American Medical Association grading scale
Clinical laxity (mm) [39]
Radiographic widening (in 20° flexion) [40]
Grade I
Localized tenderness but no instability
3–5 mm
3.2 mm difference compared to contralateral side
Grade II
Localized tenderness and a partial tear of the MCL and POL
6–10 mm
–
Grade III
Complete disruption and instability with valgus stress testing
>10 mm
9.8 mm difference compared to contralateral side
Table 34.2
Average gapping increase compared to normal knee
Protocol | Medial joint gapping (mm) in 0° knee flexion | Medial joint gapping (mm) in 20° knee flexion |
---|---|---|
Intact | 7. ± 0.7 | 7.4 ± 0.7 |
Proximal sMCL | 9.4 ± 1.0 | 10.6 ± 1.9 |
MF | 9.9 ± 1.2 | 12.2 ± 2.0 |
POL | 12.2 ± 1.5 | 14.1 ± 2.1 |
Distal sMCL | 13.2 ± 2.6 | 15.3 ± 2.3 |
MT | 14.1 ± 2.8 | 16.2 ± 2.8 |
ACL | 15.9 ± 3.9 | 21.2 ± 3.9 |
PCL | 21.6 ± 4.2 | 27.8 ± 4.7 |
In combined injuries, valgus stress testing at 0° of flexion is more informative [41]. Excessive laxity on valgus stress will indicate injuries to the MCL and secondary stabilizers of the knee [42]. With the anterior drawer, MCL and ACL tears together may result in greater anteroposterior (AP) translation [8, 10]. The Slocum-modified anterior drawer test is a way to identify PMC injuries. An external rotation anterior drawer test, performed in 10–15° of external rotation of the tibia, exposes PMC injuries [43]. External rotation stress is thought to be applied in the following order: PMC, anterior MCL, and ACL. Conversely, intact lateral-sided ligaments will prevent an anterior drawer of the tibia on the femur when performed in 30° of internal rotation even if the MCL and ACL are torn.
The dial test, more commonly used to detect posterolateral corner (PLC) and PCL injuries, can also show increased external rotation at 30 and 90° of flexion with medial-sided injuries [14, 41]. Performing the examination in both the supine and prone position can be used to distinguish the difference between anteromedial and posterolateral tibial rotation, using a combination of visualization and palpation [14].
Laterally displaceable patellae and extensor mechanism damage have been variably reported in the literature to occur in 9–59 % of combined ligament injuries [44, 45]. While these injuries rarely have been found to cause instability, the literature that examines their relative contribution is poor, and careful examinations should be performed to identify potentially aggravating injuries.
34.3 Imaging
Acutely, static widening of the medial joint space on plain radiographs can indicate a medial-sided injury or structure incarceration, e.g., medial capsule or MCL (≥5 mm). The “irreducible” knee dislocation can present this way following posterolateral joint subluxation or vastus medialis entrapment [46, 47]. Valgus stress radiographs can confirm suspicions of medial-sided injury [14, 17]. LaPrade et al. quantified side-to-side differences of 1.7 mm and 3.8 mm at 0° and 20°, respectively, in isolated MCL tears and 6.5 mm and 9.8 mm at 0° and 20°, respectively, in combined MCL and posteromedial corner disruption [40]. Otherwise, an examination under anesthetic can be used to detect rotatory injuries not previously detected by preoperative imaging or examination [42].
Chronically, radiographic changes can provide clues to the pattern of underlying injury. A Pellegrini-Stieda lesion, an ossified posttraumatic avulsion lesion of the MCL from the medial epicondyle of the femur [48], a deep femoral notch sign, peaked tibial spines, or cupula lesions can indicate long-standing MCL and ACL injuries.
MRI without contrast remains the gold standard where the diagnosis of medial-sided knee injuries can be performed with an accuracy of 87 % [49]. Its greatest advantages are in suspected complete MCL tears, suspected ACL tears, persistent clinical instability, and identifying the location of tear where surgery is required [50]. Individual medial-sided structures and the exact location of the injury can be visualized (see Fig. 34.1) [51]. MRI arthrograms enhance the identification of PMC injuries. Kimori et al. found arthrography to be more useful than arthroscopy and clinical examination in detecting tears, but interpretation can be difficult [51–53].


Fig. 34.1
Coronal images of type I (a, b) and type III (c, d) MCL injuries. The superficial fiber, which is depicted as low-signal image on spin echo (a, arrow) and gradient echo (b, arrow) images, is interrupted by high-signal image at the femoral attachment site in type I MCL injury. In contrast, interruption of the superficial fiber by high-signal image is observed throughout the length of the fiber in type III MCL injury (Ref. [53])
Nakamura et al. (contributing author) developed a new classification for MCL injuries based on the appearance of the superficial medial collateral ligament (sMCL) on MRI: femoral insertion site injury (type I), tibial insertion site injury (type 2), or injury throughout the length of the MCL (type 3) [53]. All five of their type 3 injuries required MCL reconstruction and there were no type 2 injuries. No differences were observed in IKDC sagittal laxity or valgus stability in all injuries.
Ligament discontinuity, subcutaneous edema, internal (ligament) change of signal intensity, and contrecoup bipolar bone bruises have all been associated with MCL tears [54–56]. When the pivot-shift mechanism does not dissipate all the deforming forces of certain high-energy injuries, varus, the internal rotation impaction on the anteriorly subluxated proximal tibia, is thought to lead to central medial femoral condyle and posterior tibial plateau contusion [57, 58].
The recently described “wave sign” indicates a distal tibial avulsion injury (Fig. 34.2a–c) [36]. This is thought to occur because the distal end of the ligament is not tethered to other soft tissue structures locally and takes on a serpiginous appearance when it retracts proximally. Taketomi et al. described three types: an avulsion injury where the distal end of the torn ligament remains under pes anserinus, the so-called “Stener” lesion of the knee where the distal end of the ligament sits outside pes anserinus [59], and MCL incarceration within the joint. They make the argument that potentially all of these types of MCL tears require surgical intervention.


Fig. 34.2
(a) “Wave sign”: the waving of the superficial layer (triangle). (b) The distal end of the superficial MCL (arrow). (c) The entrapment of the distal end of the superficial layer into the medial knee joint (arrow head) (Ref. [36])
34.4 Pathoanatomy and Applied Anatomy Relating to Combined ACL/MCL Injury
LaPrade et al. have extensively described (1) bony landmarks, (2) ligaments, and (3) tendons (adductor magnus, medial head of the gastrocnemius, semimembranosus, and the pes anserinus) of the medial side of the knee [21]. The MCL complex is made of the sMCL, the deep medial collateral ligament (dMCL), and POL (part of PMC) [23]. The other constituent components of the PMC are the semimembranosus tendon (and its multiple reflections), the oblique popliteal ligament, posterior horn of the medial meniscus, and medial joint capsule [60]. The sMCL has one femoral and two tibial attachments (proximal and distal). The femoral attachment is located 3.2 mm proximal and 4.8 mm posterior to the medial epicondyle [21]. Many reconstruction techniques incorrectly identify the medial epicondyle as the attachment site of the MCL [15, 26, 61–64]. The tibial insertion is broader, attaching primarily to soft tissues proximally and to bone distally, 60 mm from the joint line [65]. The dMCL is a vertical thickening of the medial joint capsule and consists of the MF (attaching 15.1 mm posterior and distal to the medial epicondyle) and MT ligaments (3.2 mm from medial tibial plateau) [65].
The posterior oblique ligament (POL) arises from behind the medial femoral epicondyle, 7.7 mm distal and 6.4 mm posterior to the adductor tubercle [65]. It fans out from its origin with three fascial arms: superficial, central, and capsular [21, 61]. The central arm is the largest, inserting near the margin of the tibial articular surface, the capsular arm reinforces the PM joint capsule, and the superficial arm blends with semimembranosus.
The MCL complex is a primary restraint to direct valgus stress. It also secondarily contributes to external rotation and anteroposterior stability [23]. The sMCL provides the majority of this stability in all degrees of flexion; the dMCL only providing secondary stability. The distal division of the sMCL is a primary stabilizer for external rotation and the POL, the primary stabilizer for internal rotation, highlighting its importance in counteracting AMRI [41].
The PMC provides one third of the restraint to valgus stress in full extension, slackening off in flexion [66]. It has a secondary role in the prevention of posterior translation of the tibia. However, in the context of combined injuries, it has a more important role in the resistance to external rotation. When damage to the PMC is combined with an MCL tear, external rotation is increased by 30° [42]. Failing to address the rotational component of this injury is what is thought to lead to residual laxity and functional compromise and the main source of controversy surrounding repair or reconstruction techniques.
Pes anserinus tendons and semimembranosus have a role in tightening medial structures in external rotation and flexion. In the context of damage to medial structures, utilization of these tendons to reconstruct MCL or POL may compromise the results of surgery inadvertently. Avoiding the harvest of hamstring autograft may be preferable, instead of favoring other graft options in these cases.
34.5 Treatment
ACL reconstruction and nonsurgical treatment of grade I and II MCL injuries have outcomes similar to that of isolated ACL injury reconstructions [67, 68]. Based on this, many authors propose protection of the MCL with a knee brace and delaying ACL reconstruction surgery [1, 69, 70]. Usually a period of 6–8 weeks is required for MCL injuries to heal.
The abovementioned approach can be utilized with grade III MCL injuries even among professional athletes with successful results [7]. However, the persistent valgus and/or AMRI of certain MCL tears can compromise ACL reconstructions if the medial side is not addressed [8, 11–13]. Both of these situations of compromised stability can prevent athletes from returning to pivoting sports [1, 2, 17].
34.5.1 Nonoperative Management of MCL Injuries
The indications for the nonoperative management of both ACL and grade III MCL tears are rare [71], with very little published on the topic [72, 73]. A higher rate of instability and a lower rate of return to sport make this a less desirable option. A number of studies have evaluated the nonoperative treatment of grade III MCL tears with concurrent reconstruction of the ACL [3, 28–30, 74–76]. Halinen et al. found that nonoperative MCL management regained ROM and quadriceps strength faster [28]. Petersen and Laprell compared early and late ACL reconstruction and reported significantly higher reoperation rates for stiffness and lower Lysholm scores with early ACL reconstruction [30]. Nonoperative management of MCL injuries is not as much of an issue as early ACL reconstruction. The vast majority of surgeons prefer not to operate in the acute phase for this reason [68, 76]. However, these studies also do not confirm superiority of nonoperative MCL management. Although sagittal and valgus stability is generally restored [3, 28–30], regaining ROM can still be an issue [28, 30, 74, 76].
Many authors have recommended a “wait and see” approach [1, 2, 33, 69, 77], bracing patients to resist coronal plain movement while permitting weight bearing and ROM for 6–8 weeks [1, 17, 78, 79]. At the time of ACL reconstruction, radiography can be used for an examination under anesthesia and valgus stress views obtained on the table [53]. Residual valgus instability after ACL reconstruction, illustrated by the medial joint space opening up more than 7–10 mm in 30° of flexion compared to the other side, should be an indication to proceed onto MCL reconstruction [33, 53, 69]. Significant residual instability can also be confirmed with arthroscopic valgus stress testing. Eight to 10 mm of opening of the medial compartment suggests persistent instability.
34.5.2 Operative Management of MCL Injuries
34.5.2.1 MCL Repair
Different treatment combinations reflect changing trends in management over time [1, 17–19]. Opinion has shifted from early repair of the MCL and reconstruction of the ACL to delayed reconstruction of both ligaments when needed [1, 27, 33, 77].
Proponents of MCL repair report relatively good correction of valgus laxity with the advantage of avoiding complicated reconstruction options [68, 80–82]. Many reconstruction options are nonanatomic and only address the anterior portion of the superficial MCL [25, 26, 63]. Surgery in the acute phase is facilitated by more pliable tissue and more easily identifiable anatomical structures [25, 26]. The trade-off is a reduction in range of motion and possibly rotatory stability. Postoperative stiffness has proven to be a problem with early surgery, with 19–38 % MUA rates [28, 30, 68, 70, 76, 81–83]. Older rehabilitation protocols have been suggested as a possible cause for these findings.
Doubt has also been cast over the rotational stability of MCL repairs [63]. A recent study by Dong et al. looked at a triangular-vector reconstruction technique versus an anatomic repair technique of the MCL (See Fig. 34.3a–c) [63]. Both treatment methods effectively treated valgus instability, but medial pain and rotational instability were higher in the repair group. Repaired oblique fibers of the middle of the MCL and POL were not able to restore the medial structures to their original level of function [12, 84].


Fig. 34.3
(a) Photograph of triangular reconstruction technique. (b) Illustration of same technique. (c) Illustration of MCL repair using suture anchors (Ref. [24])
Although MCL repair in the acute phase is not typically offered, severe valgus alignment, large bony avulsions, and sMCL tibial avulsions that get incarcerated in the joint or displaced to the other side of the pes anserine tendons (“Stener-like” lesion of the knee) are all indications for acute MCL repair [33]. Although there is no high-level evidence to support the acute fixation of these lesions, much like the Stener lesion of the thumb, it is unlikely that the distally avulsed end of the sMCL will heal to its anatomic footprint if there is interposition of the sartorius fascia and hamstring tendons [59, 68].
Our preferred approach for repair is through a medial-sided 4 cm incision centered over the medial femoral epicondyle down to the crural fascia. Under fluoroscopic control, the isometric point of the proximal sMCL insertion is found as described by Wijdicks et al. [20]. The injured structures are repaired from the deepest structures outward. A peripheral tear of the medial meniscus is commonly seen (33 %) and repaired with an open technique. An MF ligament tear can be directly repaired using sutures alone or suture anchors. Suture anchor fixation is preferred for MT ligament tears.
For proximal avulsions of the sMCL, its attachment site is found and a 3.2 mm drill is inserted to a depth of approximately 35–40 mm. The MCL is prepared with a modified running locking stitch up each side. A small slit is then made proximally, and a 4.5 mm screw with a soft tissue spiked washer is placed through the slit (see Fig. 34.4) [85]. Sutures from the free end are also tied around the screw. Final tensioning is performed with the leg in about 20–30° of flexion and slight varus.
Distal sMCL avulsions can be approached through an anteromedial incision midway between the PM border of the tibia and the tibial tubercle. The sartorius expansion is incised over the top of the pes tendons and the tendons retracted distally. Most distal avulsions occur distal to the level of the pes tendons. The sMCL can be retracted proximally some distance. Two anchors are used to reattach the proximal sMCL 1 cm below the joint line. These sutures are then weaved through the proximal MCL fibers but not tied. Then similar to the proximal MCL attachment, after lock stitching the distal ligament is split and the limbs tied around and secured by a screw and washer construct [86]. Tensioning is performed with the leg in about 20–30° of flexion and slight varus. Once the distal avulsion has been repaired, the leg is placed in full extension and the proximal anchors are sutured securely.
Whelan et al. (senior author) recently showed the biomechanical reliability of a “double row” repair of distal sMCL avulsion injuries (suture-bridge repair technique) [87]. Double row repair, in the shoulder, has shown greater healing and lower re-rupture rates, encouraging its application in the knee [88, 89]. Double-loaded suture anchors are placed at the proximal aspect of the sMCL anatomic insertion on the tibia and passed through the ligament tissue and tied but not cut. “Press fit” suture anchors are then placed at the distal aspect of the sMCL anatomic insertion site on the tibia to secure the retained sutures from the proximal anchors. The proximal sutures are “crossed over” before being secured distally as per standard suture-bridge configuration (see Fig. 34.5a, b).


Fig. 34.5
(a) sMCL suture-bridge repair technique. Double-loaded suture anchors are placed at the proximal aspect of the sMCL anatomic insertion on the tibia, and the sutures are passed through the ligament tissue and tied but not cut. (b) “Press fit” suture anchors placed at the distal aspect of the sMCL anatomic insertion on the tibia to secure the retained sutures from the proximal anchors. The proximal sutures are “crossed over” before being secured distally as per standard suture-bridge configuration (Ref. [87])
If required, posteromedial structures can be tightened to improve resistance to AMRI. Two methods have been described by Jackson et al. [90]. The first of these is based on a technique described by Hughston et al. [91]. Laxity is removed by increasing the distance between the origin and insertion of the lax structure. The Lax segments are attached to surrounding intact structures, increasing the distance the ligament or tendon travels, increasing its tension. This is then followed by mattress stitch imbrication of the body of the structure. Alternatively, the posterior medial capsule can be released from the meniscus and re-sutured to it in a more advanced position in a “pants-over-vest” fashion. Both of these procedures are best performed with the patient supine, the hip in external rotation, and the knee positioned in 30° of flexion, internally rotated and under gentle varus stress.
34.5.2.2 MCL Reconstruction
Chronic valgus laxity resulting in symptomatic instability unresponsive to conservative treatment is an indication for MCL reconstruction [17, 69]. Abnormal shear stresses and load patterns in an unstable knee can lead to degenerative change [92]. To avoid this, addressing all injured medial knee structures by restoration of native anatomy and insertion sites are recommended [17–19, 93]. Reconstruction techniques differ in graft choice, fixation method, tensioning method, number of bundles, and the medial structures they aim to reconstruct. No true consensus on the optimal method of reconstruction exists at the current time.
Reconstruction techniques can be split into three categories: anatomic, nonanatomic, and nonanatomic tendon transfer reconstructions [77]. LaPrade et al. described an anatomical reconstruction of MCL and POL to their precise, native attachment sites using hamstrings double-bundle autografts (see Fig. 34.6) [18]. Medial joint space gapping was <3 mm in all 24 of their patients. Accurate restoration of anatomic attachment sites, with independent ligament tensioning, may explain these good results. However, the extensive approach and requirement for multiple tunnels add complexity to the operation. Inadvertent disruption of bone tunnels created for other ligament reconstructions can lead to graft failure of either or both ligaments. Concerns have also been raised about stress shielding and altered knee mechanics that results from significantly over-tensioned grafts [94].


Fig. 34.6
A diagram of a right knee illustrates the superficial medial collateral ligament (sMCL) and posterior oblique ligament (POL) reconstruction grafts (Ref. [93])
Significant heterogeneity exists among nonanatomic reconstruction techniques [12, 16, 26, 63, 95–97]. Single- and quadruple-bundle hamstring autografts appear to perform equally well, with minimal medial joint gapping on valgus stress [16, 26, 95]. Tendo-Achilles (T-A) allograft is also a popular choice of graft, avoiding further compromise of medial stability through the sacrifice of hamstring autografts. Both single- and double-bundle techniques have achieved good resistance to medial gapping [12, 97]. Quadriceps tendon and bone-patella-tendon-bone techniques have also been described [16].
Dong et al.’s nonanatomic triangular-ligament reconstruction with a single-bundle semitendinosus allograft appeared to show superior control of rotatory instability compared to anatomic repair. Their graft was fixed into both ends of an anterior to posterior drilled tibial tunnel [63]. The intervening tendon is fixed at the apex of the construct in a single femoral tunnel at the level of the medial epicondyle of the femur (see Fig. 34.3).
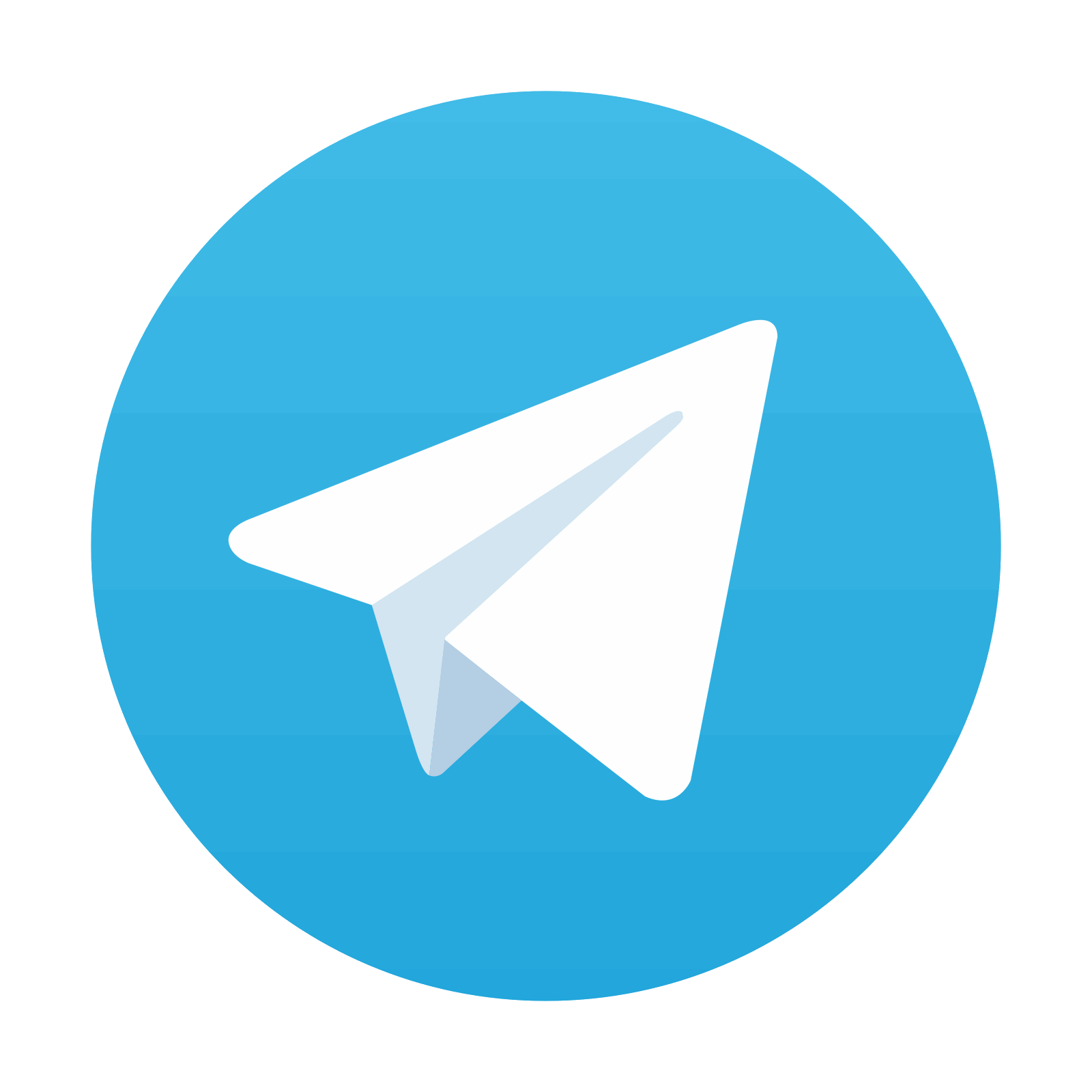
Stay updated, free articles. Join our Telegram channel

Full access? Get Clinical Tree
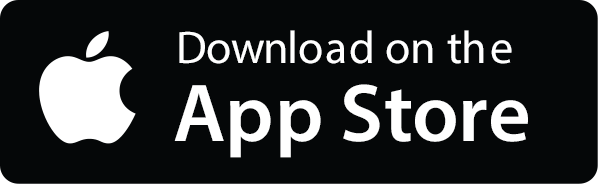
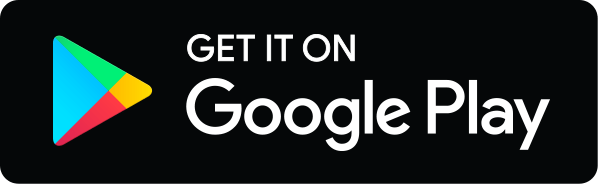