However, 75% of 233 spontaneous abortions studied were noted to have an abnormal karyotype, with 18% having a morphologic defect and normal karyotype (16). At present, only a small percentage of these are known to be caused by defined genetic events. In most cases, the cause of the congenital difference is unknown, but expanding genetic information provides optimism for increased knowledge in the near future.
![]() FIGURE 22-1. Data sheet for prospective analysis of hemiplegic function used at Boston Children’s Hospital. |
TABLE 22-1 House Classification of Upper Extremity and Hand Function for Patients with Cerebral Palsy | ||||||||||||||||||||||||||||||
---|---|---|---|---|---|---|---|---|---|---|---|---|---|---|---|---|---|---|---|---|---|---|---|---|---|---|---|---|---|---|
|
The flexor carpi ulnaris should have good motor power.
There should be good passive dorsiflexion of the wrist, extension of the fingers, and supination of the forearm.
The patient should be able to extend the fingers actively, with the wrist held in neutral position.
The patient should have good voluntary control over placement of the arm.
There should be adequate sensory function in the hand.
The patient should have reasonable intellect.
The patient should be old enough to comply with the postoperative therapy program.
No movement disorder, such as athetosis, should be present.
![]() FIGURE 22-8. In type III deformities, it may be necessary to stabilize the metacarpophalangeal joint of the thumb. This can be done by arthrodesis. In the growing child, the stabilization can be accomplished by denuding the cartilage from the joint surface and by fixing the joint with an intramedullary pin, thereby sparing the growth of the physis. Another method that preserves more of the function of the thumb, however, is described by Filler and colleagues (Tabin C. The initiation of the limb bud: growth factors, Hox genes, and retinoids. Cell 1995;80:671-674.). Through a V-shaped incision over the volar aspect of the metacarpophalangeal joint, as described for release of trigger thumb, the sheath of the flexor pollicis longus is partially excised to expose the tendon. As with release of trigger thumb, it is important to identify and retract the neurovascular bundles carefully, particularly the radial digital nerve that lies just beneath the skin and crosses the operative site. This exposes the volar plate or capsule. Its proximal insertion (A) is incised and freed. Both sides are then incised just outside of the sesamoid bones so that only the distal attachment remains. The joint is flexed 30 to 35 degrees and transfixed with a small Kirschner wire. The capsule is advanced proximally until it is taut. At this new point of insertion, a small groove is cut into the cortical bone, and a small drill hole is made from this groove to the dorsal surface of the metacarpal. A pull-out wire or a strong absorbable suture is passed through this hole and tied over a button on the dorsum of the thumb (B) to secure the insertion of the volar plate into this groove. In type IV deformity, it is necessary to lengthen the flexor pollicis longus. AZ lengthening is easily accomplished proximal to the wrist (see Fig. 22-7C). Numerous muscles can be used to augment the functions of the abductor pollicis longus, the extensor pollicis brevis, or the extensor pollicis longus. The palmaris longus, the brachioradialis, and the flexor carpi radialis muscles are commonly used. |
by natural history has been best defined by the spontaneous rate of recovery of muscle strength in the first 3 to 6 months of infancy. Gilbert and Tassin (83) described the recovery of antigravity biceps function in infancy as a predictor of the outcome of spontaneous recovery. This finding was confirmed in a similar study by Waters (82). These studies demonstrated that infants who did not recover biceps function by 3 months of life were not normal after 2 years of age. Gilbert et al. recommend microsurgical reconstruction of the plexus in the first 3 to 6 months of life for infants who fail to recover biceps function (83, 84 and 85)(61). Michelow et al. (86) noted that return of biceps function alone had a 12% error rate in predicting outcome, as defined by long-term antigravity muscle strength. By using elbow flexion, elbow extension, wrist extension, finger extension, and thumb extension (the Toronto scale), their error rate for predicting outcome decreased to 5%. In this system, each muscle group is scored as 0 (no motion), 1 (motion present but limited), or 2 (normal motion), for a maximum score of 12. A score of <3.5 predicted a poor long-term outcome without microsurgery. In all studies, the presence of Horner syndrome, total plexus involvement, and failure of return of function by 3 to 6 months of life portend a poor long-term outcome.
myelography, combined computed tomography (CT) scan and myelography, and magnetic resonance imaging (MRI). Kawai et al. (89) compared the results of all three techniques with operative findings. MRI and combined myelography and CT scan were more reliable than myelography alone. The presence of large diverticulae and meningoceles was indicative of root avulsion. Small diverticulae were diagnostic only 60% of the time. Electrodiagnostic studies, with electromyography and nerve conduction studies, are diagnostic of avulsion if there is no reinnervation after 3 months of age. However, the presence of reinnervation does not indicate the long-term quality of muscle recovery.
should have surgery between 3 and 6 months of life. However, the controversy regarding the best timing for microsurgery, whether it should be done at 3, 4, 5, 6, or even 9 months, is still unresolved. This creates difficulties for parents who are trying to do what is best for their infants. There is an ongoing prospective study sponsored by the Pediatric Orthopaedic Society of North America (POSNA) that hopes to resolve this issue.
TABLE 22-2 Computed Tomography/Magnetic Resonance Imaging (CT/MRI) Classification of Glenohumeral Deformity in Chronic Brachial Plexus Birth Palsies | ||||||||||||||||||
---|---|---|---|---|---|---|---|---|---|---|---|---|---|---|---|---|---|---|
|
techniques, the forearm is positioned in approximately 20 to 30 degrees of pronation. Others have described corrective osteotomies of the radius and ulna with plate fixation with good reported correction (109).
PIP joints. All of this will limit hand function in these children (117).
hygiene. In addition, the pectoralis major muscle crosses the shoulder and may lose strength in trying to move both the shoulder and the elbow.
of motion and function and associated regional anatomic anomalies. Surgery is indicated in children with severe aesthetic and functional limitations. Surgery does not correct the scapular hypoplasia but is indicated for improving shoulder motion by restoring more normal positioning of the scapula and the glenoid. This often consists of excising any omovertebral connections and surgically derotating and caudally relocating the scapula. Most of the procedures that are described include extraperiosteal resection of the superior pole of the scapula (130, 132). Subperiosteal resection is associated with a high rate of recurrence (133, 134). In addition to functional indications for surgery, most patients and families welcome the improvement in the appearance of the neck line.
![]() FIGURE 22-23. Various forms of fixation have been used, all more or less with success. Grogan and colleagues (6) recommend only a suture and no graft. If a graft is used, it is possible to use a Kirschner wire, which is drilled out laterally from the osteotomy site and then through the graft and the proximal fragment. If a Kirschner wire is used, it is necessary to leave the wire outside the skin, bending it at 90 degrees to prevent its migration. It is best to remove this wire within 3 to 4 weeks to prevent infection. Because of the clavicle’s complex shape, it is impossible to keep a pin or wire of any strength within the clavicle’s medullary canal. A thin Kirschner wire that can be passed through the medullary canal of the bone provides little fixation and, unless left outside the skin and bent, risks migration. The use of a pin or wire also risks migration. To avoid these problems, the surgeon can use the small reconstruction plates. The plates come in two sizes—2.7 mm and 3.5 mm. Their flexibility makes it possible to contour them exactly to the shape of the clavicle and the graft. Because immobilization is required in an active child, regardless of the method of fixation, the plates are sufficiently strong. A,B: With the graft held temporarily in place between the two resected ends of the clavicle by a small Kirschner wire, the appropriately sized reconstruction plate is contoured using the template provided. C: When the proper shape has been achieved, the plate is attached by screws to both ends of the clavicle and the graft. Each end of the clavicle should be fixed with a minimum of two screws, and at least one screw should hold the graft. The wound is irrigated, a small drain is placed adjacent to the clavicle and brought out through the skin lateral to the incision, and the wound is carefully closed in layers. In young children, a Velpeau dressing is applied and reinforced with a roll of plaster if deemed necessary. In older children, who may be more cooperative with the postoperative immobilization, a commercial sling with a strap that passes around the waist to hold the arm next to the trunk is sufficient. |
noted in infancy. Radiocapitellar incongruity can be a cause of pain and disability later in life (162, 172). Unfortunately, many children present late with pain resulting from radiocapitellar articular changes. There is often chronic discomfort with school and sports activities. On occasion, these children may present with an acute loss of motion attributable to a loose osteochondral fragment. Some individuals remain asymptomatic, and the aesthetics of the deformity is their major concern.
asymptomatic dislocated radial head alone, without painful, progressive restricted range of motion, is not an indication for radial head excision. Indications for radial head excision must include progressive pain, progressive loss of motion, and progressive restriction of activities (174), regardless of age (107).
after excision in order to provide elbow stability. Excision of the lateral olecranon will reportedly provide improved passive elbow flexion and extension, but limitation in active elbow flexion may continue because of deficiencies in the biceps and the brachialis musculature. Tendon transfers for active elbow flexion have reportedly had limited success (188). This condition (and reconstruction) is so rare that in-depth analysis of treatment options is not possible.
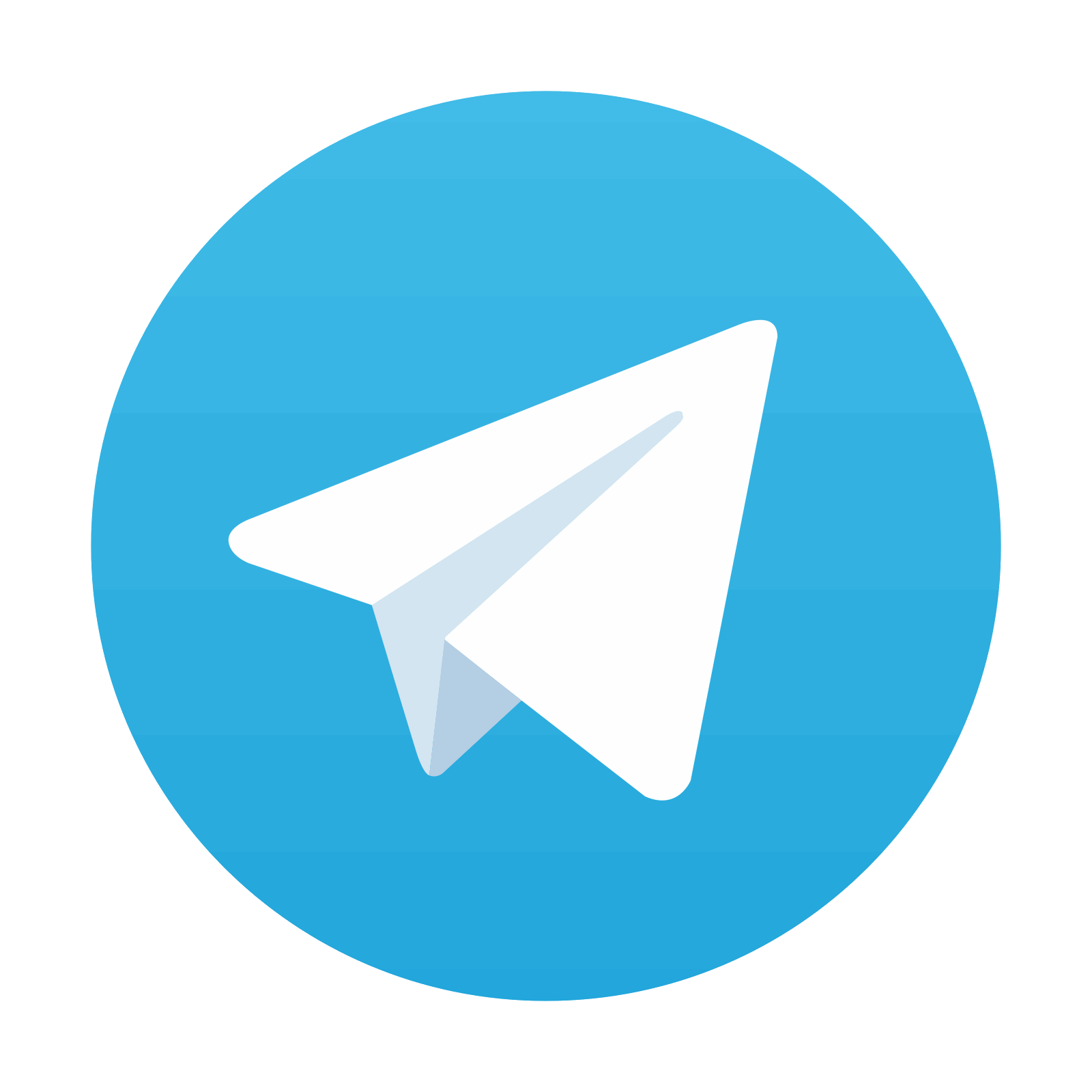
Stay updated, free articles. Join our Telegram channel

Full access? Get Clinical Tree
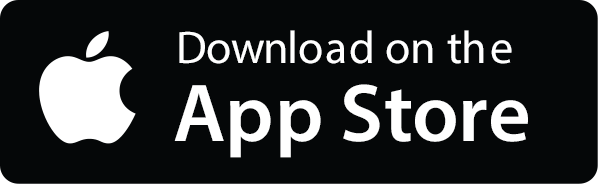
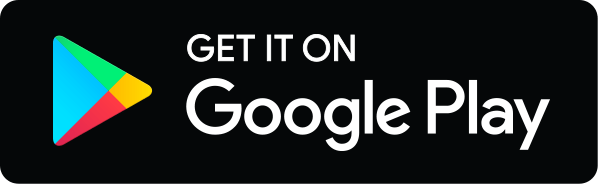
