Fig. 12.1
Anterior (L) and posterior (R) views of bone in the shoulder joint
The scapula is located on the posterolateral thoracic wall behind the second through seventh ribs, forming the back portion of the shoulder girdle. The flat and triangular body of the scapular has three processes called the acromion, spine, and coracoid process. The horizontally oriented scapular spine separates the smaller supraspinous fossa from the infraspinous fossa. The scapular spine and fossae provide attachment sites for muscles that move the arm. The spine ends in the large flat acromion that articulates with the clavicle and is an attachment site for chest and arm muscles. Below the acromion is the glenoid fossa that acts as a shallow socket for the head of the humerus. The coracoid process is a thick curved structure that projects over the glenoid fossa and directed anteriorly. The coracoid process serves as an attachment for ligaments and muscles of the arm and chest. In the anatomic position, the scapula is deviated 35° anterior to the frontal plane.
The humerus is the longest and largest bone of the upper limb. The shaft of the humerus is cylindrical in its proximal half and flattened in the anterior-posterior direction in the distal half. The head of the humerus articulates with the glenoid fossa of the scapula to form the glenohumeral joint. There are two protuberances on the proximal humerus, the greater and lesser tubercles. They serve as attachment sites for rotator cuff muscles. Retroversion of the humeral head is roughly 30° posterior to the medial-lateral axis at the elbow.
Two bones form the forearm (Fig. 12.2). The longer one of the two and located on the medial aspect of the forearm is the ulna. The proximal end of the ulna has a posterior projection called olecranon, which forms the prominence of the elbow, and an anterior projection called coronoid process. The olecranon and coronoid process together form the trochlear notch that articulate with the humerus. Radius is the bone located on the lateral aspect of the forearm. The proximal end of the radius has a disc-shaped head that articulates with the humerus. Distally, the shaft of the radius widens at the wrist, with a styloid process pointing laterally.
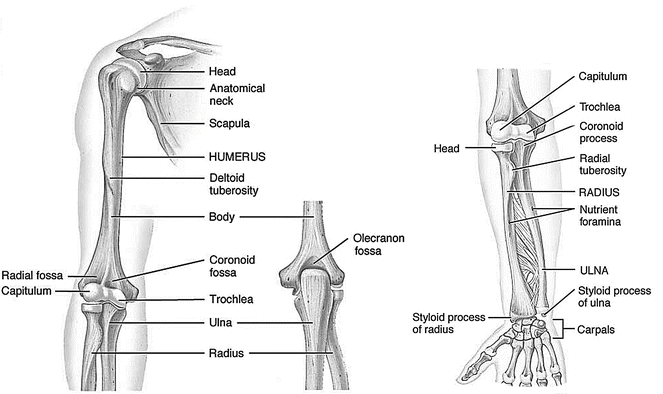
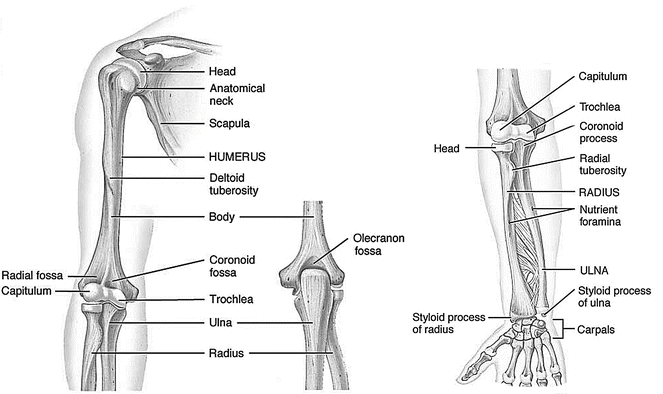
Fig. 12.2
Bones of the upper extremity
12.1.2 Joints
The shoulder girdle is comprised of three synovial joints and one gliding mechanism involving the sternum, clavicle, scapula, ribs, and humerus. These articulations, namely the sternoclavicular, acromioclavicular, and glenohumeral joints and the scapulothoracic gliding mechanism, function in a coordinated manner to allow complex arcs of motion of the upper limb. The most proximal joint within the shoulder girdle is the sternoclavicular joint, which connects the appendicular skeleton to the axial skeleton. The sternoclavicular joint is formed by the medial end of the clavicle articulating with the manubrium of the sternum just above the first rib. Approximately half of the medial end of the clavicle protrudes above the shallow sternal socket. The sternal surface is saddle shaped concave anteroposteriorly and convex downwardly [1]. In between the articular surfaces is a strong, nearly circular fibrocartilaginous disc, which is attached superiorly to the upper medial end of the clavicle. The disc functions as a sliding hinge that facilitates a greater range of motion at this joint and stabilizes the joint against medially directed forces transmitted through the clavicle.
The lateral end of the clavicle articulates with the acromion process, forming the acromioclavicular joint. This articulation forms the roof of the shoulder. The acromioclavicular joint has oblique surfaces that allow the acromion, and thus the scapula, to glide forward or backward over the clavicle. This movement of the scapula keeps the glenoid fossa continuously facing the humeral head. The acromioclavicular joint is not as mobile as the large glenohumeral joint but has an important function in contributing to total arm movement, especially when the shoulder is overhead or across the chest (adducted). The acromioclavicular joint also facilitates force transmission between the clavicle and the acromion.
The articulation that forms between the glenoid fossa of the scapular and the head of the humerus is the glenohumeral joint (Fig. 12.3). The glenohumeral joint is a multi-axial ball-and-socket joint. The joint surfaces are asymmetrical and incongruent. The surface area of the pear shaped glenoid fossa is one-third to one-fourth that of the humeral head; as a result of the mismatch, only a portion of the humeral head can be in articulation in any position of the joint. Along the margin of the glenoid fossa attaches a rim of fibrocartilaginous tissue caked the glenoid labrum. The labrum is thought to deepen the articular cavity, protect the edges of the bone, and assist in lubrication of the joint [2]. Typical healthy glenohumeral range of motion is 120° of abduction, 120–180° of flexion, 45–55° of extension, 75–85° of internal rotation, and 60–70° of external rotation. This high mobility afforded by the glenohumeral joint is at the expense of joint stability.
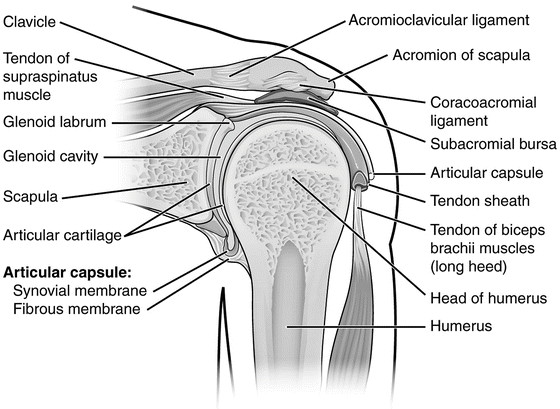
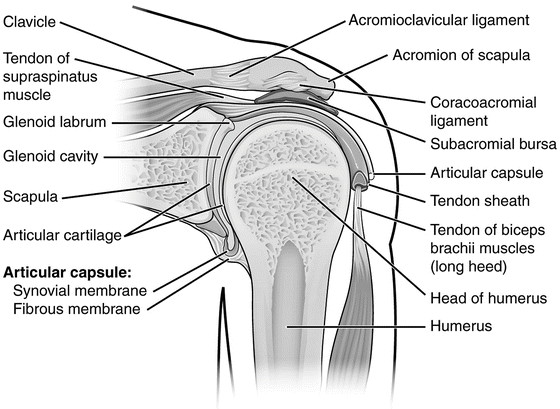
Fig. 12.3
Coronal section through right glenohumeral joint (posterior view)
The scapulothoracic gliding mechanism is not a true joint but is the riding of the concave anterior surface of the scapula along the convex posterolateral surface of the thoracic cage (ribs 2–7). The thorax and scapula are separated by the subscapularis and serratus anterior muscles, which glide over each other during movements of the scapula. The scapula is held in close approximation to the chest wall by muscular attachments. In movements of the shoulder complex, the scapula can be protracted, retracted, elevated, depressed, and rotated about a variable axis perpendicular to its flat surface. However, fibrous adhesions can sometimes occur following a shoulder injury, particularly if the joint has been immobilized for a long period of time.
The elbow joint is a modified hinge joint composed of two articulations, where the humerus connects to the radius and ulna. The humeroradial joint is formed by the head of the radius articulates with capitulum of the humerus. The humeroulnar joint is formed by the trochlear notch of the ulna articulate with the trochlea of the humerus (Fig. 12.4). The radius and ulna also articulates with one another at the proximal and distal ends, called radioulnar joints, and through a strong fibrotic band known as the syndesmosis in between the two end joints.
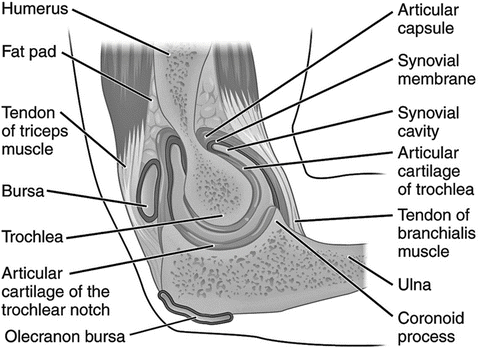
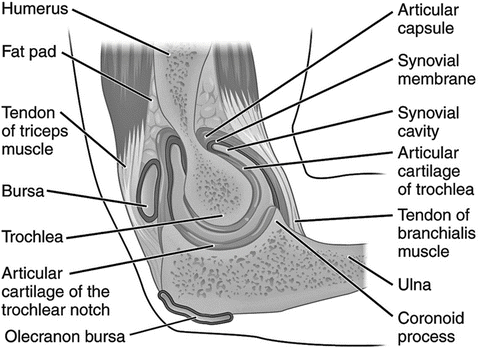
Fig. 12.4
Sagittal section through right elbow (lateral view)
12.1.3 Ligaments
Because the articular surfaces of the glenohumeral joint contribute little to joint stability, joint capsule reinforced by a group of ligaments connecting the humerus to the glenoid become the main source of stability. The coracohumeral ligament is one of the most important ligamentous structures in the shoulder complex [3]. This ligament extends from the coracoid process of the scapula to the greater tubercle of the humerus. The downward pull of gravity on the arm is counteracted largely by the superior capsule and the coracohumeral ligament. Also because it is located anterior to the axis of axial rotation of the humerus, the coracohumeral ligament restricts lateral rotation and extension.
The three glenohumeral ligaments lie on the anterior aspect of the joint (Fig. 12.5). The superior glenohumeral ligament originates from the supraglenoid tubercle and inserts on the humerus near the lesser tuberosity. The superior glenohumeral ligament, together with the coracohumeral ligament and the supraspinatus muscle, assists in preventing downward displacement of the humeral head. The middle glenohumeral ligament originates from the supraglenoid tubercle and anterosuperior region of the labrum, passes laterally, gradually enlarges, and attaches to the anterior aspect of the anatomical neck of the humerus. The middle glenohumeral ligament limits lateral rotation up to 90° of abduction. The inferior glenohumeral ligament is a hammock-like structure with anchor points on the anterior and posterior sides of the glenoid. The ligament originates from the anterior, inferior, and posterior margins of the glenoid labrum and inserts laterally to the inferior aspects of the humeral neck. The superior band strengthens the capsule anteriorly and supports the joint most effectively in the middle ranges of abduction. The inferior band supports the joint most effectively in the upper ranges of abduction and also prevents anteroinferior subluxation and dislocation [4].
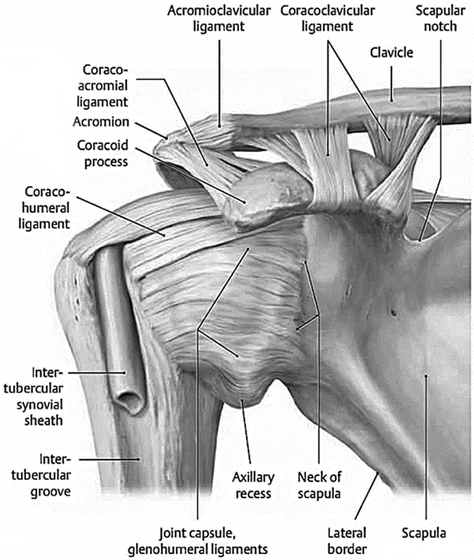
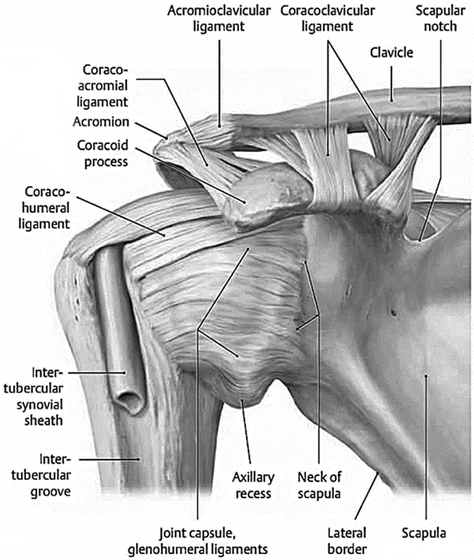
Fig. 12.5
Major ligaments of the shoulder (posterior view)
The coracoacromial ligament is a strong triangular ligament originated from the lateral border of the coracoid process and inserted to the top of the acromion process. Together with the acromion and the coracoid processes, the coracoacromial ligament forms an important protective arch over the glenohumeral joint and a restraining socket for the humeral head. This protects the joint from trauma from above and preventing dislocation of the humeral head superiorly.
The clavicle is held in place by strong ligaments at both ends. Around the sternoclavicular joint, the anterior and posterior sternoclavicular ligaments are found anterior and posterior to the joint; the interclavicular ligament links the ends of the two clavicles to each other and to the superior surface of the manubrium of the sternum; and the costoclavicular ligament that is positioned laterally to the joint and links the proximal end of the clavicle to the first rib and its related costal cartilage. On the lateral side, the acromioclavicular ligament strengthens the acromioclavicular joint and binds the clavicle to the scapula. The coracoclavicular ligament consists of two parts: trapezoid and conoid. These two components, functionally and anatomically distinct, are united at their corresponding borders. These ligaments suspend the scapula from the clavicle and transmit the force of the superior fibers of the trapezius to the scapula.
There are two strong ligament complex at the elbow joint, the medial collateral ligaments (MCL) on the ulna side and the lateral collateral ligaments (LCL) on the radius side (Fig. 12.6). The MCL complex consists of three ligaments: the anterior oblique, posterior oblique, and transverse ligaments, originated from the anteroinferior surface of the medial epicondyle. The MCL complex is a primary medial stabilizer of the flexed elbow joint. The LCL complex is made up of four ligaments: the lateral ulnar collateral ligament, radial collateral ligament, annular ligament, and accessory lateral collateral ligament, originated along the inferior surface of the lateral epicondyle. The LCL complex is the primary stabilizer of the elbow for varus and external rotation forces.
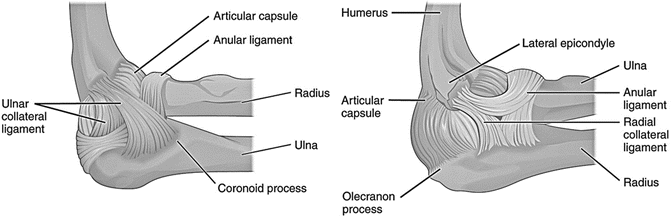
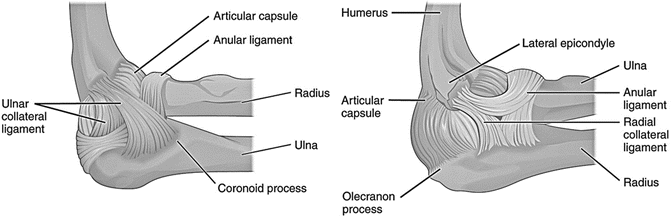
Fig. 12.6
Ligaments of the elbow (Left: medial view, Right: lateral view)
12.1.4 Muscles
The shoulder joint is surrounded by small muscles (rotator cuff) that stabilize the glenohumeral joint and large muscles that power its strong movements. The rotator cuff is a conjoined tendon of four muscles, the supraspinatus, infraspinatus, teres minor, and subscapularis. The rotator cuff muscles predominantly controls the dynamic stability of the glenohumeral joint but also contribute significantly to shoulder movement. All these muscles except subscapular attach to greater tuberosity of head of humerus, while subscapular muscle tendon attaches to lesser tuberosity. The supraspinatus is an initiator of abduction, and active throughout the range of shoulder abduction. It is the most common site for tear. The infraspinatus and teres minor lie below the scapular spine and are external rotators of the shoulder. The infraspinatus is mainly active with the arm in neutral and the teres minor contributes more when the arm is in 90° of abduction. The subscapularis is the main internal rotator of the shoulder. It is the largest and strongest rotator cuff muscle, providing 53 % of total strength.
Several muscles are responsible for elevation of the arm. The deltoid muscle is the only shoulder elevator if the supraspinatus is torn and dysfunctional. It comprises anterior, middle and posterior portions which are more active depending on the direction of arm elevation. The long head of biceps passes over the humeral head curving in two planes, and is recognized as providing a small degree of stability to the glenohumeral joint. This is predominantly with abduction and external rotation of the arm in the scapular plane. The scapular muscles that control upward rotation and protraction of the scapulothoracic joint are the serratus anterior and trapezius muscles. The latissimus dorsi and the sternocostal head of the pectoralis major are the largest adductor and extensor muscles of the shoulder. The teres major, long head of the triceps, posterior deltoid, infraspinatus, and teres minor are also primary muscles for shoulder adduction and extension. The primary muscles that internally rotate the humerus are the subscapularis, anterior deltoid, pectoralis major, latissimus dorsi, and teres major. External rotators of the humerus are the infraspinatus, teres minor, and posterior deltoid.
At the elbow joint, the primary flexor muscles include the biceps brachii, brachialis, and brachioradialis. The triceps brachii and anconeus muscles are the elbow extensors. The muscles that are responsible for forearm supination are the biceps brachii and supinator muscles. The pronator muscles of the forearm are the pronator quadratus located in the distal portion of the forearm and pronator teres on the proximal portion of the forearm.
12.2 Component Biomechanics and Tolerance
12.2.1 Clavicle
The clavicle is the most commonly fractured bone in the human body. The majority of clavicular fractures (75–80 %) occur within the middle third of the bone. In males, the annual incidence was highest under 20 years of age, decreasing in each subsequent cohort until the seventh decade. In females, the incidence was more constant, but relatively frequent in teenagers and the elderly. The common mechanism of clavicle fracture, regardless of site, is a direct blow on the shoulder such as occurs during a fall or road traffic accident [5].
Several studies have investigated the impact forces involved in clavicle fracture. Duprey et al. conducted dynamic axial compression tests until failure on 18 clavicles using a drop tower system (6 kg impactor mass), at four impact speeds between 1 and 2.5 m/s [6]. A schematic of the system is shown (Fig. 12.7). Clavicle fractures occurred at an average velocity of 1.41 ± 0.4 m/s, with an average fracture force of 1.48 ± 0.46 kN and average deflection of 5.4 ± 1.1 mm. Failure force of female clavicles was significantly lower than the male, and at the slowest impact speed (1 m/s) only female clavicles ruptured. Effect from bone mineral density (BMD) on failure force was not detected due to small samples. In a comprehensive imaging study, Harrington et al. investigated the cross-sectional geometric properties of 15 adult clavicles from five females and ten males [7]. Cross-sectional area, anatomic moments of inertia, and porosity along the length of the clavicle were measured. Mechanical properties, including axial, flexural, and torsional rigidities and critical buckling force were derived using beam theory based on the geometric data. The clavicle was found to be weakest in the central third of its length. Analysis of Euler buckling predicted a minimum critical force for buckling during axial loading of approximately two to three body weights for an average adult, estimation in support of findings from an early study [6].
Mechanical response of the clavicle was reported by Kemper et al. [8]. Ten cadaver clavicles were tested in three–point bending, with impact delivered by a servo hydraulic actuator at a velocity of 0.15 m/s. A schematic of the system is shown (Fig. 12.7). The average failure load found was 732 ± 175 Nm and the average failure moment was 28.3 ± 7.8 Nm. Most clavicles fractured in the mid-shaft region in the form of a transverse, oblique, or comminuted-wedge fracture.
12.2.2 Humerus
Early tolerance studies of the humerus were conducted using three-point bending loading technique and maximum bending moments were reported. In one of the earliest studies conducted in 1859, according to a 1995 citation moments for males and females were 115 and 73 Nm [9, 10]. Greater moments of 151 and 85 Nm were reported for males and females in the1880 study, according to the cited 1995 reference [11]. In a more recent study reported in 1970, the average fracture load for the humerus was determined to be 1,330 N and this was associated with the quasi-static load applied in the antero-posterior (AP) direction [12]. Studies conducted in the 1990s have determined the effect of loading direction (AP versus lateral-medial, ML) and rate (218 and 0.635 mm/s) on the shear forces, AP and lateral bending moments corresponding to the ML and AP loadings [13]. These authors subjected 24 specimens from one female and 13 male PMHS with age ranging from 44 to 75 years, to three-point bending. The overall moments and shear forces for all combinations of variables were 155 ± 44.6 Nm (±denotes standard deviation) and 1.96 ± 0.67 kN. These authors normalized the data to fifth and fiftieth percentile anthropometries using the impulse-momentum approach [14]. The summary of data is shown in Table 12.1. Because of the lack of statistical difference (p > 0.05) between the two loading directions and rates, the authors suggested the following magnitudes to represent the tolerance of the humerus: fiftieth percentile: 2.5 kN and 230 Nm; and fifth percentile: 1.7 kN and 130 Nm (Table 12.1). Fractures transverse to the longitudinal axis were common and this was followed by angled and spiral/irregular fractures.
Table 12.1
Summary of humerus tolerance from three-point bending tests (refer to text for details)
Standard for normalization | Injury metric | Lateral-medial loading | Antero-posterior loading |
---|---|---|---|
Fiftieth percentile | Force (kN) | 2.68 ± 0.69 | 2.17 ± 0.28 |
Moment (Nm) | 236 ± 57.7 | 226 ± 77.3 | |
2.5 kN and 230 Nm for both loadings | |||
Fifth percentile | Force (kN) | 1.86 ± 0.48 | 1.51 ± 0.20 |
Moment (Nm) | 137 ± 33.6 | 131 ± 44.9 | |
1.7 kN and 130 Nm for both loading |
A later study used 9.48-kg dropping mass, similar to those used in the clavicle studies described above, described earlier, to impact 12 female humerii under the three-point bending configuration at a velocity of 3.6 m/s [15]. The peak failure moment was 154 ± 27 Nm and the normalized peak moment corresponding to the fifth percentile anthropometry was 128 ± 19 Nm [16]. The impactor force is shown (Fig. 12.8, the dashed and dotted curves are not the subject matter of this chapter).
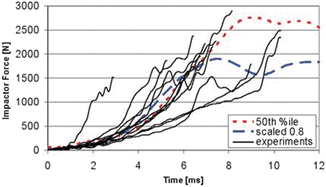
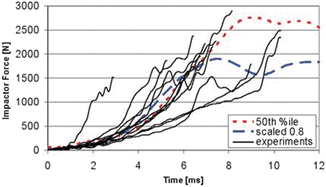
Fig. 12.8
Force recorded from the impacting mass accounting for inertial compensation from the 12 humerii tests, from Ref. [17] (Reprinted with permission from the Stapp Association)
12.2.3 Forearm and Elbow
Like the humerus, early human forearm tests were done using quasi-static loading. For example, a 1970 study found that the failure of ulna and radius occurs at 630 and 520 N [12]. Dynamic tests were conducted to determine effects of pronation and supination tolerance and potential application to airbag loading in motor vehicle crashes [18]. Two drop tests were conducted at 4.6 m/s with a 9-kg impacting mass. In the first, the forearm was pronated with the distal third of the ulna oriented towards the impacting mass (simulating ‘natural driving posture’), and in the second, the specimen was fully supinated position with the anterior surface of the wrist facing the mass. The peak failure loads and moments for the supinated and pronated forearms were 2.1 and 1.5 kN, and 115 and 80 Nm, respectively. Ulna fractures preceded radius fractures in both specimens.
In a later study, Pintar et al. evaluated the response of the PMHS forearms at dynamic rates using a material testing device [19]. They subjected 30 forearm specimens (12 female and 18 male) with age ranging from 41 to 89 years to three-point bending from 15 PMHS (Fig. 12.9). Loading velocities were 3.3 and 7.6 m/s in the two groups of equally divided specimens. Thirteen had butterfly fractures of the ulna (10 from male specimens). Out of the 16 comminuted fracture specimens, 11 were from the high rate of loading. There were no statistical (p > 0.05) differences on the fracture location based on the two loading rates and no statistical differences were apparent with regard to the anatomical aspect (p > 0.05). However, peak force and bending moments were significantly (p < 0.05) lower in female than male specimens. These results clearly indicated that the tolerances of female forearms to three-point bending are lower than males (Table 12.2). Furthermore, compared to the quasi-static tests, these results showed a dynamic factor of 1.7 to account for the increased rate of loading. This factor was found to be in-line with similar data for other bones. For example, the dynamic rise factor is reported to be 1.68 and 1.66 for the tibia and femur bones [20, 21]. Based on this research, using the mean moment of 94 Nm for all specimen as the basis, Pintar et al. proposed a tolerance of 47 Nm for a smaller size occupant [19]. Fractures from this study are shown (Fig. 12.10).
