Understanding and Managing the Healing Process Through Rehabilitation
William E. Prentice, PhD, PT, ATC, FNATA
After reading this chapter,
the athletic training student should be able to:
- Describe the pathophysiology of the healing process.
- Identify the factors that can impede the healing process.
- Discuss the etiology and pathology of various musculoskeletal injuries associated with various types of tissues.
- Compare healing processes relative to specific musculoskeletal structures.
- Explain the importance of initial first aid and injury management of these injuries and their impact on the rehabilitation process.
- Discuss the use of various analgesics, anti-inflammatories, and antipyretics in facilitating the healing process during a rehabilitation program.
Figure 2-1. A cycle of sport-related injury. (Adapted from Booher JM, Thibadeau GA. Athletic Injury Assessment. St. Louis, MO: Mosby; 2000.)16
Injury rehabilitation requires sound knowledge and understanding of the etiology and pathology involved in various musculoskeletal injuries that may occur.17,71 When injury occurs, the athletic trainer is charged with designing, implementing, and supervising the rehabilitation program. Rehabilitation protocols and progressions must be based primarily on the physiologic responses of the tissues to injury and on an understanding of how various tissues heal.62 Thus, the athletic trainer must understand the healing process to effectively supervise the rehabilitative process. This chapter discusses the healing process relative to the various musculoskeletal injuries that may be encountered by an athletic trainer.
UNDERSTANDING THE HEALING PROCESS
Rehabilitation programs must be based on the cycle of the healing process (Figure 2-1). The athletic trainer must have a sound understanding of the sequence of the various phases of the healing process. The physiologic responses of the tissues to trauma follow a predictable sequence and time frame.35 Decisions on how and when to alter and progress a rehabilitation program should be primarily based on recognition of signs and symptoms, as well as on an awareness of the time frames associated with the various phases of healing.46 The healing process consists of the inflammatory response phase, fibroblastic repair phase, and maturation remodeling phase. It must be stressed that although the phases of healing are presented as 3 separate entities, the healing process is a continuum. Phases of the healing process overlap one another and have no definitive beginning or end points.63
Figure 2-2. Initial injury and inflammatory response phase of the healing process. (A) Cut blood vessels bleed into the wound. (B) Blood clot forms, and leukocytes clean the wound. (C) Blood vessels regrow, and granulation tissue forms in the fibroblastic repair phase of the healing process. (D) Epithelium regenerates, and connective tissue fibrosis occurs in the maturation-remodeling phase of the healing process. (Adapted from McKinley M, O’Loughlin V. Human Anatomy: An Integrative Approach. Chicago, IL: McGraw-Hill; 2015.)
Primary Injury
Primary injuries are almost always described as being either chronic or acute in nature, resulting from macrotraumatic or microtraumatic forces. Injuries classified as macrotraumatic occur as a result of acute trauma and produce immediate pain and disability. Macrotraumatic injuries include fractures, dislocations, subluxations, sprains, strains, and contusions. Microtraumatic injuries are most often called overuse injuries and result from repetitive overloading or incorrect mechanics associated with repeated motion.5 Microtraumatic injuries include tendinitis, tendinosis, tenosynovitis, bursitis, etc. A secondary injury is essentially the inflammatory or hypoxia response that occurs with the primary injury.
Inflammatory Response Phase
Once a tissue is injured, the process of healing begins immediately88 (Figure 2-2A). The destruction of tissue produces direct injury to the cells of the various soft tissues. Cellular injury results in altered metabolism and the liberation of materials that initiate the inflammatory response. It is characterized symptomatically by redness, swelling, tenderness, and increased temperature.20 This initial inflammatory response is critical to the entire healing process.72 If this response does not accomplish what it is supposed to or if it does not subside, normal healing cannot take place.
Inflammation is a process through which leukocytes and other phagocytic cells and exudates are delivered to the injured tissue. This cellular reaction is generally protective, tending to localize or dispose of injury byproducts (eg, blood and damaged cells) through phagocytosis, thus setting the stage for repair. Local vascular effects, disturbances of fluid exchange, and migration of leukocytes from the blood to the tissues occur.70
Clinical Decision-Making Exercise 2-1
A physical education student fell on his wrist playing flag football. It is very swollen, and he has decreased strength and range of motion. The athletic trainer does not suspect a fracture. A decision is made to provide an initial treatment instead of sending the student to the emergency room. What should the athletic trainer’s goals be at this time?
Hemostasis
Hemostasis is a vascular reaction that involves vascular spasm, formation of a platelet plug, blood coagulation, and growth of fibrous tissue.65 The immediate response to tissue damage is a vasoconstriction of the vascular walls in the vessels leading away from the site of injury that lasts for about 5 to 10 minutes. This vasoconstriction presses the opposing endothelial wall linings together to produce a local anemia that is rapidly replaced by hyperemia of the area as a result of vasodilation.18 This increase in blood flow is transitory and gives way to slowing of the flow in the dilated vessels, thus enabling the leukocytes to slow down and adhere to the vascular endothelium. Eventually, there is stagnation and stasis. The initial effusion of blood and plasma lasts for 24 to 36 hours.
Chemical Mediators
The events in the inflammatory response are initiated by a series of interactions involving several chemical mediators, which cause the vascular and cellular changes resulting from inflammation. Some of these chemical mediators are derived from the invading organism, some are released by the damaged tissue, others are generated by several plasma enzyme systems, and still others are products of various white blood cells participating in the inflammatory response. Four chemical mediators—cytokines, leukotrienes, prostaglandins, and histamine—are important in limiting the amount of exudate, and thus swelling, after injury. Cytokines, in particular chemokines and interleukin, are the major regulators of leukocyte traffic and help to attract leukocytes to the actual site of inflammation. Responding to the presence of chemokines, phagocytes enter the site of inflammation within a few hours. Leukotrienes and prostaglandins are responsible for margination, in which leukocytes (neutrophils and macrophages) adhere along the cell walls. They also increase cell permeability locally, thus affecting the passage of the fluid and white blood cells through cell walls via diapedesis to form exudate. Consequently, vasodilation and active hyperemia are important in exudate (plasma) formation and in supplying leukocytes to the injured area. Histamine, released from the injured mast cells, causes vasodilation and increased cell permeability, owing to a swelling of endothelial cells and then separation between the cells. The amount of swelling that occurs is directly related to the extent of vessel damage.
Formation of a Clot
Platelets do not normally adhere to the vascular wall. However, injury to a vessel disrupts the endothelium and exposes the collagen fibers. Platelets adhere to the collagen fibers to create a sticky matrix on the vascular wall, to which additional platelets and leukocytes adhere and eventually form a plug. These plugs obstruct local lymphatic fluid drainage and thus localize the injury response (Figure 2-2B).
The initial event that precipitates clot formation is the conversion of fibrinogen to fibrin. This transformation occurs because of a cascading effect, beginning with the release of a protein molecule called thromboplastin from the damaged cell. Thromboplastin causes prothrombin to be changed into thrombin, which, in turn, causes the conversion of fibrinogen into a very sticky fibrin clot that shuts off blood supply to the injured area. Clot formation begins around 12 hours after injury and is completed within 48 hours.
As a result of a combination of these factors, the injured area becomes walled off during the inflammatory stage of healing. The leukocytes phagocytize most of the foreign debris toward the end of the inflammatory phase, setting the stage for the fibroblastic phase. This initial inflammatory response lasts for about 2 to 4 days after initial injury.
Clinical Decision-Making Exercise 2-2
A back-stroker suffered a second-degree latissimus dorsi strain. The coach wants to know why he can’t be ready to compete the next day. What should the athletic trainer tell the coach about the healing process and how long it may take the strain to heal?
Chronic Inflammation
A distinction must be made between the acute inflammatory response as previously described and chronic inflammation. Chronic inflammation occurs when the acute inflammatory response does not respond sufficiently to eliminate the injuring agent and restore tissue to its normal physiologic state. Thus, only low concentrations of the chemical mediators are present. The neutrophils that are normally present during acute inflammation are replaced by macrophages, lymphocytes, fibroblasts, and plasma cells. As this low-grade inflammation persists, damage occurs to connective tissue, resulting in tissue necrosis and fibrosis prolonging the healing process. Chronic inflammation involves the production of granulation tissue and fibrous connective tissue. These cells accumulate in a highly vascularized and innervated loose connective tissue matrix in the area of injury.75 The specific mechanisms that cause an insufficient acute inflammatory response are unknown, but they appear to be related to situations that involve overuse or overload with cumulative microtrauma to a particular structure.11 There is no specific time frame in which the acute inflammation transitions to chronic inflammation. It does appear that chronic inflammation is resistant to both physical and pharmacologic treatments.72
Use of Anti-Inflammatory Medications
A physician will routinely prescribe non-steroidal anti-inflammatory drugs (NSAIDs) for a patient who has sustained an injury.79 These medications are certainly effective in minimizing pain and swelling associated with inflammation and can enhance return to normal activity. However, there are some concerns that the use of NSAIDs acutely following injury might actually interfere with inflammation, thus delaying the healing process.
Fibroblastic Repair Phase
During the fibroblastic phase of healing, proliferative and regenerative activity leading to scar formation and repair of the injured tissue follows the vascular and exudative phenomena of inflammation35 (Figure 2-2C). The period of scar formation referred to as fibroplasia begins within the first few hours after injury and can last as long as 4 to 6 weeks. During this period, many of the signs and symptoms associated with the inflammatory response subside. The patient might still indicate some tenderness to touch and will usually complain of pain when particular movements stress the injured structure. As scar formation progresses, complaints of tenderness or pain gradually disappear.26
During this phase, growth of endothelial capillary buds into the wound is stimulated by a lack of oxygen, after which the wound is capable of healing aerobically.20 Along with increased oxygen delivery comes an increase in blood flow, which delivers nutrients essential for tissue regeneration in the area.20
The formation of a delicate connective tissue called granulation tissue occurs with the breakdown of the fibrin clot. Granulation tissue consists of fibroblasts, collagen, and capillaries. It appears as a reddish granular mass of connective tissue that fills in the gaps during the healing process.
As the capillaries continue to grow into the area, fibroblasts accumulate at the wound site, arranging themselves parallel to the capillaries. Fibroblastic cells begin to synthesize an extracellular matrix that contains protein fibers of collagen and elastin, a ground substance that consists of nonfibrous proteins called proteoglycans, glycosaminoglycans, and fluid. On about day 6 or 7, fibroblasts also begin producing collagen fibers that are deposited in a random fashion throughout the forming scar. As the collagen continues to proliferate, the tensile strength of the wound rapidly increases in proportion to the rate of collagen synthesis. As the tensile strength increases, the number of fibroblasts diminishes, signaling the beginning of the maturation phase.
This normal sequence of events in the repair phase leads to the formation of minimal scar tissue. Occasionally, a persistent inflammatory response and continued release of inflammatory products can promote extended fibroplasia and excessive fibrogenesis, which can lead to irreversible tissue damage.9 Fibrosis can occur in synovial structures, as with adhesive capsulitis in the shoulder, in extra-articular tissues including tendons and ligaments, in bursa, or in muscle.
Clinical Decision-Making Exercise 2-3
A cross-country runner strained her quadriceps. How will the healing process for this injury differ from the process for a ligamentous injury?
The Importance of Collagen
Collagen is a major structural protein that forms strong, flexible, inelastic structures that hold connective tissue together. There are at least 16 types of collagen, but 80% to 90% of the collagen in the body consists of types I, II, and III. Type I collagen is found in skin, fascia, tendon, bone, ligaments, cartilage, and interstitial tissues; type II can be found in hyaline cartilage and vertebral discs; and type III is found in skin, smooth muscle, nerves, and blood vessels. Type III collagen has less tensile strength than does type I and tends to be found more in the fibroblastic repair phase. Collagen enables a tissue to resist mechanical forces and deformation. Elastin, however, produces highly elastic tissues that assist in recovery from deformation. Collagen fibrils are the load-bearing elements of connective tissue. They are arranged to accommodate tensile stress but are not as capable of resisting shear or compressive stress. Consequently, the direction of orientation of collagen fibers is along lines of tensile stress.52
Collagen has several mechanical and physical properties that allow it to respond to loading and deformation, permitting it to withstand high tensile stress. The mechanical properties of collagen include elasticity, which is the capability to recover normal length after elongation; viscoelasticity, which allows for a slow return to normal length and shape after deformation; and plasticity, which allows for permanent change or deformation. The physical properties include force relaxation, which indicates the decrease in the amount of force needed to maintain a tissue at a set amount of displacement or deformation over time; creep response, which is the ability of a tissue to deform over time while a constant load is imposed; and hysteresis, which is the amount of relaxation a tissue has undergone during deformation and displacement. Injury results when the mechanical and physical limitations of connective tissue are exceeded.62
Maturation Remodeling Phase
The maturation remodeling phase of healing is a long-term process (Figure 2-2D). This phase features a realignment or remodeling of the collagen fibers that make up scar tissue according to the tensile forces to which that scar is subjected. Ongoing breakdown and synthesis of collagen occur with a steady increase in the tensile strength of the scar matrix. With increased stress and strain, the collagen fibers realign in a position of maximum efficiency parallel to the lines of tension.7 The tissue gradually assumes normal appearance and function, although a scar is rarely as strong as the normal injured tissue. Usually by the end of about 3 weeks, a firm, strong, contracted, nonvascular scar exists. The maturation phase of healing might require several years to be totally complete.
Role of Progressive Controlled Mobility During the Healing Process
Wolff’s law states that bone and soft tissue will respond to the physical demands placed on them, causing them to remodel or realign along lines of tensile force.86 Consequently, it is critical that injured structures be exposed to progressively increasing loads throughout the rehabilitative process.72
In animal models, controlled mobilization is superior to immobilization for scar formation, revascularization, muscle regeneration, and reorientation of muscle fibers and tensile properties.89 However, a brief period of immobilization of the injured tissue during the inflammatory response phase is recommended and will likely facilitate the process of healing by controlling inflammation, thus reducing clinical symptoms. As healing progresses to the repair phase, controlled activity directed toward return to normal flexibility and strength should be combined with protective support or bracing.44 Generally, clinical signs and symptoms disappear at the end of this phase.
As the remodeling phase begins, aggressive active range of motion (ROM) and strengthening exercises should be incorporated to facilitate tissue remodeling and realignment. To a great extent, pain will dictate the rate of progression. With initial injury, pain is intense; it tends to decrease and eventually subside altogether as healing progresses. Any exacerbation of pain, swelling, or other clinical symptoms during or after a particular exercise or activity indicate that the load is too great for the level of tissue repair or remodeling. The therapist must be aware of the time required for the healing process and realize that being overly aggressive can interfere with that process.
Clinical Decision-Making Exercise 2-4
A track athlete is recovering from a grade 1 ankle sprain. Beginning exercises as soon as possible will increase the injured runner’s chances of recovering quickly and strongly. Why is this so?
Factors That Impede Healing
Extent of Injury
The nature of the inflammatory response is determined by the extent of the tissue injury. Microtears or soft tissue involve only minor damage and are most often associated with overuse. Macrotears involve significantly greater destruction of soft tissue and result in clinical symptoms and functional alterations. Macrotears are generally caused by acute trauma.77
Edema
The increased pressure caused by swelling retards the healing process, causes separation of tissues, inhibits neuromuscular control, produces reflexive neurologic changes, and impedes nutrition in the injured part. Edema is best controlled and managed during the initial first-aid management period, as described previously.3
Hemorrhage
Bleeding occurs with even the smallest amount of damage to the capillaries. Bleeding produces the same negative effects on healing as does the accumulation of edema, and its presence produces additional tissue damage and, thus, exacerbation of the injury.37
Poor Vascular Supply
Injuries to tissues with a poor vascular supply heal poorly and at a slow rate. This response is likely related to a failure in the initial delivery of phagocytic cells and fibroblasts necessary for scar formation.37
Separation of Tissue
Mechanical separation of tissue can significantly impact the course of healing. A wound that has smooth edges in good apposition will tend to heal by primary intention with minimal scarring. Conversely, a wound that has jagged, separated edges must heal by secondary intention, with granulation tissue filling the defect, and excessive scarring.
Muscle Spasm
Muscle spasm causes traction on the torn tissue, separates the 2 ends, and prevents approximation. Local and generalized ischemia can result from spasm.
Atrophy
Wasting away of muscle tissue begins immediately with injury. Strengthening and early mobilization of the injured structure retard atrophy.
Corticosteroids
Use of corticosteroids in the treatment of inflammation is controversial. Steroid use in the early stages of healing has been demonstrated to inhibit fibroplasia, capillary proliferation, collagen synthesis, and increases in tensile strength of the healing scar. Their use in the later stages of healing and with chronic inflammation is debatable.3
Keloids and Hypertrophic Scars
Keloids occur when the rate of collagen production exceeds the rate of collagen breakdown during the maturation phase of healing. This process leads to hypertrophy of scar tissue, particularly around the periphery of the wound.
Infection
The presence of bacteria in the wound can delay healing, causes excessive granulation tissue, and frequently causes large, deformed scars.16
Humidity, Climate, and Oxygen Tension
Humidity significantly influences the process of epithelialization. Occlusive dressing stimulates the epithelium to migrate twice as fast without crust or scab formation. The formation of a scab occurs with dehydration of the wound and traps wound drainage, which promotes infection. Keeping the wound moist provides an advantage for the necrotic debris to go to the surface and be shed.
Oxygen tension relates to the neovascularization of the wound, which translates into optimal saturation and maximal tensile strength development. Circulation to the wound can be affected by ischemia, venous stasis, hematomas, and vessel trauma.
Health, Age, and Nutrition
The elastic qualities of the skin decrease with age. Degenerative diseases, such as diabetes and arteriosclerosis, also become a concern of the older patient and can affect wound healing. It has been shown that malnutrition negatively affects the healing process.75 A patient who is undernourished or malnourished prolongs the inflammatory response phase and thus delays the fibroblastic phase by decreasing the proliferation of fibroblasts and subsequently the formation of collagen, thus reducing tensile strength of the healing wound. It can also increase the risk for infection by decreasing T-cell function and phagocytic activity.75
THE IMPORTANCE OF NUTRITION TO THE HEALING PROCESS
It appears that nutrition plays a critical role in the healing process regardless of the type of tissue that is injured.75 Healing of injuries to any of the body’s tissues requires optimal intake of various nutrients, which collectively provide the energy necessary to fuel the healing process. Nutrients have 3 major functions: production of energy; growth, repair, and maintenance of all body tissues; and regulation of body processes.33 Nutrients are categorized into 6 major classes: carbohydrates, fats (also called lipids), proteins, water, vitamins, and minerals. Carbohydrates, proteins, and fats are referred to as the macronutrients, the absorbable components of food from which energy is derived.62 Multiple factors, both individually and collectively, dictate energy demands, including age, gender, activity level, basal metabolic rate, nutritional status, body mass index, stress of illness, severity, size and number of wounds, and stage in the healing process.85
Carbohydrates are the body’s most efficient immediate source of energy. During digestion, carbohydrates are easily broken down and converted to glucose. The glucose that is not needed to provide immediate energy is stored as glycogen in the liver and muscle cells. A supply of glucose must be kept available to prevent the use of protein for energy. This is called the protein-sparing action of glucose.
Like carbohydrates, fats are a primary source of energy. Fats are the most concentrated source of energy, providing more than twice the calories per gram when compared with carbohydrates or proteins.62 However, the role of fat in wound healing is not clear, but it is well accepted that with injury, there is an increased need for essential fatty acids. For example, ω-3 fatty acids have anti-inflammatory actions, improve immune function, and reduce infection rates.75
Figure 2-3. General anatomy of a synovial joint. (Reprinted with permission from Saladin K. Anatomy & Physiology. 6th ed. New York, NY: McGraw-Hill; 2014.)67
Proteins play the most critical role throughout the whole healing process.85 Proteins make up the major structural components of the body. They are needed for the growth, maintenance, and repair of all body tissues. The cells that make up the immune system, including leukocytes, macrophages, lymphocytes, monocytes, and phagocytic cells, are composed primarily of proteins. These cells each play an essential role during the inflammatory response phase, which initiates the healing process. Protein is also necessary for the synthesis of enzymes involved in wound healing, many hormones, antibodies that help fight infection, and proliferation of connective tissue cells and collagen.75
Vitamins, minerals, and water are considered to be micronutrients, which are necessary for regulating normal body functions.62 They do not provide energy, but without sufficient quantities of micro nutrients, the energy from macronutrients cannot be utilized. Vitamin A stimulates the immune system by increasing the number of macrophages and monocytes in a wound during inflammation. Both Vitamins A and C enhance wound healing by stimulating epithelialization and increasing collagen deposition by fibroblasts. Vitamin C also enhances capillary formation and neutrophil activity.75,85
Magnesium, copper, and zinc are minerals, each of which contributes to tissue healing. Magnesium is a cofactor for enzymes necessary for protein and collagen formation and tissue growth during wound healing. Copper is important in the formation of hemoglobin. Zinc is essential in numerous aspects of cellular metabolism including immune function, DNA synthesis, and protein and collagen synthesis.75
Water is the most essential of all the nutrients. Ensuring adequate water intake is necessary for perfusion and oxygenation of healthy and healing tissues. Prevention and treatment of skin breakdown requires optimal fluid intake.75
INJURIES TO ARTICULAR STRUCTURES
Before discussing injuries to the various articular structures, a review of joint structure is in order48 (Figure 2-3). All synovial joints are composed of 2 or more bones that articulate with one another to allow motion in one or more places. The articulating surfaces of the bone are lined with a very thin, smooth, cartilaginous covering called a hyaline cartilage. All joints are entirely surrounded by a thick, ligamentous joint capsule. The inner surface of this joint capsule is lined by a very thin synovial membrane that is highly vascularized and innervated. The synovial membrane produces synovial fluid, the functions of which include lubrication, shock absorption, and nutrition of the joint.48
Figure 2-4. Grade 3 ligament sprain in the knee joint.
Some joints contain a thick fibrocartilage called a meniscus. The knee joint, for example, contains 2 wedge-shaped menisci that deepen the articulation and provide shock absorption in that joint. Finally, the main structural support and joint stability is provided by the ligaments, which may be either thickened portions of a joint capsule or totally separate bands.
Ligament Sprains
Ligaments are composed of dense connective tissue arranged in parallel bundles of collagen composed of rows of fibroblasts. Although bundles are arranged in parallel, not all collagen fibers are arranged in parallel. Ligaments and tendons are very similar in structure. However, ligaments are usually more flattened than tendons, and collagen fibers in ligaments are more compact. The anatomical positioning of the ligaments determines in part what motions a joint can make.
A sprain involves damage to a ligament that provides support to a joint. A ligament is a tough, relatively inelastic band of tissue that connects one bone to another. A ligament’s primary function is 3-fold: to provide stability to a joint, to provide control of the position of one articulating bone to another during normal joint motion, and to provide proprioceptive input or a sense of joint position through the function of free nerve endings or mechanoreceptors located within the ligament.62
If stress is applied to a joint that forces motion beyond its normal limits or planes of movement, injury to the ligament is likely (Figure 2-4). The severity of damage to the ligament is classified in many different ways; however, the most commonly used system involves 3 grades (degrees) of ligamentous sprain:
- Grade 1 sprain: There is some stretching or perhaps tearing of the ligamentous fibers, with little or no joint instability. Mild pain, little swelling, and joint stiffness might be apparent.
- Grade 2 sprain: There is some tearing and separation of the ligamentous fibers and moderate instability of the joint. Moderate-to-severe pain, swelling, and joint stiffness should be expected.
Clinical Decision-Making Exercise 2-5
A basketball player twisted his ankle today in practice. The mechanism and the location of pain suggest an inversion sprain. There is gross laxity with the anterior drawer test and talar tilt test. The swelling is severe and profuse over the lateral side of the ankle. The athlete is incapable of dorsiflexion and has only a few degrees of plantar flexion. He is experiencing very little pain. How would you grade the severity of this injury?
- Grade 3 sprain: There is total rupture of the ligament, manifested primarily by gross instability of the joint. Severe pain might be present initially, followed by little or no pain because of total disruption of nerve fibers. Swelling might be profuse, and thus the joint tends to become very stiff some hours after the injury. A third-degree sprain with marked instability usually requires some form of immobilization lasting several weeks. Frequently, the force producing the ligament injury is so great that other ligaments or structures surrounding the joint are also injured. With cases in which there is injury to multiple joint structures, surgical repair reconstruction may be necessary to correct an instability.
Clinical Decision-Making Exercise 2-6
Why is it likely that an athlete with a grade 3 ligament sprain initially will experience little pain, relative to the severity of the injury?
Physiology of Ligament Healing
The healing process in the sprained ligament follows the same course of repair as with other vascular tissues. Immediately after injury and for about 72 hours, there is a loss of blood from damaged vessels and attraction of inflammatory cells into the injured area. If a ligament is sprained outside of a joint capsule (extra-articular ligament), bleeding occurs in a subcutaneous space. If an intra-articular ligament is injured, bleeding occurs inside of the joint capsule until either clotting occurs or the pressure becomes so great that bleeding ceases.
During the next 6 weeks, vascular proliferation with new capillary growth begins to occur along with fibroblastic activity, resulting in the formation of a fibrin clot. It is essential that the torn ends of the ligament be reconnected by bridging this clot. Synthesis of collagen and ground substance of proteoglycan as constituents of an intracellular matrix contributes to the proliferation of the scar that bridges between the torn ends of the ligament. This scar initially is soft and viscous, but it eventually becomes more elastic. Collagen fibers are arranged in a random woven pattern with little organization. Gradually, there is a decrease in fibroblastic activity, a decrease in vascularity, and an increase to a maximum in collagen density of the scar.36 Failure to produce enough scar and failure to reconnect the ligament to the appropriate location on a bone are the 2 reasons why ligaments are likely to fail.
During the next several months, the scar continues to mature, with the realignment of collagen occurring in response to progressive stresses and strains. The maturation of the scar may require as long as 12 months to complete.36 The exact length of time required for maturation depends on mechanical factors such as apposition of torn ends and length of the period of immobilization.
Factors Affecting Ligament Healing
Surgically repaired extra-articular ligaments have healed with decreased scar formation and are generally stronger than unrepaired ligaments initially, although this strength advantage might not be maintained as time progresses. Unrepaired ligaments heal by fibrous scarring effectively lengthening the ligament and producing some degree of joint instability. With intra-articular ligament tears, the presence of synovial fluid dilutes the hematoma, thus preventing formation of a fibrin clot and spontaneous healing.38
Data from several studies indicate that actively exercised ligaments are stronger than those that are immobilized. Ligaments that are immobilized for several weeks after injury tend to decrease in tensile strength and also exhibit weakening of the insertion of the ligament to bone.62 Thus, it is important to minimize periods of immobilization and progressively stress the injured ligaments while exercising caution relative to biomechanical considerations for specific ligaments.36
It is not likely that the inherent stability of the joint provided by the ligament before injury will be regained. Thus, to restore stability to the joint, the other structures that surround that joint (primarily muscles and their tendons) must be strengthened. The increased muscle tension provided by resistance training can improve stability, neuromuscular control, and function of the injured joint.6,36
Cartilage Damage
Cartilage is a type of rigid connective tissue that provides support and acts as a framework in many structures. It is composed of chondrocyte cells contained in small chambers called lacunae, surrounded completely by an intracellular matrix. The matrix consists of varying ratios of collagen and elastin and a ground substance made of proteoglycans and glycosaminoglycans, which are nonfibrous protein molecules. These proteoglycans act as sponges and trap large quantities of water, which allow cartilage to spring back after being compressed.31 Cartilage has a poor blood supply, thus healing after injury is very slow. There are 3 types of cartilage. Hyaline cartilage is found on the articulating surfaces of bone and in the soft part of the nose. It contains large quantities of collagen and proteoglycan. Fibrocartilage forms the intervertebral disc and menisci located in several joint spaces. It has greater amounts of collagen than proteoglycan and is capable of withstanding a great deal of pressure. Elastic cartilage is found in the auricle of the ear and the larynx. It is more flexible than the other types of cartilage and consists of collagen, proteoglycan, and elastin.67
Figure 2-5. Osteoarthritis is a degeneration and erosion of the hyaline cartilage.
Osteoarthrosis is a degenerative condition of bone and cartilage in and about the joint (Figure 2-5). Arthritis should be defined as primarily an inflammatory condition with possible destruction.68 Arthrosis is primarily a degenerative process with destruction of cartilage, remodeling of bone, and possible secondary inflammatory components.
Cartilage fibrillates, that is, releases fibers or groups of fibers and ground substance into the joint.32 Peripheral cartilage that is not exposed to weightbearing or compression–decompression mechanisms is particularly likely to fibrillate. Fibrillation is typically found in the degenerative process associated with poor nutrition or disuse. This process can then extend even to weightbearing areas, with progressive destruction of cartilage proportional to stresses applied on it. When forces are increased, thus increasing stress, osteochondral or subchondral fractures can occur. Concentration of stress on small areas can produce pressures that overwhelm the tissue’s capabilities. Typically, lower limb joints have to handle greater stresses, but their surface area is usually larger than the surface area of upper limbs. The articular cartilage is protected to some extent by the synovial fluid, which acts as a lubricant. It is also protected by the subchondral bone, which responds to stresses in an elastic fashion. It is more compliant than compact bone, and microfractures can be a means of force absorption. Trabeculae might fracture or might be displaced due to pressures applied on the subchondral bone. In compact bone, fracture can be a means of defense to dissipate force. In the joint, forces might be absorbed by joint movement and eccentric contraction of muscles.28
In the majority of joints where the surfaces are not congruent, the applied forces tend to concentrate in certain areas, which increases joint degeneration. Osteophytosis occurs as a bone attempts to increase its surface area to decrease contact forces. People typically describe this growth as “bone spurs.” Chondromalacia is the nonprogressive transformation of cartilage with irregular surfaces and areas of softening. It typically occurs first in nonweightbearing areas and may progress to areas of excessive stress.27
In physically active individuals, certain joints may be more susceptible to a response resembling osteoarthrosis.30 The proportion of body weight resting on the joint, the pull of the musculotendinous unit, and any significant external force applied to the joint are predisposing factors. Altered joint mechanics caused by laxity or previous trauma are also factors that come into play.50 The intensity of forces can be great, as in the hip, where the previously mentioned factors can produce pressures or forces 4 times that of body weight and up to 10 times that of body weight on the knee.
Typically, muscle forces generate more stress than body weight itself. Particular injuries are conducive to osteoarthritic changes such as subluxation and dislocation of the patella, osteochondritis dissecans, recurrent synovial effusion, and hemarthrosis. Also, ligamentous injuries can bring about a disruption of proprioceptive mechanisms, loss of adequate joint alignment, and meniscal damage in the knees with removal of the injured meniscus.54 Other factors that have an impact are loss of full ROM, poor muscular power and strength, and altered biomechanics of the joint. Spurring and spiking of bone are not synonymous with osteoarthrosis if the joint space is maintained and the cartilage lining is intact. It may simply be an adaptation to the increased stress of physical activity.32
Physiology of Cartilage Healing
Cartilage has a relatively limited healing capacity. When chondrocytes are destroyed and the matrix is disrupted, the course of healing is variable, depending on whether damage is to cartilage alone or also to subchondral bone. Injuries to the articular cartilage alone fail to elicit clot formation or a cellular response. For the most part, the chondrocytes adjacent to the injury are the only cells that show any signs of proliferation and synthesis of matrix. Thus, the defect fails to heal, although the extent of the damage tends to remain the same.34,40
If subchondral bone is also affected, inflammatory cells enter the damaged area and formulate granulation tissue. In this case, the healing process proceeds normally, with differentiation of granulation tissue cells into chondrocytes occurring in about 2 weeks. At about 2 months, normal collagen has been formed.
Injuries to the knee articular cartilage are extremely common, and until recently, methods for treatment did not produce good long-term outcomes.12 A better understanding of how articular cartilage responds to injury has produced various techniques that hold promise for long-term success.29,76 One such technique is autologous chondrocyte implantation, in which a patient’s own cartilage cells are harvested, grown ex vivo, and reimplanted in a full-thickness articular surface defect. Results are available with up to 10 years of follow up, and more than 80% of patients have shown improvement with relatively few complications.
Figure 2-6. The gross structure of the long bones includes the diaphysis, epiphysis, articular cartilage, and periosteum. (Reprinted with permission from Saladin K. Anatomy & Physiology. 6th ed. New York, NY: McGraw-Hill; 2014.)67
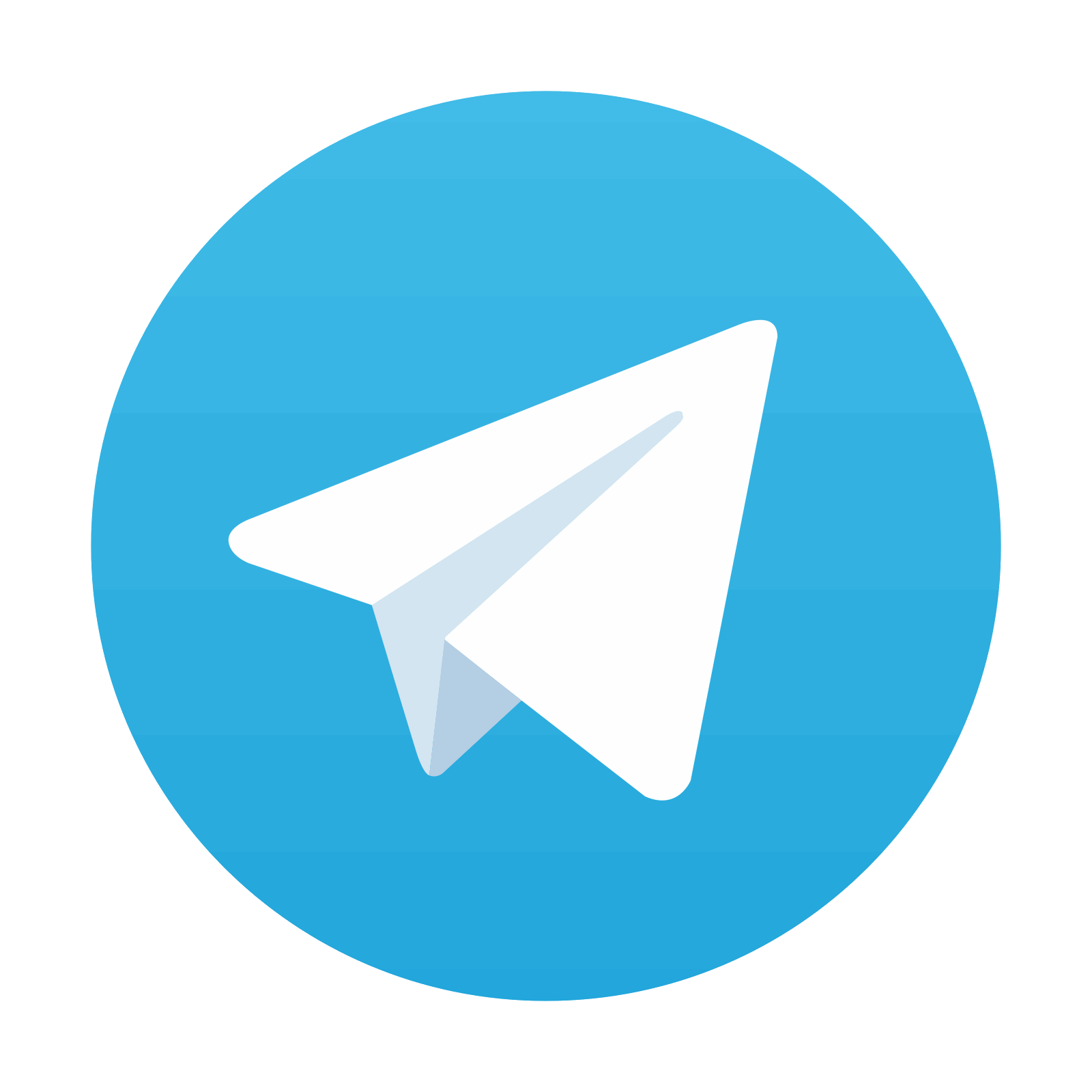
Stay updated, free articles. Join our Telegram channel

Full access? Get Clinical Tree
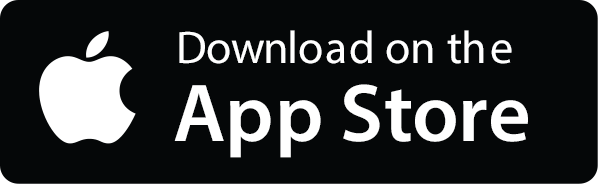
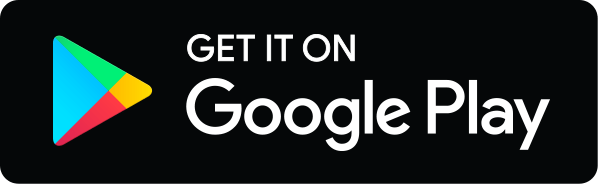