Type 1 (Insulin-Dependent) Diabetes Mellitus
Leslie P. Plotnick
Type 1 (insulin-dependent) diabetes mellitus is a common, serious disease of children and adolescents. The diagnosis usually is straightforward, but long-term management is a major challenge for the child, family, and health care team. Developments since the 1980s have rendered the attainment of metabolic control a technical possibility. However, achievement of true metabolic normalcy remains an elusive goal. In addition, diabetes management is stressful to the family, and psychological and behavioral issues often interfere with the goal of metabolic control. Few other diseases require the extensive self-care management needed to care for type 1 diabetes.
DEFINITION AND DIAGNOSTIC CRITERIA
In 1997, an expert committee reclassified and updated diagnostic criteria for diabetes. Type 1 diabetes is the type most
frequently found in children and adolescents. It has been called juvenile-onset, ketosis-prone, brittle diabetes, and, until most recently, type 1 or insulin-dependent diabetes mellitus (IDDM). Patients with type 1 diabetes are insulinopenic and need exogenous insulin to prevent development of ketosis and to preserve life.
frequently found in children and adolescents. It has been called juvenile-onset, ketosis-prone, brittle diabetes, and, until most recently, type 1 or insulin-dependent diabetes mellitus (IDDM). Patients with type 1 diabetes are insulinopenic and need exogenous insulin to prevent development of ketosis and to preserve life.
Type 2 diabetes is found more commonly in adults and obese persons. This type also has been called adult-onset, maternity-onset, ketosis-resistant, stable diabetes, and, until most recently, type 2 or non–insulin-dependent diabetes mellitus (NIDDM). As obesity increases in the pediatric and adolescent populations, type 2 diabetes is becoming increasingly common in this age group. Type 2 diabetes is discussed in detail in Chapter 379. It is caused primarily by insulin resistance without adequate compensatory insulin secretion. Thus, a relative, not absolute, insulin deficiency occurs. Affected patients are not dependent on insulin for survival and are less ketosis-prone, but they may need exogenous insulin for metabolic control, and they can develop ketosis and ketoacidosis in certain situations. Sometimes distinguishing between type 1 and type 2 is difficult at presentation.
Other specific types of diabetes occur due to causes such as genetic defects in beta-cell function (maturity-onset diabetes in youth [MODY]) and insulin action, and others are caused by diseases of the exocrine pancreas.
To be diagnosed with diabetes, a child or adolescent must have classic symptoms with a random plasma glucose level at or above 200 mg/dL (11.1 mmol/L). If the patient is asymptomatic, fasting plasma glucose must be at or above 126 mg/dL (7.0 mmol/L) or the 2-hour plasma glucose levels on an oral glucose tolerance test must be at or above 200 mg/dL. The asymptomatic criteria require confirmation by repeat testing (on another day). The diagnosis of diabetes in a child rarely is subtle. Most children present with the classic symptoms of polyuria, polydipsia, polyphagia, weight loss, and lethargy. Glucose tolerance testing rarely is necessary for establishing the diagnosis.
EPIDEMIOLOGY
The prevalence of type 1 diabetes in the United States in children and adolescents varies somewhat according to different sources, with most studies reporting a rate of 1.2 to 1.9 cases per 1,000 members of the population of this age group. This incidence is age-dependent with a range of fewer than 1 per 1,000 at age 5 years to approximately 3 per 1,000 at age 16 years. The U.S. incidence is about 12 to 15 new cases per year per 100,000 of the pediatric population.
Major worldwide variations in incidence exist, with the United States having an intermediate incidence. Incidence increases with age and peaks in early to middle puberty. A seasonal distribution of newly diagnosed cases exists: for unknown reasons, more cases are diagnosed in the cooler months. Male and female subjects are approximately equally affected.
GENETICS, ETIOLOGY, AND PATHOGENESIS
Our understanding of the genetics, cause, and pathogenesis of type 1 diabetes has increased greatly. No one diabetes gene exists. Instead, certain genetic alterations raise or lower the risk of beta-cell damage. Inheritance of HLA antigens DR3 and DR4 is associated with an increased risk of developing type 1 diabetes. Inheritance of one of these HLA antigens confers a threeto fivefold risk of developing type 1 diabetes; inheritance of both confers an approximately tenfold risk. DR3 or DR4 occurs in approximately 95% of persons with type 1 diabetes. Variations on the DQ antigens also account for changes in the risk of developing type 1 diabetes. An amino acid variation at position 57 of the DQ beta chain (nonaspartic acid) is associated with a marked increase in risk. In addition, a higher risk is conferred by an arginine at position 52 of the DQ alpha chain.
BOX 378.1 Risk of Developing Type 1 Diabetes if One Sibling is Affected
Identical twins: <50%
HLA identical: 1 in 5
HLA haploidentical: 1 in 20
HLA nonidentical: 1 in 100
The chance of type 1 diabetes developing in a second sibling is summarized in Box 378.1 and is related directly to the shared HLA haplotypes. Approximately 5% of a diabetic patient’s siblings develop type 1 diabetes.
Less than 50% of identical twins are concordant for type 1 diabetes, indicating that a susceptibility to type 1 diabetes, rather than the disease itself, is inherited. This inherited susceptibility appears to place the beta cell at unusual risk for immunologic, inflammatory damage. The less than 50% concordance in identical twins and the marked geographic variation in incidence of type 1 diabetes support the significance of external factors in the process of beta-cell damage.
Beta-cell destruction occurs in genetically susceptible people. Researchers suggest that at least 80% of the functional beta-cell mass must be destroyed before overt glucose intolerance occurs. The process of beta-cell destruction in most cases is immune-mediated, probably takes months or years, and may be initiated by environmental factors. Whether the process is relentless and progressive, inevitably producing type 1 diabetes, or may wax and wane over the course of time and sometimes enters remission remains controversial. One hypothesis is that the exposure to the environmental factors may be recurrent and intermittent or continuous (e.g., viral infections, dietary factors, environmental toxins, stress) rather than a single triggering event. Viruses or other environmental factors may precipitate beta-cell destruction by direct damage (exposing antigens for future immunologic attack) or by molecular mimicry caused by similar protein sequences in beta-cell antigens and the offending agent (e.g., virus, bovine serum albumin). The damaged beta cell presents antigens recognized as nonself to the immune system. The ability of macrophages to present these antigens to helper T cells depends on the class II (D) HLA molecules and their three-dimensional structure, which is determined by the amino acid sequence of the HLA molecules’ alpha and beta chains. Another hypothesis suggests that certain HLA types may bind the antigens intracellularly, preventing their exposure to the immune system and protecting against the development of type 1 diabetes.
The autoimmune processes release cytokines that are destructive in the beta cells directly or that destroy tissue by generating free radicals. The process of beta-cell destruction probably occurs in two phases: an early cytokine-dependent initiation phase and a later phase with antigen-specific T-lymphocyte proliferation, which amplifies and perpetuates beta-cell destruction. Beta-cell protective mechanisms may help beta cells resist immunologic damage.
Type 1 diabetes susceptibility probably is conferred by unfavorable combinations of common gene alleles for HLA and beta-cell destructive and beta-cell protective mechanisms.
Specific HLA types appear to be necessary for development of type 1 diabetes, but they are not sufficient.
Specific HLA types appear to be necessary for development of type 1 diabetes, but they are not sufficient.
Most patients with newly diagnosed type 1 diabetes have measurable immunologic markers: islet cell antibodies (ICAs) and insulin autoantibodies (IAAs). ICAs may be cytoplasmic or surface antibodies. High-titer ICAs confer a greater risk of subsequently developing type 1 diabetes than do low titers. ICAs probably are more predictive in children, as is the persistence of positivity.
Another important immunologic marker is IAAs. IAAs in combination with ICAs and high-titer IAAs are associated with an increased risk of developing type 1 diabetes. Other beta-cell autoantigens, including glutamic acid decarboxylase (GAD65), also are important markers.
PATHOPHYSIOLOGY
Insulin is the body’s major anabolic hormone. In the fed state, it stimulates energy storage in the forms of glycogen, protein, and adipose tissue. When insulin levels are low or deficient, mobilization of stored substrate occurs (i.e., glycogenolysis, proteolysis, lipolysis) and tissue uptake of glucose is inhibited. Because insulin is a potent antilipolytic hormone, a greater degree of insulin deficiency is required for lipolysis than for glucose intolerance to occur. In the early stages of insulin deficiency, hyperglycemia predominates, and a more severe degree of insulin deficiency is necessary for ketonuria, ketonemia, and acidosis to develop.
In addition to insulin deficiency, a relative excess of counterregulatory hormones (e.g., growth hormone, cortisol, glucagon, catecholamines) must exist to produce the picture of diabetic ketoacidosis (DKA). Hyperglycemia produces an osmotic diuresis, causing the symptoms of polyuria and polydipsia. Passive electrolyte loss occurs along with the osmotic diuresis. Weight loss is caused by the general catabolic state and the osmotic diuresis. Eventually, dehydration results and is especially severe if the child cannot drink enough fluid to compensate for the diuresis, as in the case of vomiting or a decreased level of consciousness. Lipolysis results in production of ketones, causing metabolic acidosis.
CLINICAL PRESENTATION
Most children with type 1 diabetes present with the classic symptoms of polyuria, polydipsia, polyphagia, and weight loss; many also complain of lethargy. If the diagnosis is not made and treatment is not begun, further metabolic decompensation occurs, with worsening DKA.
In young children and infants, the diagnosis is more likely to be missed in its early stages because of difficulty in recognizing early symptoms, and children of these ages are more likely to present with severe ketoacidosis. If pediatricians inquire specifically about the classic symptoms in patients with nonspecific signs of illness and weight loss, this diagnosis is more likely to be made quickly. Establishing the diagnosis early avoids further metabolic decompensation and the risks of DKA and its treatment.
Most children with new-onset type 1 diabetes have symptoms of less than 1 month’s duration, but some have had mild to moderate symptoms for several months. Questions about bedwetting, nocturia, number of diapers used, or leaving class to use the bathroom may help uncover the presence of polyuria.
Type 1 diabetes must be considered in any child with clinical dehydration who continues to urinate regularly. Too often, frequent urination leads the parent or physician to conclude incorrectly that the child is not dehydrated. Routine dipstick testing for urine glucose and ketones in patients with nonspecific symptoms such as lethargy, weight loss, nausea, and vomiting and in those with specific type 1 diabetes symptoms could enhance greatly the early establishment of diagnosis of this disease before severe metabolic decompensation has occurred.
New-onset type 1 diabetes may be managed in an inpatient or outpatient setting, depending on the severity of the patient’s metabolic abnormalities and the health care resources available.
CLINICAL COURSE
After the initial presentation, many children with newly diagnosed type 1 diabetes undergo a honeymoon period or remission phase. During this period, the remaining functional beta cells regain the ability to produce insulin, possibly as a result of elimination of hyperglycemia. Measurement of C-peptide levels has demonstrated that improved secretion of insulin occurs during this phase. Because endogenous insulin secretion increases, requirements for exogenous insulin decrease, usually dropping to less than 0.5 U/kg per day. Hypoglycemia becomes a potential problem. This phase usually begins within 1 to 3 months after diagnosis is made and lasts for several months, sometimes as long as 12 to 24 months. The honeymoon period is a period of relative well-being, with metabolic near normalcy as indicated by normal glycosylated hemoglobin levels. Information about the honeymoon period must be included in the education of the newly diagnosed patient because denial of having the disease and subsequent failure to monitor it are likely to occur unless patients and families learn about this phase and expect its occurrence and its end.
As this phase ends, the remaining beta cells lose their capacity to secrete insulin, and requirements for exogenous insulin increase. This phase usually occurs gradually, but, as in cases of acute infection, it may be abrupt. Careful monitoring and frequent adjustments of doses are extremely important during the end of the remission phase, and close contact between the patient and physician is necessary.
GOALS OF TREATMENT
The overall goals of treatment of type 1 diabetes center around the (currently unattainable) goal of true metabolic normalcy, which in day-to-day life means avoiding short-term complications (hypoglycemia, hyperglycemia, ketosis, and DKA) and minimizing risks of developing long-term complications. In addition, helping the child and family achieve normal psychological maturation and independence is an ongoing and lifelong goal. Certain specific goals are discussed below.
Normal Growth
Normal growth in height and weight and normal timing of adolescent pubertal development are important goals of long-term management. Chronically undertreated children with poorly controlled type 1 diabetes often fail to grow and gain weight normally and have delayed skeletal maturation (i.e., bone age) and delayed sexual maturation. The growth retardation can be severe. Growth is an important factor to follow, and height, weight, and pubertal development should be monitored carefully. The causes for deviations from normal velocities should be sought.
Children receiving excessive doses of insulin may gain weight too rapidly. Excessive doses of insulin, which can cause
rebound hyperglycemia and ketosis, can produce on occasion a similar degree of growth retardation, as can chronically inadequate doses. Mauriac syndrome (diabetes with growth retardation and hepatomegaly) is caused by poor diabetic control, and, although patients with this syndrome usually are receiving inadequate insulin doses, excessive doses on occasion have been associated with this clinical picture.
rebound hyperglycemia and ketosis, can produce on occasion a similar degree of growth retardation, as can chronically inadequate doses. Mauriac syndrome (diabetes with growth retardation and hepatomegaly) is caused by poor diabetic control, and, although patients with this syndrome usually are receiving inadequate insulin doses, excessive doses on occasion have been associated with this clinical picture.
Effective Management Choices
Education of the patient and family with the goal of independent management of type 1 diabetes at home is important. Some families achieve independence quickly. Others require intensive and repeated education on a one-to-one basis or in group programs. Independent decision making by patients and families who are well educated about diabetes and its management enhances independence, feelings of control, and self-esteem and is important for long-term psychological success. Most day-to-day decisions regarding hyperglycemia, hypoglycemia, illness, ketonuria, and unusual activities or eating schedules can be handled appropriately by knowledgeable families. Frequent blood glucose monitoring, which is discussed later in this chapter, is mandatory.
Children and adolescents with type 1 diabetes should be encouraged to participate in any activities that are appropriate for their ages and interests. An adolescent with sports practice three times a week after school can learn how to increase calories or decrease insulin doses and keep blood glucose levels in an acceptable range during the activity. Certain precautions must be taken. For example, a source of sugar must be readily available during a physical activity. When adolescents drive, a readily available glucose source must be in easy and rapid reach. Medic-Alert bracelets or necklaces should be worn.
The choice of insulin regimen, including lifestyle choices, education level, and motivation to do the work needed for diabetes management, should meet the needs of the individual child and family.
The diet should be designed around the child’s and family’s food preferences and habits, using sound nutritional principles. Families should participate in the planning of the diet, and the amount of food must be adequate for satiety to maximize adherence. With basal/bolus insulin regimens, discussed below, dietary flexibility is the norm.
Avoiding Metabolic Abnormalities
Avoidance of metabolic abnormalities is another important goal. Monitoring blood glucose levels several times a day along with urinary ketone checks is mandatory for successful management. Significant hyperglycemia and hypoglycemia should be avoided. In young children, especially preschoolers, blood glucose levels often vary widely. To avoid development of serious hypoglycemia in this age group, compromises may have to be made in tolerating hyperglycemia. Glycosylated hemoglobin levels should be monitored regularly, usually every 3 months, with a goal of achieving a level as close to the normal range as possible. Normal values vary depending on the laboratory method used. Blood lipids should be monitored and dietary modifications made if hyperlipidemia occurs.
Ketonuria should be treated early. In most patients who monitor regularly, DKA can be avoided by responding to hyperglycemia, ketosis, and periods of illness with adjustments in insulin doses. The demands of the diabetes management regimen are high and require care and understanding on the part of the families and the health care team to maximize the child’s chances for successful emotional development.
MANAGEMENT
Insulin
Many insulin preparations, including standard insulin and new “designer” insulin analogues, are available. Animal (beef and pork) insulin no longer is available; all insulin now is human, made by recombinant DNA methods.
Three rapid-acting insulin analogues are available. The first, lispro (Humalog, Eli Lilly), was approved for use in 1996. Aspart (NovoLog, Novo-Nordisk), and glulisine (Apidra Snofi-Aventis) were approved more recently. These are biosynthetic analogues of regular insulin and are absorbed and cleared more rapidly than is regular insulin and, therefore, more closely mimic pancreatic insulin secretion. They can be used effectively even after a meal in children with unpredictable eating habits.
Regular insulin is short-acting and is the standard insulin used in intravenous infusion to treat DKA. Neutral protamine hagedorn (NPH) or Lente is intermediate in peak and duration of action. Ultralente is a longer-acting insulin than is NPH, with a duration of approximately 18 to 22 hours, and has a significant peak. Glargine (Lantus, Aventis) is an insulin analogue that is the first peakless long-acting insulin, with a duration of action of approximately 20 to 24+ hours (Table 378.1). Levemir (Detemir, Novo Nordisk) is a long acting insulin given 1 to 2 times per day.
With the advent of insulin analogues, many options for insulin regimens now are possible.
Split/Mixed Regimens
These regimens are the basic two or three injections using an intermediate insulin (e.g., NPH) or a longer-acting one (e.g., Ultralente). Most children and adolescents require at least two injections per day of shortand intermediate-acting insulin to achieve satisfactory metabolic control; the injections are administered shortly before breakfast and dinner. During the honeymoon period, when insulin requirements are at a minimum, one injection per day may be satisfactory for control. Except for this period, achieving control with a single daily injection is nearly impossible. Absorption may vary from different injection sites and is more rapid in exercised sites and at higher temperatures. Injection into hypertrophied sites may slow absorption.
These regimens use, for example, NPH and regular insulin at breakfast and again at dinner. The total dose is split into two shots, and each shot is a mix of NPH and regular insulin. A rapid-acting analogue can be used instead of regular insulin.
A variation of this schedule is to split the evening dose further into regular insulin or a rapid-acting analogue taken with dinner and move the time for taking the NPH to bedtime. The peak actions of the insulin used in split/mixed regimens do not correlate well with usual mealtimes, and excess of between-meal insulin levels causes a need for snacks to avoid development of hypoglycemia. There is an increased risk of development of nocturnal hypoglycemia. Use of a rapid-acting insulin instead of regular insulin helps somewhat with this problem, but the peaks of NPH and Ultralente remain nonphysiologic (Box 378.2).
A variation of this schedule is to split the evening dose further into regular insulin or a rapid-acting analogue taken with dinner and move the time for taking the NPH to bedtime. The peak actions of the insulin used in split/mixed regimens do not correlate well with usual mealtimes, and excess of between-meal insulin levels causes a need for snacks to avoid development of hypoglycemia. There is an increased risk of development of nocturnal hypoglycemia. Use of a rapid-acting insulin instead of regular insulin helps somewhat with this problem, but the peaks of NPH and Ultralente remain nonphysiologic (Box 378.2).
TABLE 378.1. TIMING OF ACTION OF AVAILABLE INSULIN (TIMES ARE APPROXIMATE) | ||||||||||||||||||||||||||||||||||||||||
---|---|---|---|---|---|---|---|---|---|---|---|---|---|---|---|---|---|---|---|---|---|---|---|---|---|---|---|---|---|---|---|---|---|---|---|---|---|---|---|---|
|
BOX 378.2 Example of Split/Mixed Insulin Regimen for a 30-kg Child on a Diet with a Fixed Number of Carbohydrates per Meal
Morning (before breakfast): 12 NPH
Before dinner or at bedtime: 6 NPH
Sliding scale regular, lispro or aspart dose
Basal/Bolus Regimens
These regimens are attempts to achieve more physiologic insulin levels. One insulin type is given to provide basal (or fasting) insulin needs, and another insulin type is used to cover “bolus” needs (food requirements). Bolus doses are determined based on the amount and type of food ingested (mainly carbohydrates) and on the blood glucose level at the time the meal is eaten. Basal insulin can be provided either with the basal rate on an insulin pump or with glargine. Bolus doses are provided by use of rapid-acting analogues. Basal requirements usually are approximately 50% of total daily insulin needs.
No matter which regimen is used, frequent blood glucose monitoring is necessary so that patients can respond to the levels by adjusting their insulin doses. For example, with a split/mixed regimen, if an occasional fasting blood glucose level falls above the target range, the morning short-acting insulin dose should be increased. If the fasting blood glucose level is increased for several consecutive days, the evening NPH dose should be increased.
During the honeymoon period, dose requirements may drop to less than 0.5 U/kg/day. Except during this period, most preadolescent children need approximately 0.75 to 1.0 U/kg/day. Teenagers usually require approximately 1.0 to 1.2 U/kg/day.
Patients on a split/mixed regimen typically need approximately two-thirds of the total dose in the morning and one-third before eating dinner. The doses usually are split between one-third shortor rapid-acting (regular, lispro, or aspart) and two-thirds NPH to one-half shortor rapid-acting and one-half NPH. More shortor rapid-acting insulin usually is required in the morning because of the dawn phenomenon, which is caused by normal nocturnal increases in some counterregulatory hormones, producing less insulin sensitivity in the early morning.
BOX 378.3 Example of a Basal Bolus Regimen for a 40-kg Child on a Diet with no Fixed Number of Carbohydrates
Total daily insulin dose = 32 units
50% basal = 16 units for basal insulin given as either 16 units glargine per day OR 0.6 units per hour of lispro or aspart as pump basal rate
Bolus doses: Insulin-to-carbohydrate ratio for meals and snacks: 450 divided by total insulin dose (450/32 = 14). Use 1 unit per 15 carbohydrate grams.
Correction factor: 1,800 rule = 1,800 divided by total insulin dose (1,800/32 = 56). Use 1 unit per 60 blood glucose points (or 0.5 unit per 30 blood glucose points) above target. Target might be 120 in the daytime and 150 at night.
Use of a basal/bolus regimen requires either an insulin pump or use of daily glargine (given once or sometimes twice per day) and doses of lispro or aspart before meals and snacks. Glargine cannot be mixed with any diluent nor with any other insulin, which means that use of this regimen requires multiple daily injections. Lispro and aspart can be given with use of an insulin pen device, which simplifies administration. Starting dose calculations are shown in Box 378.3. These doses are based on empiric formulas, and modifications can be made once responses to starting doses are assessed. Bolus doses with rapid-acting insulin have two parts to the dose: the amount of insulin needed to cover the carbohydrates in the meal (the insulin-to-carbohydrate ratio) and the amount of insulin needed to correct for a blood glucose level outside the target range (the correction or sensitivity factor). Thus, the bolus dose for a meal is calculated based on the insulin-to-carbohydrate ratio and the amount of carbohydrates the child plans to eat, and then the calculated dose is increased or decreased based on the amount that the blood glucose is above or below the target range. Target ranges may be set at 80 to 120 for daytime and 100 to 150 at bedtime, for example. When converting a child from a twoor three-shot regimen with NPH to a basal/bolus regimen, the total daily dose usually is lower and recommendations are to use 50% to 80% of the NPH dose for the initial basal insulin dose, with the lower percentages used for younger children.
Insulin pumps offer the ability to have different basal rates at different times of the day. Basal/bolus regimens offer near complete flexibility in timing and amount and content of meals and snacks.
When insulin doses are more than 1.5 U/kg/day and especially when they are at or more than 2 U/kg/day, overtreatment must be considered. Excess doses of insulin can worsen control and produce a clinical picture of widely variable blood glucose values. The Somogyi phenomenon is rebound hyperglycemia after hypoglycemia, which may be asymptomatic. It is caused by release of counterregulatory hormones (e.g., catecholamines, cortisol, glucagon, growth hormone) in response to hypoglycemia.
Improvements in the purity of insulin preparations have decreased local and systemic allergic reactions markedly. Lipoatrophy, the loss of subcutaneous adipose tissue, is a rare finding. Lipohypertrophy, increased deposits of adipose tissue, still occurs and may be related to poor injection technique. Proper site rotation is important in preventing lipohypertrophy.
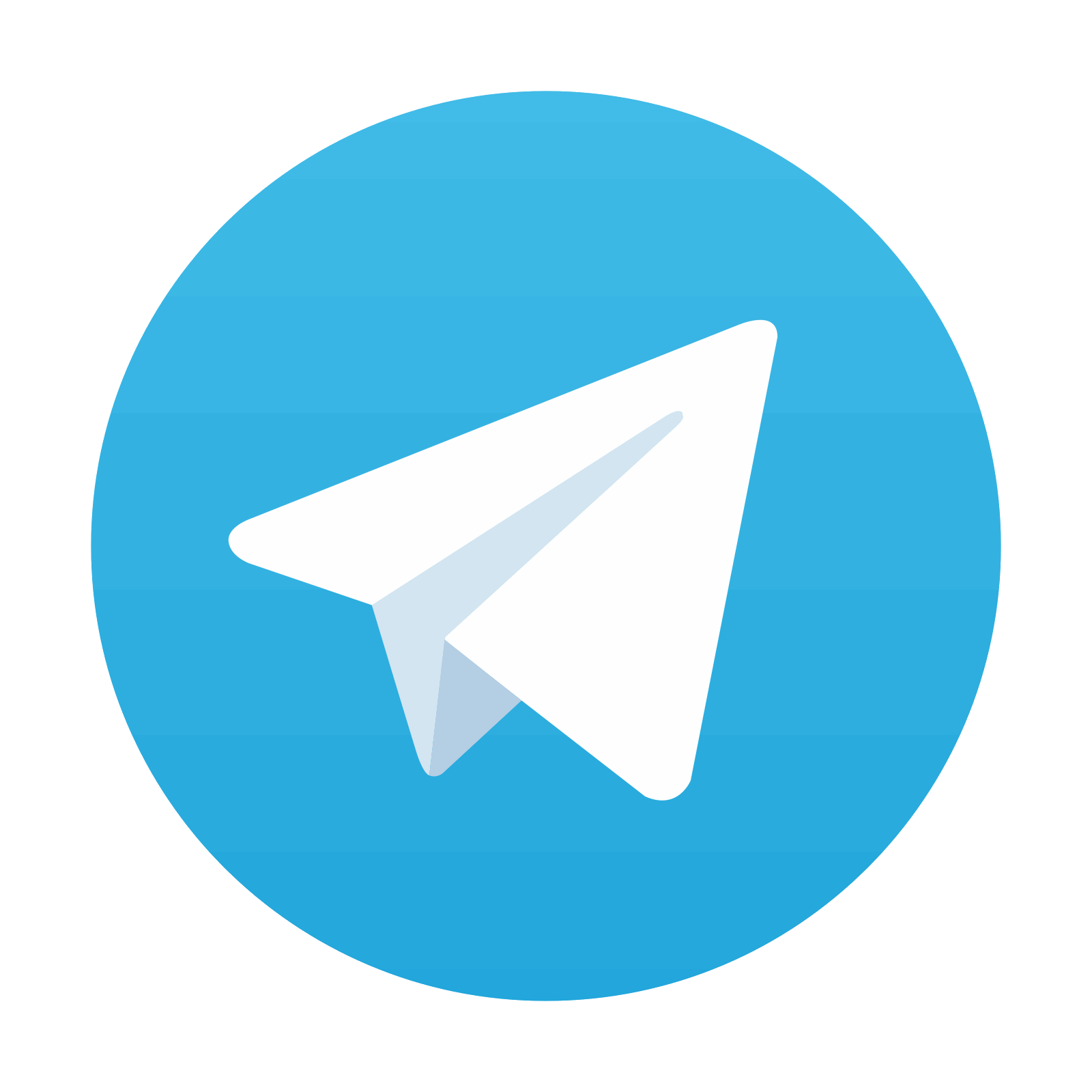
Stay updated, free articles. Join our Telegram channel

Full access? Get Clinical Tree
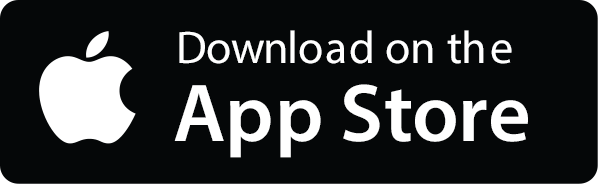
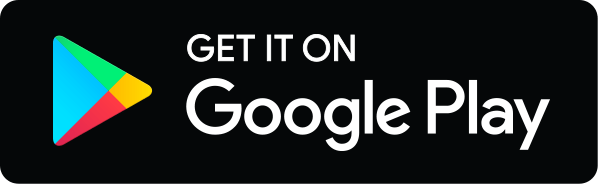
