INTRODUCTION
ESSENTIALS OF DIAGNOSIS
Traumatic brain injury (TBI) may be mild, moderate, or severe.
In mild TBI, duration of loss of consciousness (LOC) is <30 minutes, duration of post-traumatic amnesia (PTA) is <1 hour, and Glasgow Coma Scale (GCS) score is 13–15.
In moderate TBI, duration of LOC is <24 hours, duration of PTA is between 1 and 7 days, and GCS score is 9–12.
In severe TBI, duration of LOC is >24 hours, duration of PTA is >7 days, and GCS score is ≤ 8.
Computed tomography (CT) scan of the head is the initial imaging modality of choice.
GENERAL CONSIDERATIONS
Traumatic brain injury (TBI) is a major cause of morbidity in the United States and the world. A wide range of deficits can be seen, including physical, cognitive, and behavioral problems, and difficulties encountered in one area may hinder the patient’s progress in other areas. TBI is characterized by a change in neurologic functioning and can be classified as mild, moderate, or severe. Because some neuromedical problems seen after TBI are rather specific to the brain injury population, the rehabilitation physician must be familiar with diagnosing and treating these complications. TBI rehabilitation encompasses the spectrum of care from the intensive care unit (ICU) through acute inpatient rehabilitation, and outpatient care that extends to family, community, vocational, and avocational activities. Appropriate rehabilitation improves functional outcome, reduces complications, and enhances the quality of life for individuals with TBI and those in their social network.
The Centers for Disease Control and Prevention (CDC) estimates that there are 1.7 million TBIs annually in the United States. Of these, approximately 75% are considered to be mild TBI, or concussions. Despite this fact, brain injury is thought to be a contributing factor in nearly one third of all injury-related deaths. Of the 1.7 million patients with TBIs annually, 80% are treated and released from an emergency department; however, 275,000 are hospitalized. The CDC estimates that 52,000 people die each year from injuries related to head trauma. Currently there is no estimate for the number of brain injuries that occur in which people never present to an emergency department or seek medical care. The CDC estimates the total of direct and indirect costs related to TBI to be $60 billion annually.
Falls are the leading cause of all TBIs and are responsible for 35% of head injuries in the general population. Falls are an even greater cause of TBI in children and the elderly, accounting for 50% of TBIs in children younger than 14 years and for 61% of TBIs in adults aged 65-plus. Motor vehicle accidents and traffic-related accidents cause 17% of head injuries. Struck by or against events (eg, colliding with a moving or stationary object) cause another 16% of head injuries. Assaults account for 10% of head injuries. Brain injury is more common in males than females across all ages. Additionally, alcohol intoxication is involved in 12% of hospitalizations due to head injury and in nearly 50% of all head injuries. Blast injury is a leading cause of TBI in military personnel. Blast injuries involve force applied to the brain and body from explosion-generated pressure waves and also from flying debris.
PATHOGENESIS
Changes in the brain due to trauma are the result of a complex interplay between the direct force and the resultant cascade of neurochemical and hemodynamic changes. Primary injuries are those caused by the direct force of the trauma, while secondary injuries are a result of the cascade of intracerebral changes. Primary injuries include focal pathology such as contusions, hematomas (subdural, epidural, subarachnoid, intraparenchymal), lacerations, and direct injury from penetrating or missile injuries. Primary injury can also be diffuse in nature as is the case in diffuse axonal injury.
Contusions result from bruising of the surface (cortex) of the brain. Areas of the cortex that are most susceptible to contusion injury are those that are in close proximity to irregular bony areas of the skull vault. These areas include the anterior temporal lobes and the inferior frontal lobes.
Hematomas are hemorrhages in and around the brain. They are defined by their location and by the type of injured blood vessels that result in the hemorrhage. Epidural hematomas are located between the dura mater and the skull. They result from injury to arteries and classically involve the middle meningeal artery but can also occur due to middle meningeal vein injury. Skull fractures are present in over 90% of cases. Epidural hematomas appear convex on neuroimaging.
Subdural hematomas are located in the subdural space between the dura and arachnoid. They result from injury to bridging veins. Risk factors for subdural hematoma include older age and alcoholism. These hematomas have a concave appearance on neuroimaging.
Subarachnoid hematomas are located in the space between the arachnoid and pia mater. They are also the result of injury to microvessels. Traumatic subarachnoid hematoma is frequently found near areas of contusion. Blood products in the subarachnoid space increase the risk for communicating hydrocephalus due to obstruction of arachnoid villi, and also increase the risk of vasospasm.
Intraparenchymal hematomas are located within the brain tissue itself and are also referred to as intracerebral hemorrhages. They are most commonly found in association with cortical contusions in the frontal and temporal lobes. Intraparenchymal hematomas are the result of severe cortical contusions, lacerations, or penetrating injury.
Primary injuries also can be diffuse in nature, as is the case with diffuse axonal injury. Diffuse axonal injury is caused by sheer and tensile strains on axons as a result of angular acceleration of the brain. The axons of the cerebral white matter, corpus callosum, and brainstem are particularly vulnerable to this type of injury. Diffuse axonal injury is a complex entity in which mechanical stretch of an axon leads to changes in axon permeability, resulting in disruption of axon transport and impaired cellular metabolism. This can cause immediate or delayed cell death.
Although much attention is, understandably, focused on the primary injury, it would be foolish to overlook the importance of secondary injury. Secondary injuries significantly contribute to morbidity and mortality. Cerebral edema has been described as a hallmark finding in severe TBI. Cerebral edema is a result of brain swelling from vasogenic edema and vascular dysregulation, which leads to increased cerebral blood volume. Cerebral edema plays a prominent role in the development of elevated intracranial pressure (ICP). Elevated ICP, or intracranial hypertension, compromises cerebral perfusion pressures, putting the patient at risk for ischemic injury. Additionally, intracranial hypertension can produce brain herniation syndromes. Focal injuries such as hematomas also contribute to secondary injury via mass effect and edema, resulting in compression of blood vessels, alterations of cerebral perfusion pressures, and brain herniation syndromes. Areas at greatest risk of secondary ischemic injury post-TBI include the hippocampus, basal ganglia, and cerebellum. Excitotoxicity is another form of secondary injury in which damaged neurons release large amounts of excitatory neurotransmitters, such as glutamate, which build up to toxic levels causing cell death. Apoptosis has also been identified as a form of secondary injury as damaged cells undergo programmed cell death.
Focal injuries may present clinically with a loss of function directly corresponding to the region of the brain that is injured. However, it is important to remember that both focal and diffuse injuries can exist at the same time in the same patient. In addition, the presence of secondary injuries can alter the clinical picture. For these reasons, diffuse injury and secondary injury may mask focal deficits that would be anticipated from focal pathology.
CLINICAL FINDINGS
The most important aspect in diagnosing a brain injury is a clinical history of trauma. Brain injury results from a traumatic force and may occur after direct trauma to the head, or trauma to the body that is then transmitted to the head. Neurologic functioning is objectively measured using the Glasgow Coma Scale (GCS; Table 13–1). Duration of loss of consciousness (LOC) and duration of post-traumatic amnesia (PTA) are key features of this evaluation.
Scale Value | Best Motor Response | Best Verbal Response | Best Eye Opening Response |
---|---|---|---|
6 | Obeys commands | Oriented | — |
5 | Localizes stimulus | Oriented | — |
4 | Withdraws from stimulus | Conversant, but confused | Eyes open spontaneously |
3 | Flexes arm | States recognizable words or phrases | Eyes open to voice |
2 | Extends arm | Makes unintelligible sounds | Eyes open to painful stimulus |
1 | No response | No response | Remain closed |
A rehabilitation professional performing an assessment of a patient with TBI will likely be confronted by a wide spectrum of clinical findings. Disorders of consciousness, weakness, impaired cognition, aphasia, apraxia, incoordination, visuospatial dysfunction, impaired balance, and spasticity are some of the most common clinical findings in brain-injured patients. Owing to the complex interplay among primary injury, secondary injury, focal injury, and diffuse injury, these deficits are less likely to exist in isolation or in discrete patterns, as might be the case in stroke syndromes. Instead, they are more likely to exist in complex combinations of deficits. Some of these clinical findings are outlined in greater detail below.
Severe TBI can result in disorders of consciousness, the defining behavioral features of which are listed in Table 13–2. Disorders of consciousness are manifested by profound impact on alertness, cognition, and behavior. The most severe of these disorders is coma. In the comatose state there is an absence of sleep–wake cycles, the eyes remain closed, and the patient is unable to be aroused. The loss of arousal includes the absence of spontaneous arousal and stimulus-induced arousal. Coma is typically a self-limited condition that resolves within 2–4 weeks.
Behavior | Coma | Vegetative | Minimally Conscious |
---|---|---|---|
Eye opening | None | Spontaneous | Spontaneous |
Spontaneous movement | None | Reflexive/Patterned | Automatic/Object manipulation |
Response to pain | None/Posturing | Posturing/Withdrawal | Localization |
Visual response | None | Startle | Object recognition/Pursuit |
Affective response | None | Random | Contingent |
Commands | None | None | Inconsistent |
Verbalization | None | Random vocalization | Intelligible words |
Communication | None | None | Unreliable |
Some cases of coma progress into the other disorders of consciousness, vegetative state and minimally conscious state. The vegetative state typically represents a transitional state between coma and minimal consciousness or consciousness. The Multi-Society Task Force has stated that all of the following conditions must be present to diagnose vegetative state: (1) intermittent wakefulness manifested by sleep–wake cycles (ie, times during which the eyes are open); (2) no evidence of sustained reproducible, purposeful, or voluntary behavioral responses to visual, auditory, tactile, or noxious stimuli; and (3) no evidence of language comprehension or expression.
The terms persistent vegetative state and permanent vegetative state are frequently the source of great confusion and misuse by the general public and also within the medical community. The terms are often incorrectly applied or inappropriately used interchangeably. The Multi-Society Task Force determined that the term persistent should apply when the vegetative state is present for 4 or more weeks. The term permanent vegetative state is used to denote a time point after which the probability for recovery of consciousness is poor. The Task Force determined that the vegetative state should be considered permanent when it has been present for 3 months or more after hypoxic–ischemic, metabolic, and congenital brain injuries, and only after at least 12 months following a TBI.
The minimally conscious state was defined by the Aspen Neurobehavioral Working Group as “a condition of severely altered consciousness in that there is minimal but definite behavioral evidence of conscious awareness.” The diagnosis is based on the bedside examination revealing at least one of the following behaviors: (1) simple command following, (2) intelligible verbalization, (3) recognizable verbal or gestural “yes–no” responses, and (4) movements or emotional responses triggered by relevant environmental stimuli that cannot be attributed to reflexive activity.
The Working Group also identified two behaviors that signify the emergence from a minimally conscious state: (1) reliable demonstration of interactive communication, and (2) functional object use. It is important to note that for a patient to meet the criteria for emergence from a minimally conscious state, reliable communication or functional object use must be consistent, not merely reproducible.
Assessment of disorders of consciousness is best performed using a standardized neurobehavioral rating scale. A brief bedside neurologic examination has poor reliability for determining LOC. For example, 30–40% of patients who cannot speak and do not follow commands are incorrectly diagnosed as being vegetative. The use of a standardized neurobehavioral rating scale minimizes the risk of both diagnostic and prognostic error. Although several formal rating scales are available for use, the Disorders of Consciousness Task Force determined that the Coma Recovery Scale–Revised (CRS-R) is the most acceptable scale. The CRS-R is a widely used and accepted tool for diagnosing and monitoring progression of disorders of consciousness. Its six subscales each address a functional system: auditory, visual, motor, oromotor/verbal, communication, and arousal. Within each subscale, itemsare hierarchically arranged so that the lowest items reflect brainstem-mediated functions and the highest, cortical functions. A sample CRS-R scoring sheet is shown in Figure 13–1.
A broad spectrum of cognitive impairments can be seen after TBI. Information processing and attention, general intellectual functioning, memory, spatial cognition, and executive functions are among the prime cognitive domains affected by TBI. The patient may indicate a lack of awareness of the deficits, even when these are significant. When evaluating a patient for cognitive dysfunction post-TBI, it is important to take into account any other conditions that may be affecting his or her abilities. For example, preexisting conditions such as learning disabilities or attention-deficit/hyperactivity disorder may affect cognitive abilities. Factors related to the brain injury itself, such as seizures, medication side effects, and pain, can also affect cognitive abilities. Mood disturbances such as depression have also been shown to negatively influence cognitive test scores.
Information processing involves both the speed and accuracy of responses to external stimuli. The control of information processing is not well localized in the brain. Instead, it is controlled by broad networks, including multiple cortical and subcortical structures. Delayed information processing is manifested in TBI patients by prolonged response times. Diffuse injury disrupting cognitive networks results in the patient having to expend more mental effort and energy to perform a task that previously would have been easily accomplished. This increased energy expenditure results in both mental and physical fatigue.
Anosognosia refers to the loss of awareness of deficits. It is a condition in which the patient does not fully acknowledge that there is any neurologic dysfunction. Anosognosia has been identified as a barrier to rehabilitation, often due to the patient’s reluctance to participate in a formal rehabilitation process. It is unclear if anosognosia is a result of purely psychological denial, purely cognitive dysfunction from disruption of several cognitive domains, or is multifactorial in nature.
Overall intellectual functioning is impaired in patients with TBI. This can be measured by a variety of formal evaluations; however, the Wechsler Adult Intelligence Scale is widely used. This scale generates an intelligence quotient (IQ) score, but more importantly, allows for evaluation of a broad range of cognitive capacities ranging from digit span to language, attention, and vocabulary. Again, it is important to note that IQ scores will be heavily influenced by slow information processing, aphasia, and inattention.
Memory impairment is a hallmark feature of traumatic brain injury. Initially, impairments in memory manifest as post-traumatic amnesia (PTA). PTA is defined as the timeframe in which brain-injured patients are unable to encode or retain new information. It is manifested by confusion, agitation, perseveration, and disorientation. The duration of PTA is a powerful predictor of long-term functional outcome post-TBI (see Prognosis, below). As such, formal tools have been devised to evaluate the progression and emergence of PTA. Two such tools are the Galveston Orientation and Amnesia Test (GOAT) and the Orientation-Log (O-log). Once a patient attains a score above 75 on the GOAT or above 34 on the O-log on two consecutive evaluations, he or she is considered to have emerged from the period of PTA. After emergence from PTA, there is a great variability between individuals with respect to the severity of memory impairments. Again, it is prudent to consider the effects of pain, sleep disturbances, mood disturbances, and medication side effects on learning and memory. Although learning and memory are controlled by multiple neurologic structures and networks, the medial temporal lobe is integral to these functions. There is significant correlation between memory impairment and decreased volumes in the hippocampi and medial temporal white matter. It remains unclear whether memory deficits are due to dysfunctions in encoding, consolidation, or retrieval.
Spatial cognition skills are also affected post-TBI. The term spatial cognition is broad and encompasses multiple functions, including visual scanning, exploring one’s environment, constructional praxis, route finding, and leaning of spatial patterns and complex scenes. Hemispatial neglect can also be seen post-TBI and is associated with injury to the right parietal lobe.
Executive functions can also be impaired in the brain-injured patient. Executive functions are the processes of the brain responsible for higher order complex activities such as planning, judgment, and decision making. These processes are reliant upon working memory, prospective memory, strategic planning, cognitive flexibility, abstract reasoning, and self-monitoring to function properly. The frontal lobe is thought to be responsible for executive function, in conjunction with broad cortical and subcortical circuits. The frontal lobe executive function networks have been divided into the dorsolateral, orbital, and medial prefrontal networks. The dorsolateral prefrontal network is thought to be responsible for abstract reasoning, planning, and working memory; the orbital prefrontal network, for social and emotional aspects of behavior, emotion-based learning, and social judgment. The medial prefrontal network is thought to be responsible for motivational and attentional processes, which include initiation, inhibition, and maintenance of behavior. The orbital and medial prefrontal areas of the brain are more susceptible to injury from trauma. Frontal lobe and temporal lobe injury can also result in a change in personality and social interactions. The spectrum of personality change is highly variable between patients. Some will become socially disinhibited, manifesting vulgar behavior, irresponsibility, and aggression. Others may become abulic and apathetic. The clinical interview and obtaining a history from family members may assist with recognition of these changes in personality.
The upper motor neuron syndrome (UMNS) results from damage to the corticospinal tract. Its characteristic findings can be divided into positive or negative signs. Positive signs include increased muscle stretch reflexes, spasms, and co-contraction of agonist and antagonist muscle groups. This constellation of signs is often collectively referred to as “spasticity” and represents motor overactivity. The negative signs of UMNS include decreased force production and dexterity of movement. These signs are associated with loss of control of voluntary movement and represent motor underactivity. UMNS is a common finding in TBI. Approximately 25% of TBI patients will develop UMNS during inpatient rehabilitation. Given the diffuse nature of intracranial injury in TBI, the presentation of spasticity may be highly variable in this population. The presence of long bone fracture and peripheral nerve injuries can complicate the clinical picture of UMNS due to mobility restrictions and the flaccidity and areflexia of lower motor neuron injury. There exists a broad spectrum of treatment options for UMNS in brain-injured patients. These treatments include therapeutic modalities, splinting and casting, oral systemic medications, intrathecal therapy, and chemodenervation. For a more comprehensive review of UMNS and spasticity, refer to Chapter 6.
Computed tomography (CT) scan of the head is the initial imaging modality of choice in brain-injured patients. CT scan is able to quickly detect intracerebral hemorrhages, skull fractures, edema, and signs of increased ICP. CT is superior to magnetic resonance imaging (MRI) in detecting injury to the skull vault, whereas MRI has greater sensitivity for detecting intracranial abnormalities. In particular, MRI is superior to CT in the detection of diffuse axonal injury as well as injury to the brainstem, posterior fossa, and subtemporal and subfrontal regions. Findings of diffuse axonal injury may be absent or subtle on CT scan. Small petechial hemorrhages at the junction of gray matter and white matter in the corpus callosum may be seen on CT. MRI susceptibility-weighted images are useful for detecting areas of microhemorrhage associated with this injury. MRI is contraindicated in patients with pacemakers, metallic implants, ocular foreign bodies, and certain vascular clips.
Although not routinely available in clinical use, several advanced neuroimaging techniques show promise for assessment of TBI. Diffusor tensor imaging (DTI) is an MRI sequence used for evaluating the structural integrity of white matter tracts in the brain. DTI utilizes fractional anisotropy and apparent diffusion coefficient, which are measures of water diffusion in the brain. This technique can provide a roadmap of white matter tracts and their relative integrity. Unfortunately, fiber tracking algorithms are not well developed and lack sensitivity, and currently the primary use of DTI is as a research tool.
Magnetic resonance spectroscopy (MRS) is used to detect levels of neurometabolites post-TBI. Metabolites measured include N-acetylaspartate, creatinine, choline, glutamate, lactate, and myoinositol. It is beyond the scope of this chapter to discuss the variations in these metabolites in TBI. However, MRS is used to detect TBI when conventional neuroimaging is normal, to predict outcome, to assess severity of injury, and to determine the stage of brain recovery. It is currently limited to research applications owing to complexities of data interpretation, which are influenced by severity of injury, time of measurement, and location examined within the brain.
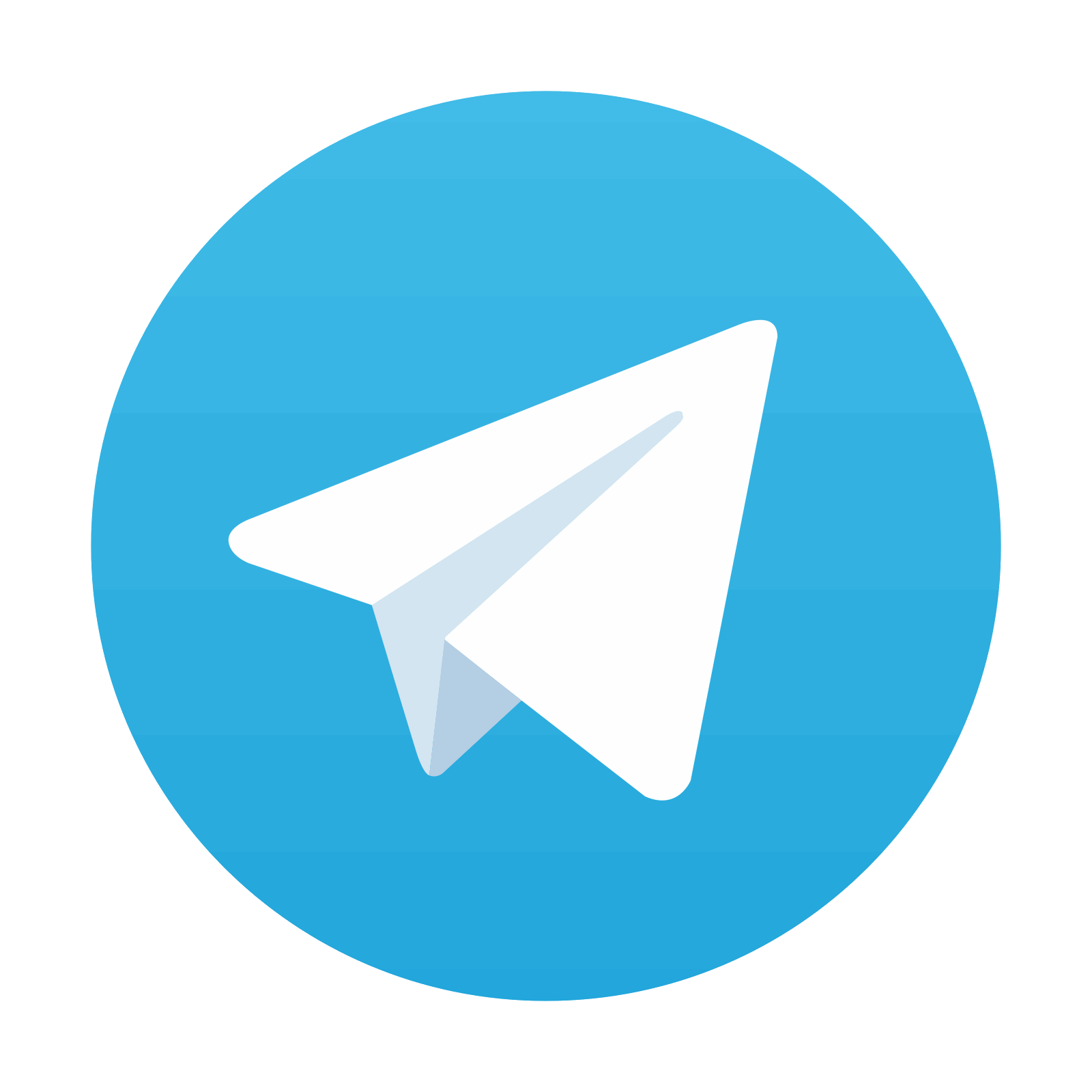
Stay updated, free articles. Join our Telegram channel

Full access? Get Clinical Tree
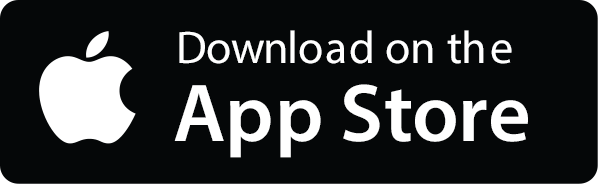
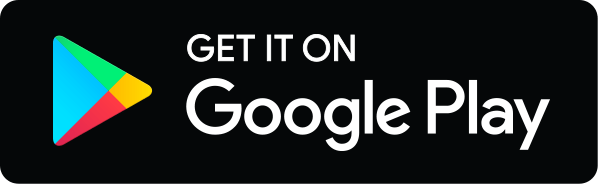