Using Animal Models in Osteoarthritis Biomarker Research
Osteoarthritis (OA) is characterized by morphologic, molecular, biochemical, and bio-mechanical abnormalities in the articular cartilage and other articular tissues. It has been estimated that over 27 million adults have a clinical diagnosis of OA; therefore, it is the most common form of arthritis.1 OA was historically regarded as a noninflamma-tory arthritis, but there is now cumulative evidence that inflammation has a prominent role in OA.2–5 Signals, including those associated with proinflammatory cytokines, can be sent and received among articular tissues, thus creating a “cross-talk” environment. A complex interaction involving cytokines,3,4,6–13 proteolytic enzymes,7,10,11,13,14 leukocytes,15 synoviocytes,16,17 and chondrocytes3,11–13,18 likely initiates and exacerbates pathologic changes in osteoarthritic joints. Even with this knowledge much of the pathogenesis of OA remains unknown, and diagnosis early in the disease course is rare. Consequently the desire to develop an early OA diagnostic biomarker panel, a process that could have profound ramifications on treatment and prevention of this disease, has exploded.
Animal models are routinely used to study human OA as these models provide significant advantages over clinical human research. The most relevant models have been described in the dog, horse, sheep, goat, guinea pig, rat, rabbit, and mouse, and for more details on each of these models the reader is directed to a series of initiatives.19–27 Highlights of each animal model, as well as a concise list of their advantages and disadvantages ( Table 4.1 ), are included here.
Given that the dog is considered a nearly ideal species for translational investigation of human OA and at least 20% of dogs over 1 year of age in the United States are affected by naturally occurring clinical OA,28 the dog is the most used animal model for investigation of OA.20 Surgical transection of the cranial (anterior) cruciate ligament29 and meniscal transection30 are useful methods of surgical induction, but spontaneous or chemical induction models and additional surgical methods are also described.20 Surgical and chemical induction methods are employed in rabbits and guinea pigs, whereas only surgical induction methods have been reported in sheep and goats.22,23,27 The spontaneous OA-prone Dunkin-Hartley guinea pig31 also offers a unique opportunity to study disease mechanisms. A commonly used surgical model of OA induction in the horse is the osteochondral fragment-exercise model,32–34 which, unlike most other surgical induction models, does not induce joint instability. The medial meniscal tear model with or without concurrent exercise is most often used to induce OA in the rat.21,35,36 While mice can be challenging to study for reasons related to their small size, the availability of genetically modified or knockout mice is invaluable to learning more about OA.26 As an example of animal modeling, the authors will focus on the dog to illustrate the level and limitations of modeling within a specific species.
Biomarkers are “objective indicators of normal biologic processes, pathogenic processes, or pharmacologic responses to therapeutic interventions,”37 and they have been classified under the BIPED scheme: Burden of disease, Investigative, Prognostic, Efficacy of intervention, and Diagnostic.38 Expansion of this classification system is already under way as the Osteoarthritic Research Society International Food and Drug Administration (OARSI FDA) initiative Biomarkers Working Group recently elected to modify this acronym to BIPEDS to include a safety category.39 Another method of biomarker classification involves whether each is a direct or an indirect indicator of OA.40 Direct biomarkers originate from the articular tissues, whereas indirect biomarkers do not arise from the joint tissues but can ultimately affect the joint′s microenvironment.41 Both types provide useful information; therefore, the study of both direct and indirect biomarkers of OA is warranted.
Changes in direct markers reflect extracellular matrix (ECM) catabolism or anabolism and may reveal the status of joint degeneration. Several biomarker candidates causing or resulting from chondrocyte,13,42–46 collagen,11,47–51 or aggrecan52–55 degradation have been assessed, and these include matrix metalloproteases (MMPs), keratan sulfate, cartilage oligomeric matrix protein (COMP), and the collagenase-generated cleavage epitope of type II collagen (C2C or Col2–3/4C). Studies have shown strong correlations between these degradative markers and magnetic resonance imaging (MRI) findings.56–60 Markers associated with bone turnover have also been identified, but they are less commonly considered to be diagnostic markers as these are often altered only during advanced stages of disease.61–64
MMPs are zinc- and calcium-dependent enzymes that degrade specific substrates of the ECM and include stromelysins, collagenases, gelatinases, and membrane metal-loproteases. MMPs have a role in the normal turnover of the connective tissue matrix that occurs during growth and development, but the unchecked production of MMPs is a commonly documented cause of cartilage matrix degradation in OA in animals.48,49,54,65–69 Increased collagen 2 denaturation and fragmentation are often present in OA articular cartilage,48 and MMP13, which is increased in OA chondrocytes, appears to be directly involved.50 Stimulation of canine chondrocytes with interleukin 1β (IL-1β) to represent an in vitro OA environment led to an increase in MMP13 versus controls.3 MMP3 has also been shown to increase in cartilage and synovial fluid from canines with experimentally induced OA,70 and cultured canine chondrocytes stimulated with IL-1β demonstrated increased matrix degradation and MMP3 expression.71
Keratan sulfate, a glycosaminoglycan constituent of the cartilage ECM, has been primarily investigated in animal models using the dog. Synovial fluid keratan sulfate concentrations have been shown to increase72,73 or decrease74,75 in dogs with OA with no clear correlation to factors such as manner of disease initiation (induced vs. spontaneous) or chronicity of disease. Given the discordant results, keratan sulfate may not be ideal for detecting or prognosticating OA, but it may prove useful for serial monitoring of known OA individuals.75
COMP is one of the most comprehensively studied diagnostic biomarker candidates in the synovial fluid, serum, and urine of veterinary patients. Elevations in COMP have been reported in the serum and synovial fluid of naturally occurring OA dogs compared with controls, but elevations due to induced synovitis were also reported, suggesting a lack of specificity (SP) for cartilage damage.46 Another canine study supported these conclusions by showing that a variety of articular tissues, including ligament, tendon, meniscus, and articular cartilage, produced COMP.76 Regardless, COMP may be diagnostically useful as serum and synovial fluid levels have been shown to correlate with canine knee MRI grades of cartilage lesions.60 Urinary COMP was also predictive of the presence of osteophytes in racehorses with carpal fractures.77 Serum COMP concentration was lower in osteoarthritic horses versus normal.78
The C2C or Col2–3/4C has also been investigated in several animal studies with some discordant results. Synovial fluid Col2 levels increased in dogs following induced anterior cruciate rupture.42,79 Serum increases were also detected in the Pond-Nuki model, and these increases correlated with a marker of lipid peroxidation, suggesting a link between oxidative stress and cartilage degeneration.80 In contrast, differences were not detected in synovial fluid, serum, or urine concentrations in dogs with naturally occurring anterior cruciate rupture compared with controls even though lameness, joint effusion, and osteophytes were present.81
The pathophysiology of OA centers on an imbalance of cartilage degradation and synthesis; therefore, several anabolic markers are also under investigation. Anabolic markers reflect molecules that are present in small quantities or entirely absent in health, or they can exhibit an altered structure in which epitopes usually hidden are revealed during OA.51,55,82–86 Chondroitin sulfate (epitopes 3-B-3[−] and 846) is one example of an anabolic marker.
Like keratan sulfate, chondroitin sulfate comprises a large portion of the proteoglycan aggrecan. A monoclonal antibody (3-B-3) recognizes chondroitin sulfate epitopes with (3-B-3[+]) or without (3-B-3[−]) chondroitinase pretreatment. The 3-B-3(−) epitope is identified in the growth plate cartilage during normal growth and development and in the early stages of OA, but it is absent in healthy adult canine cartilage, suggesting anabolic production of an altered form of chondroitin sulfate as an attempted repair response.85,86 Furthermore, it is expressed in superficial zone articular cartilage from destabilized canine knee joints in early stages of disease, and it is detectable before a loss of matrix and proteoglycans is identifiable by toluidine blue staining.86 Synovial fluid values are increased in dogs with naturally occurring OA and experimental OA as early as 4 weeks after surgical destabilization.73,79,85,87 In contrast to synovial fluid data, serum concentrations in dogs with hip dysplasia were lower than in those without joint disease.88
While direct markers may more closely represent the status of joint degeneration, indirect markers, including but not limited to cytokines, can be used to learn more about the processes preceding or leading to the development of OA. Cytokine and chemokine fluctuations within the synovial fluid of osteoarthritic patients have been documented, but comprehensive assessment of the potential clinical significance of those alterations is largely lacking in the literature.9,16,89,90 Cytokines and chemokines have also demonstrated roles in the pathogenesis of OA, including induction of proteinase expression and inhibition of proteoglycan synthesis.91,92 A more thorough exploration of these roles may provide significant information about the pathogenesis of OA and lead to the identification of early OA diagnostic bio-markers. Following stimulation with IL-1β, the up- regulation of cytokines, chemokines, and MMPs was more rapid than the down-regulation of matrix gene expression (COL2A1 and aggrecan), suggesting that these types of molecules may be the first changes identifiable in early OA.12 As a result, the authors were interested in learning more about the cytokine and chemokine profiles of dogs with and without OA and the relationships of these profiles to MMP concentrations.
Our objectives for this study were (1) to delineate the temporal alterations of cytokine, chemokine, and MMP concentrations in synovial fluid, serum, and urine in induced and naturally occurring osteoarthritic dogs in comparison to healthy dogs and (2) to assess the diagnostic value of particular markers through evaluation of sensitivity (SN), SP, and receiver operating characteristic (ROC) curve analysis. We hypothesized there would be strong correlations between cytokine and chemokine fluctuations and the status of the joint with respect to OA.
Materials and Methods
Sample Collection
Part 1: Model Dogs
All procedures were approved by the institution′s animal care and use committee. In this study, 21 adult, intact female, purpose-bred hound dogs >20 kg were included. No more than 24 hours before surgery, blood was drawn from the cephalic vein into serum separator tubes. The serum was harvested within 2 hours of collection, and the specimens were stored in individual, airtight containers at −80°C. Urine was obtained from each dog by aseptic cystocentesis or by manual bladder expression if cystocentesis was not successful. The urine specimens were kept on ice until processing for storage in individual, airtight containers at −20°C. Serum and urine were collected again at 4, 8, and 12 weeks after surgery using the same protocol.
On the day of surgery, each dog was premedicated, anesthetized, and aseptically prepared for arthroscopic surgery of the right stifle (knee). Synovial fluid was collected via aseptic arthrocentesis of the knee joint, and the samples were kept on ice until they were aliquoted and frozen at −80°C for subsequent analysis. Using standard arthroscopic technique and instrumentation for the canine knee,93 one of four surgical procedures was performed on each dog: transection of the anterior cruciate ligament29(ACL-T; n = 5), complete radial transection of the meniscus (MR)30 (n = 5), creation of two 6.0- to 8.0-mm-long full-thickness grooves (GRs) in the cartilage of the weight-bearing portion of the medial femoral condyle (GR; n = 6) using a 3 mm OD (outside diameter) arthroscopic curette, or manipulation of all the aforementioned intra-articular structures without insult using an arthroscopic probe (SHAM; n = 5). Transections were visually confirmed, and GRs were measured with a calibrated probe. The nonoperated, contralateral hind limb served as an internal control for each dog, although synovial fluid was not collected at the time of surgery (baseline). Twelve weeks later, synovial fluid was collected by arthrocentesis from both the operated and contralateral control knees, and aliquots were frozen at −80°C. A second arthroscopic evaluation of the operated joint was performed and articular tissues were collected for histology as part of a concurrent study.
Part 2a: Client-Owned OA Dogs
Informed client consent was obtained for each dog. Blood and synovial fluid were obtained from 10 adult medium and large breed dogs presenting for surgical intervention of unilateral stifle OA (Pre-sx OA; n = 10). These dogs ranged from 3 to 8 years old (median 4.5 years) and included five male castrated and five female spayed dogs. Synovial fluid was obtained from the affected stifle via routine aseptic arthrocentesis, and blood was collected via jugular venipuncture. Synovial fluid samples were kept on ice until they were aliquoted and frozen at −80°C for subsequent analysis. Clinical OA was confirmed in each dog by a board-certified veterinary orthopaedic surgeon as determined by knee physical examination based on the presence of effusion, periarticular fibrosis, pain upon flexion and extension, and a lameness evaluation based on the visual examination of gait at a walk and trot. Radiographic evidence of knee OA including signs of osteophytosis, effusion, and sclerosis was confirmed by a board-certified veterinary radiologist.
All dogs underwent surgery for assessment, lavage, and stabilization of cruciate ligament deficiency and recovered uneventfully. Eight to 12 weeks later, the dogs returned for a postoperative recheck, and blood and synovial fluid were collected again to assess changes in markers after surgical intervention. Two female dogs did not return for follow-up; therefore, their postoperative blood and synovial fluid samples could not be obtained (Post-sx OA; n = 8). These two dogs were subsequently excluded from paired statistical analysis.
Part 2b: Control Group
The normal control group comprised seven medium and large breed adult dogs ranging from 2 to 5 years old (median 2.75 years). There were three castrated males, two spayed females, and two intact males. These dogs had no clinical history of joint trauma, were not lame, and were deemed to be free of clinical OA as determined by a board-certified veterinary orthopaedic surgeon. Radiographic evaluation of the shoulders, knees, and hips verified the absence of OA. Blood and synovial fluid were collected in a similar manner to the OA dogs at a time convenient to the clients, and synovial fluid samples were kept on ice until they were ali-quoted and frozen at −80°C for subsequent analysis.
Multiplex Analysis
An aliquot (25 µL) from each synovial fluid, serum, and urine sample was thawed. The urine and synovial fluid samples were centrifuged at 14,000 rpm for 10 minutes to pellet debris, and the supernatant was removed. The synovial fluid was incubated with hyaluronidase (MP Biomedicals, LLC, Solon, Ohio) at 37°C for 60 minutes to decrease viscosity. Each aliquot was subsequently analyzed in duplicate using a multiplex canine cytokine and chemokine immunoassay (Millipore Corp., St. Louis, MO) based on the xMAP platform (Qiagen Inc., Valencia, CA) for IL-2, IL-4, IL-7, IL-8, IL-15, IL-18, IP-10, interferon gamma (INF-γ), tumor necrosis factor-alpha (TNF-α), monocyte chemoattractant protein 1 (MCP1), keratinocyte-derived chemoattractant (KC), and granulocyte-macrophage colony-stimulating factor (GM-CSF) according to the manufacturers’ directions. The synovial fluid and serum from the client-owned OA dogs and their control group were also analyzed for IL-6 and IL-10 using the same assay. A multiplex human MMP immunoassay (R&D Systems, Minneapolis, MN) based on the xMAP platform for four MMPs: MMP2, MMP3, MMP9, and MMP13 was also used to analyze the client-owned dogs and their control group in duplicate. This MMP assay had been previously shown within our laboratory to cross-react with samples of canine origin.94 Briefly, for each of the xMAP assays the synovial fluid, serum, and urine samples were admixed with antichemokine, cytokine, or MMP monoclonal antibody–charged, small (5.6 micron), polystyrene microspheres in a 96-well plate. Following an overnight incubation at 4°C, a biotinylated polyclonal secondary antibody was added, as well as streptavidin-phycoerythrin. The median fluorescence intensity was determined for each sample. The urine creatinine concentration was measured with an in-house chemistry analyzer (AU400; Olympus America Inc., Irving, TX), and the urine cytokine and chemokine values were standardized to this concentration (pg/mg).
Statistics
Planned comparisons between preoperative and postoperative samples (synovial fluid, serum, and urine) and between operated and contralateral limbs (synovial fluid only) were performed with the paired t-test. Comparisons between surgery model groups or between OA and normal individuals were performed with the unpaired t-test or the Mann–Whitney rank sum test (SigmaStat 3.5; Systat Software, Inc., San Jose, CA). Significance was set at p < 0.05. SN and SP were calculated for select markers of interest using the histopathological data as the reference test for the model dogs and the clinical examination and radiographic data for the hospital patients. When possible, Youden′s index was used to select optimal concentration cut-offs that maximized SN and SP for each marker. When this index did not provide a balance between SN and SP, concentration cut-offs that selectively led to higher SN were chosen. The 95% confidence intervals (CIs) were calculated using the Clopper–Pearson method.
ROC Curves
ROC curves based on the logistic regression model were developed for markers of interest to the authors following initial statistical analysis. These curves were created and area under the curve (AUC) calculated for assessment of diagnostic value of certain parameters using JMP 7.0.2 software (SAS Institute, Cary, NC).
Results
Part 1: Induction of OA
Arthroscopic findings ( Fig. 4.1a, b ), lameness scoring performed by a board-certified veterinary surgeon, and histologic scoring performed by a board-certified veterinary pathologist confirmed the induction of clinically significant OA in the ACL-T, GR, and MR groups, whereas evidence of OA was not identified in the SHAM dogs. The ACL-T and MR dogs exhibited the most severe joint pathologic changes (although characteristically different from each other), but GR dogs also had articular cartilage damage and synovitis. Both investigators were blinded with respect to the group during evaluation.

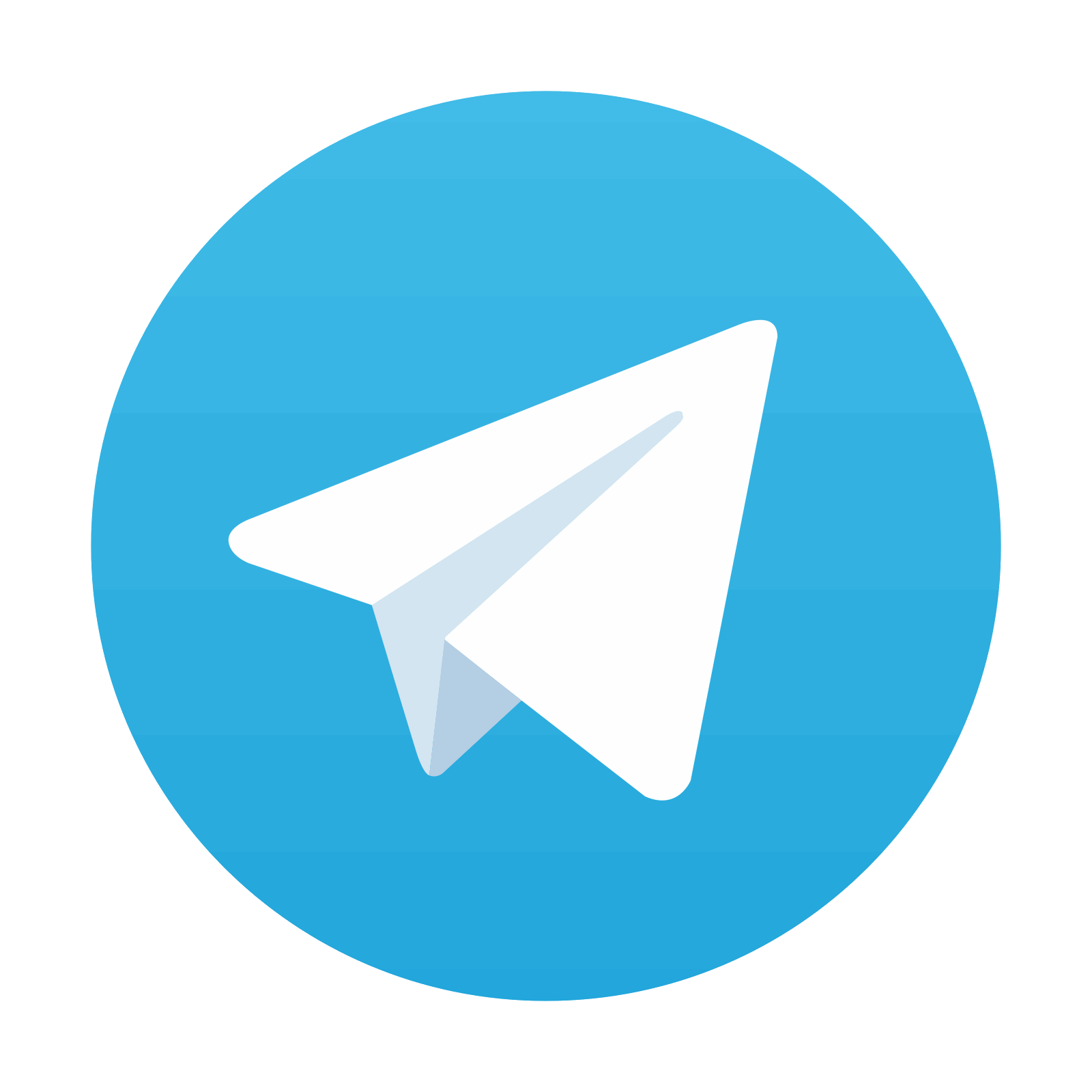
Stay updated, free articles. Join our Telegram channel

Full access? Get Clinical Tree
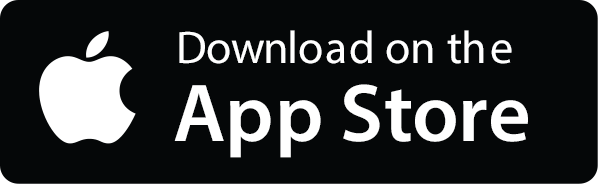
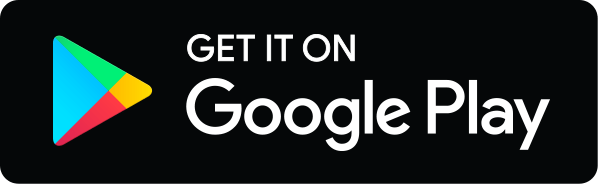
