2 Morphometry and Microarchitecture of the Human Scaphoid
Among the bones of the wrist, fractures most commonly occur in the scaphoid, which accounts for almost 70% of all carpal fractures.1 Experimental studies in cadavers have demonstrated that the scaphoid will fracture at its waist when the palm is loaded with the wrist in hyperextension.2 Another proposed mechanism for waist fractures is the transmission of a palmarly directed shear force across the distal scaphoid when force is transmitted from the index metacarpal via the trapezoid and trapezium, as happens with punching.3 The waist may be more prone to fracture because it is the fulcrum, whereas the distal and proximal portions remain firmly attached to the bones of the respective carpal rows.
The higher incidence of fractures at the waist may also be related to the morphology and structural properties of the bone, as will be detailed in this chapter.
Several different implants are commercially available for scaphoid fixation. Knowing the internal architecture of the scaphoid helps in understanding fracture patterns and the effects of fixation, and this may influence implant choice. Additionally, knowledge of the scaphoid dimensions is helpful for choosing the appropriate implant from the large variety of screw sizes that are commercially available. This chapter details the morphometric and microarchitectural anatomy of the human scaphoid and is based on data from human cadaver studies.
▪ Microarchitecture
Because of the scaphoid’s small size and its position at 45 degrees to both the sagittal and coronal planes, it is difficult to study the bone architecture with conventional imaging techniques. Microcomputed tomography (μCT) is a relatively new engineering tool that employs CT at microscopic resolutions to allow a detailed and accurate three-dimensional (3-D) study of a specimen in its native state without prior sectioning or preparation.4 Unlike conventional clinical CT, which sections bone at 1- or 2-mm slices, μCT sections are performed at 30 to 40 μm, creating images that are extremely detailed. The machines are generally desktop-based, and the large amounts of radiation involved make this technology suitable only for experimental studies.
We studied five cadaveric scaphoid specimens at the University of Arkansas Center for Orthopedic Research (study in publication) with a Micro CT 40 scanner and a slice thickness of 36 μm. After each specimen was scanned, accurate 3-D models of the bone were created ( Fig. 2.1 ). It was then possible to virtually section the 3-D image in any desired plane to observe the bone structure as well as to accurately quantify the trabecular content in different areas of the scaphoid.
Morphological assessment of the images revealed that the scaphoid consists of a narrow rim of cortex enclosing dense trabecular bone ( Fig. 2.2 ). The trabecular pattern is consistent among different scaphoid specimens and varies by the region of the scaphoid. At the proximal and distal poles, the trabeculae largely run across the shortest distance and are closely packed. At the waist, arising from the vascular ridge on the dorsum of the scaphoid, the trabeculae radiate obliquely to the proximal and distal poles. It is interesting to note that the trabecular pattern closely follows the vascular distribution, as has been previously reported ( Fig. 2.3 )5 Most of the scaphoid blood supply enters from the dorsal ridge and then branches to reach the proximal and distal poles. The trabeculae are likely laid along the minute vascular channels, which appear to rely on the trabeculae for support. By virtue of their orientation and distribution, the trabeculae are more spaced out and less dense at the waist.



The bone is densest at the proximal pole, where the trabecule are the thickest, at around 0.20 mm each, and are more tightly packed, with 1.81 trabeculae per millimeter. In contrast, they are thinnest at the waist, with an average thickness of 0.18 mm, and are more sparsely distributed, at 1.58 trabeculae per millimeter.
These data suggest that the waist is structurally the weakest part of the bone, which may be one of the factors accounting for the high incidence of fractures that involve the scaphoid waist.
Effect of Implant Placement
There are several commercially available screw implant designs for scaphoid fracture fixation. The screws are headless and generate compression by means of either a fully threaded variable pitch design or two threaded ends connected by a smooth shaft. Selection of the implant is usually based on the difficulty of instrumentation and surgeon preference. It is very useful to understand the “footprint” of an implant within the scaphoid because the fixation relies on its purchase in trabecular bone. We studied the effect of implant placement in the intact scaphoid with μCT for two different implant designs: a fully threaded, variable-pitch screw (Acutrak, Acumed LLC, Beaverton, OR) and a screw threaded at either end of a smooth narrower shaft (Herbert, Zimmer Inc., Warsaw, IN).
Images obtained after screw insertion demonstrate that reaming the scaphoid produces a track within the bone by impacting trabeculae peripherally. A fully threaded screw fills the reamed channel and maintains bone contact along its length ( Fig. 2.4 ). Stability is thus achieved by radial contact between the screw and the trabeculae throughout its length and by compression between the fractured fragments. An implant that is threaded only at the ends, however, fails to contact the bone in the unthreaded portion, leaving a void at the scaphoid waist ( Fig. 2.5 ). In a fractured bone, this screw design will maintain reduction as long as the fracture is stable under compression. Because there is no purchase in the central portion, fixation with a partially threaded implant may be compromised in comminuted fractures or after bone resorption with healing.
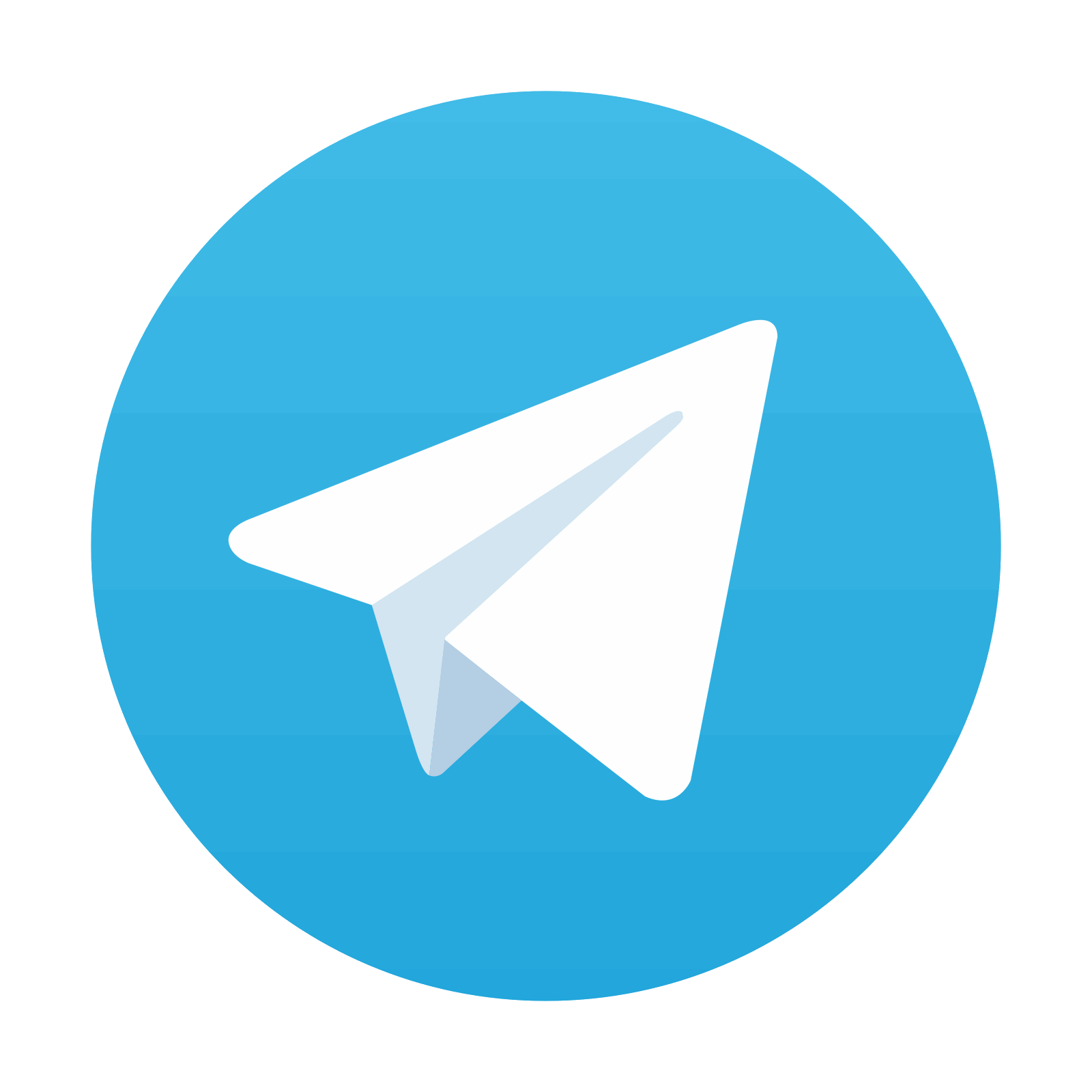
Stay updated, free articles. Join our Telegram channel

Full access? Get Clinical Tree
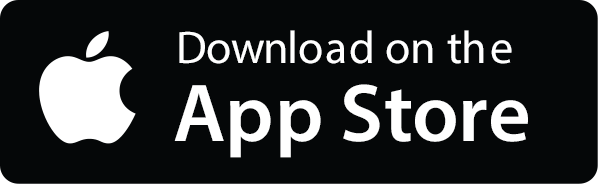
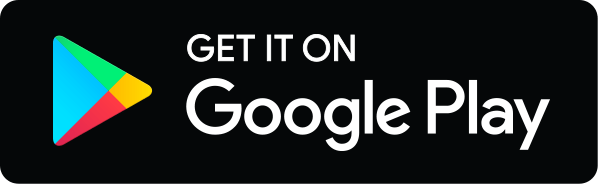
