use of cadaveric allografts is the possible spread of infectious diseases, such as hepatitis C virus (HCV) and human immunodeficiency virus (HIV). To date, nine cases of transmission of HIV in allograft bone have been documented, eight of which occurred before 1986 and one in 1996. There have been 10 reported cases of HCV transmission, none since 2000. 32 The combination of meticulous donor screening and tissue processing has reduced the risk of infection from allograft bone to less than one per million transplants. 33
Cortical allografts offer substantial structural stability and are well suited for intervertebral body arthrodesis, although this type of fusion is rarely indicated in AIS. Threaded cylinders (of cortical allograft) may serve as a source of bone graft material while stabilizing the spinal column. Hollow centers allow the insertion of osteoinductive materials (such as autograft) to aid anterior fusions. Fusion with these techniques occurs slowly, by means of periosteal new bone formation around the allograft. Cortical allografts never fully incorporate at their site of engrafting and remain a mixture of avascular (dead) and viable bone. On the other hand, corticocancellous allograft material (commonly called “croutons”) initially imparts very little mechanical support, but because its relatively large surface area is integrated more rapidly and fully than a purely cortical bone graft.
Both autogenous bone and allogenic bone are incorporated into the fusion mass according to a well-defined cascade of biologic events, consisting of hemorrhage, inflammation, and vascular invasion, culminating in the replacement of graft material with new bone. With allogenic bone, this remodeling process occurs more slowly, and there is greater resorption of the graft than with autograft bone. This may manifest itself in the intervertebral body milieu by cortical lucencies that indicate resorption and vascular invasion. Genetic incompatibility of the donor and recipient has been found to be associated with increased resorption of allograft bone and histologic evidence of rejection; however, routine screening for genetic compatibility may be impractical, 34 and this has never been shown to be a clinical problem.
The differing mechanical and biologic properties of the various types of allografts make each type suitable for different applications. Predominantly cortical allografts, such as femoral rings or fibular struts, should be used when there is a need for structural support under compression (e.g., intervertebral body applications). Allografts of cancellous bone, such as cancellous bone chips, are best suited in areas that require little mechanical strength, but there is a desire for more rapid bone incorporation, remodeling, and revascularization.
The adult literature that addresses the impact of allogenic bone graft on fusion rates is often hard to interpret due to evolving (e.g., more rigid) surgical implants and variable grafting techniques. Additionally, the relevance of these studies to patients with AIS is not clear. In studies specific to AIS, few seem to be truly powered to find a difference where one exists, as the published pseudarthrosis rates are so low. Dodd et al 35 reported a 100% fusion rate in adolescent patients with idiopathic scoliosis who had implants of femoral head allograft and local autograft bone. This study followed only 40 patients, and fusion was determined by radiographic findings at 6 months. Betz et al 36 compared the clinical results of posterior spinal fusion (PSF) with allogenic bone augmentation and those without grafting in patients with AIS. Ninety-one patients with AIS undergoing PSF with instrumentation were randomized into two treatment groups of whom 76 patients had more than 2-years of follow-up and were included in the review. There were 37 patients randomized to corticocancellous bone allografts and 39 underwent the same procedure without any bone graft at all (no autograft or allograft). There was only one pseudarthrosis in the study period that happened to occur in the allograft group; there were no cases of pseudarthrosis in the no-bone-graft group. A more recent prospective but nonrandomized study similarly showed no difference in fusion rates between patients with AIS getting allograft to augment local autograft and those getting local autograft alone. 22
In conclusion, there seems to be good evidence that nonstructural allogenic bone graft (bone chips or “croutons”) can function successfully as an osteoconductive bone graft extender. However, it should also be noted that there is evidence that in the AIS population, fusion can occur in the complete absence of bone graft. 36
27.3.4 Ceramics
Ceramics are synthetic bone graft substitutes that consist of an inorganic material that acts as scaffolding for growing bone. Ceramics are commonly made of hydroxyapatite (HA), tricalcium phosphate (TCP), or a combination of these two materials, such as coralline HA, which is derived from sea coral. Ceramics are exclusively osteoconductive and contain a pore structure that allows the ingrowth of new bone. 37 Ceramics are nontoxic, nonimmunogenic, easy to sterilize, and available in virtually unlimited supply. Their main disadvantage is that they are brittle and have little shear strength or fracture resistance. 38 However, these are not characteristics typically needed for AIS surgery. Ceramics are commonly used in conjunction with internal fixation, owing to their poorer mechanical strength; they must be protected from loading until they are incorporated into the host bone.
There are multiple studies examining the use of ceramics in patients with AIS. Although some of these studies are small and uncontrolled, 39 , 40 there are a few controlled trials comparing ceramics to either autologous iliac crest 41 , 42 or cancellous croutons and local autogenous graft. 43 Two randomized trials compared ceramics to iliac crest autograft. 42 , 44 Interestingly, there were no cases of pseudarthrosis in any of these reports in either the ceramic or the control group.
It is important to remember that ceramics do not exhibit osteogenic or osteoinductive properties; they are osteoconductive and depend on the local environment for osteoprogenitor cells and signals. The existing literature does not support any benefit of using these materials over local autograft alone. However, ceramic scaffolds have been shown to facilitate cellular adhesion, support vascular ingrowth, and promote new bone formation when used with autogenous bone graft or bone marrow aspirate, and there is good evidence in the literature that they can function as bone graft extenders in cases where that is deemed necessary. An additional benefit of ceramics as a component of composite graft substitutes is that they act as viscous matrices that limit the diffusion of osteogenic cells and signals away from the fusion site. 45
27.3.5 Demineralized Bone Matrix
Demineralized bone matrices (DBMs) are generated by the acid extraction of processed allograft bone, giving rise to a demineralized matrix consisting of type I collagen and noncollagenous proteins as well as numerous signaling cytokines. Removal of the mineral phase of the bone releases these biologically active cytokines, making them more accessible to osteogenic or inflammatory cells. BMPs constitute less than 0.1% by weight of all bone proteins found in DBMs. However, these growth factors are essential to osteoinduction and ultimately to bone formation; thus, DBMs can be considered to have osteoinductive properties. 46
After being extracted from bone, DBM exists as a particulate powder. Its effectiveness in grafting depends on its localization and retention at the graft site. Human DBM is often combined with other components (carriers) intended to make DBM easier to handle by turning it into a putty or paste. These carriers must be biocompatible with bone, not reduce the osteoconductivity of DBM, maintain graft containment during wound irrigation and closure, and maintain graft localization until the graft site is stabilized.
To be used as a bone graft, DBM must be combined with a carrier, and this carrier usually represents the majority of the complex (~85% carrier and 15% DBM). There exist a variety of DBM carriers including glycerol, gelatin, calcium sulfate, lecithin, and hyaluronic acid. Some of these carriers have theoretical risks: ultrahigh doses of glycerol containing DBMs (Grafton) have proven toxic when administered to athymic rats, eventually leading to death from renal failure in a dose-dependent manner. 47 However, there have been no reported cases of glycerol toxicity related to the implantation of these DBM products in humans. The osteoinductive capability of commercially available DBMs varies from one product to another and may even vary from lot to lot of a specific product. This variability is thought to come from differences in BMP content, as noted by Bae et al. 48 However, the absolute concentration of BMP in a particular DBM preparation may not correlate with its clinical efficacy as different methods of processing and sterilization, as well as the carrier used in the product, can affect its osteoinductivity.
Cost-effective 49 and readily available from human tissue banks, DBM formulations are attractive graft enhancers and extenders. The demineralization process eradicates the antigenic epitopes in bone, making DBM considerably less immunogenic than mineralized bone allograft.
As with ceramics, DBMs appear to be most effective in fusion environments that allow unimpeded angiogenesis and a steady passage of osteoprogenitor cells. Autograft or bone marrow aspirates may be added to DBMs to increase the osteoinductive index and possibly osteogenic potential of the bone graft. However, in preclinical models, DBMs have been found to promote successful arthrodesis of the spine when used alone or in conjunction with autograft, bone marrow, or ceramics. 50 , 51 , 52 These results may be a direct indication of the species-specific nature of DBMs for spinal fusion.
The literature on the efficacy of DBM in human spinal fusion is based mainly on adult cervical 53 and lumbar fusions 54 , 55 for degenerative spinal disorders. The initial studies were not overwhelmingly positive—rates of pseudarthrosis were higher in DBM groups compared to autograft (46 vs. 26% 53 ), although the differences were not statistically significant. Most lumbar studies showed more equivalence between autograft and DBM plus autograft with no differences at 2 years, 54 , 55 although one study using coralline HA found that augmenting with DBM actually decreased fusion rates. 56
Price et al 57 conducted the only study in AIS patients looking at posterior fusions augmented by bone graft. They found no difference in fusion rates with iliac crest autograft and DBM mixed with bone marrow aspirate.
In summary, current data suggest that DBMs have limited efficacy as graft substitutes but may be indicated for use as bone graft extenders and enhancers when used in combination with autograft bone or bone marrow, especially in situations where there is a limited amount of available autograft bone.
The rest of this chapter will discuss existing products that have been described and used in surgery for adult degenerative and deformity surgery. They are included for completeness, but to date, there is no literature supporting their use in AIS.
27.3.6 Osteoinductive Proteins
The most extensively studied osteopromotive factors in the processes of osteoinduction and bone formation are the BMPs, which have been shown to initiate and encourage the osteoblastic differentiation of pluripotential mesenchymal stem cells in vitro (Table 27‑3). They are also the only known protein-signaling molecules capable of inducing ectopic bone production in vivo. BMPs are soluble, low-molecular-weight glycoproteins that share extensive homology with transforming growth factor-β (TGF-β). By binding to specific receptors on the surfaces of receptive mesenchymal stem cells, these extracellular factors activate intracellular signal transduction pathways responsible for osteoblastic differentiation and function.
As with DBMs, recombinant human BMPs (rhBMPs) need to be combined with an inert carrier that serves to restrict their elution, keeping them in the fusion bed without having an adverse effect on the osteoinductive properties of the particular protein factor being used. The carrier may also act as an osteoconductive scaffold that supports new bone formation by promoting cellular adhesion and angiogenesis. Autogenous bone graft, DBMs, collagen, ceramics, and polylactic acid have all been used to deliver rhBMPs, but the ideal carrier for these recombinant proteins remains elusive. There will be site-specific mechanical and biologic demands on the specific carrier depending on the indication for its use; for example, an anterior spinal fusion will require more structural stability as compared to a posterolateral intertransverse fusion.
The genes encoding the BMPs have been sequenced and subsequently cloned, allowing the mass production of a single specific BMP including BMP-2 and BMP-7. 58 , 59 , 60 rhBMP-2 has been approved by the Food and Drug Administration (FDA) specifically for applications in anterior spinal fusion as well as for open tibial fractures. rhBMP-7 was approved in the early 2000s by the FDA under the Humanitarian Device Exemption (HDE) program as an alternative to autograft bone in cases of recalcitrant long-bone nonunion in which the use of autograft bone is unfeasible and alternative treatments have failed. However, failing to get an expanded approval from the FDA in 2009 ultimately led to the discontinuation of rhBMP-7, which is no longer marketed in the United States.
Unfortunately, since the previous edition of this textbook, there has been some significant controversy about the ethics of studies done demonstrating the effectiveness of BMPs, and the stated conclusions of much of the literature have been questioned. 61 It seems that many complications and adverse effects were under-reported and are now being captured through the Yale University Open Data Access (YODA). 61 Along with the increased reports of harm, recent studies have demonstrated that these products are still inferior to iliac crest bone graft in lumbar surgery. 62
Although BMPs have been used anecdotally in AIS, there is no approved indication for use in AIS. A recent review of an administrative database found no benefit of BMPs in the AIS population. 63 Based on the potential for harm and the lack of any evidence of increased effectiveness in this population, authors believe there is no routine role for rhBMP-2 or rhBMP-7 in the AIS population.
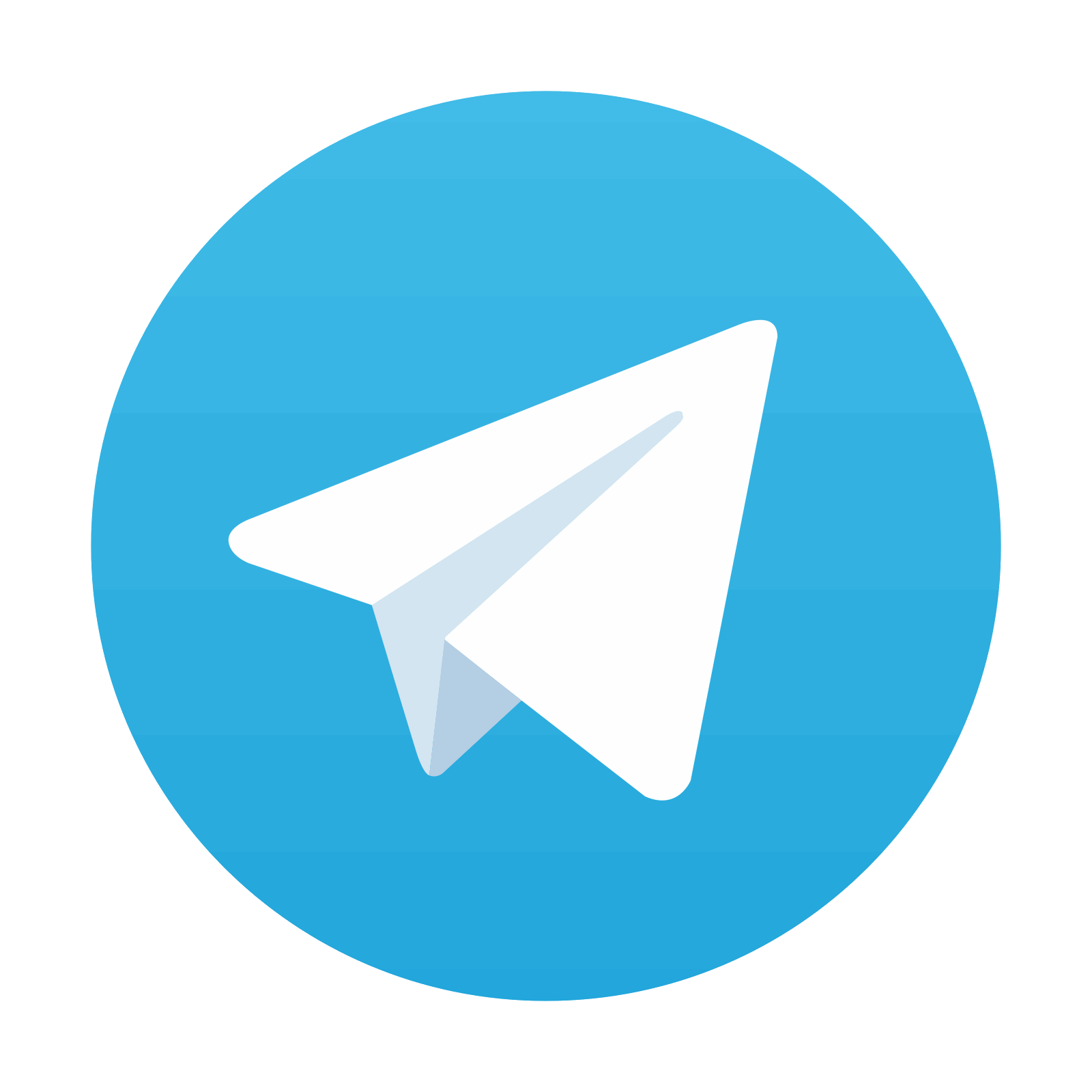
Stay updated, free articles. Join our Telegram channel

Full access? Get Clinical Tree
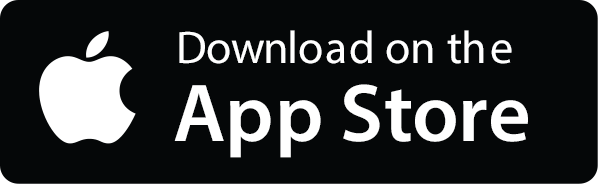
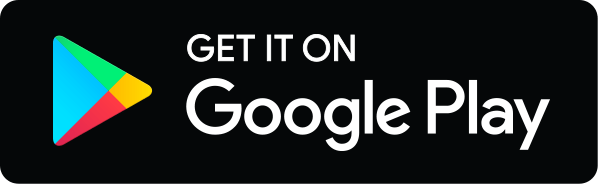
