Tibial Shaft Fractures
Fractures of the tibial shaft remain an extremely common injury and are the most common long-bone fracture that orthopaedists treat, having a frequency of 26 fractures per 100,000 people per year. These fractures are approximately two to three times more common in males than females, with the average age of injury being 37 years.1,2 Motor vehicle accidents and sports injuries account for considerably more than half of these fractures, with high-energy mechanisms being associated with increasing comminution and open wounds.1,2 It has been estimated that closed tibial shaft fractures result in 77,000 hospitalizations, 569,000 hospital days, and 825,000 office visits per year.3
There are four accepted treatment methods for tibial shaft fractures: nonoperative treatment, plate stabilization, intramedullary nailing, and external fixation. The goal of each method is to adequately stabilize the tibia in an acceptable alignment while minimizing associated complications. The choice of treatment depends on balancing the relative risks and benefits of these four methods for each individual patient and situation. Although the focus of this chapter is on surgical treatment, nonoperative treatment remains an excellent option for some patients with certain fracture patterns. This chapter review these treatment methods and their surgical techniques.
Classification
The goals of fracture classification systems are to facilitate communication among surgeons, to guide treatment, to provide prognostic insight, and to assist in documentation. Although classification systems are conceptually simple, given the infinite variety of fracture patterns as well as the cumbersome application of the classification systems, their mediocre inter- and intraobserver reliability makes the realization of these goals difficult. Practically, however, tibial shaft fractures are still commonly classified by simple descriptive terms, such as fracture pattern, displacement, and location. The Arbeitsgemeinschaft für Osteosynthesefragen/Orthopaedic Trauma Association (AO/OTA) classification system is a formal radiographic comprehensive alphanumeric fracture classification scheme in which each bone is assigned a unique numerical designation.4,5 Using antero-posterior (AP) and lateral radiographs, fractures are then classified according to a basic framework (types A, B, and C) that suggests increasing energy, comminution, and fracture complexity. Each type can be subsequently subdivided into numerous groups. Type A fractures are unifocal, and the subdivision into groups is based on the orientation of the fracture line and the presence or absence of a fibula fracture. Type B fractures are wedge fractures and are subdivided into spiral bending or fragmented wedges. Type C fractures are more complex, and include complex spiral fractures, comminuted fractures, and segmental fractures (Fig. 33.1).

The magnitude of soft tissue injury is a critical element in the therapeutic decision making for tibial shaft fractures. Currently, two relevant classification systems are in widespread use for this purpose, one for evaluating the soft tissue envelope associated with closed fractures, and the other for evaluating open fractures. The soft tissue injury classification system of Tscherne and Gotzen6 is subjective and categories soft tissue injuries of closed fractures into four grades labeled 0 to 3 (Table 33.1).
The Gustilo and Anderson7 classification is based on the degree of soft tissue injury associated with open fractures, and progresses from type I to type III as the soft tissue injury increases. Type III wounds are commonly the result of high-energy mechanisms and have been subdivided into categories A, B, and C.8 Type IIIA injuries have extensive soft tissue damage but have adequate local soft tissue coverage. Type IIIB injuries demonstrate substantial periosteal stripping, contamination, and exposed bone that cannot be covered without local or distant soft tissue transfer. Type IIIC open tibia fractures are those that demonstrate the presence of an arterial injury requiring repair, and are at high risk for complications.
Because of the poor inter- and intraobserver variability demonstrated with the Gustilo and Anderson classification system, a newer open fracture classification system has been developed by Classification and Outcomes Committee of the OTA.9 This system classifies open fractures of the upper extremities, lower extremities, and pelvis, providing (1) greater stratification of injury severity, (2) better assessment of the factors that must be considered for treatment, (3) better distinction among the various injured tissues, and (4) optimal communication for clinical care and research. The OTA Open Fracture Classification is composed of five categories: skin injury (S), muscle injury (M), arterial injury (A), contamination (C), and bone loss (B). Each category is stratified into three subcategories ordered according to the extent of the injury: mild, moderate, and severe (Table 33.2). When critically evaluated, this newer classification system demonstrated moderate to excellent interobserver reliability, but the reliability in two key areas—muscle damage and contamination—remains unimpressive. Ultimately, it is hoped that a reliable open fracture classification system will facilitate uniformity in stratification of these injuries in both their treatment and research.10
Nonoperative Treatment
Nonoperative treatment of tibial shaft fractures with casting and functional bracing has a long history of success and was the standard of care for generations prior to the introduction of effective surgical methods. The end points that determine success, however, are subject to debate and continue to evolve. Despite several patients being lost to follow-up, Sarmiento et al,11 in 1995, reported on their extensive experience with functional bracing. Patients were selected for functional bracing if they had axially stable fractures (reduced transverse) or fractures with < 15 mm of initial shortening. The mean duration of treatment with a long-leg cast was 3.7 weeks, at which time they were transitioned to a prefabricated brace and encouraged to perform active ankle and knee range of motion and begin weight bearing as dictated by pain tolerance. Fractures healed at a mean of 18.1 weeks and the overall nonunion rate was 1.1%. Despite the majority of fractures having healed in a shortened position, only 10% demonstrated shortening of more than 1 cm. Approximately 50% of the fractures healed with a varus deformity, but only 5% had more than 8 degrees of varus angulation. Nearly all had less than 10 degrees of sagittal plane deformity. It was necessary to discontinue brace treatment in 2.4% of cases because of progressive angulation. In 1964, Nicoll12 reported on 705 cases of open and closed tibial shaft fractures treated nonoperatively in a long leg cast. He reported union time of ~ 16 weeks and a malunion rate of 8.6%. However, he noted a 25% prevalence of residual ankle and knee joint stiffness that increased to 70% in those who had nonunion associated with an open fracture.
Over time, the orthopaedic community has generally agreed that nonoperative treatment of selected tibial shaft fractures is associated with relatively high union rates, satisfactory alignment, and good function. Nonoperative treatment is appropriate for stable isolated fractures with alignment that can be controlled within 5 degrees in any plane, less than 1 cm of shortening, and < 50% translation. In these patients, nonoperative treatment remains an excellent option especially when compared with the complications associated with surgical options. Markers of instability include those fractures with increasing comminution, high-energy mechanisms, significant soft tissue injury (especially open wounds), and significant fracture displacements on presentation radiographs such as shortening greater than 1 cm and translations > 50% (Fig. 33.2). In these latter situations, nonoperative treatment is associated with higher nonunion and delayed union rates, difficulty with maintaining alignment within prescribed parameters, protracted immobilization, and joint stiffness regardless of whether long leg casts,13–17 patellar-tendon-bearing casts,18–20 or functional braces were used.11,21–23 Similarly, those tibial fractures with an intact fibula may be more prone to varus deformity or nonunion.24

Technique for a Tibial Shaft Fracture
Nonoperative treatment for a tibial shaft fracture typically begins with a manipulative reduction and application of a long leg cast. Adequate analgesia and sedation to allow manipulative reduction and casting can be obtained in the clinic or emergency room, but the entire process is simplified in the operating room under general anesthesia. Once the patient is sedated, the limb is placed off the edge or side of the bed in a flexed knee position, allowing the limb to hang dependently, utilizing gravity to aid in reduction. A trial reduction maneuver is performed and imaged with fluoroscopy to assess the adequacy of reduction. The limb is adequately padded, particularly around the malleoli and calcaneal tuberosity to avoid pressure points and skin compromise in these areas. Excessive padding, however, is to be avoided as this may contribute to loss of reduction. A short leg plaster cast is subsequently applied with the fracture maintained in a reduced position and the foot and ankle plantigrade (Fig. 33.3). In some distal tibial fractures, however, placement of the ankle in a neutral position may result in a recurvatum deformity at the fracture site. In this latter situation, the ankle can be maintained in a modest degree of plantar flexion, with the plan being to bring the ankle into a neutral plantigrade position over the ensuing several weeks as the fracture begins to stabilize.

As the short leg plaster cast begins to set, a mold is applied to the cast to aid in maintenance of the reduction. Biplanar fluoroscopy is performed and the adequacy of reduction is assessed. If the reduction is within the acceptable parameters that are described above, the cast is then extended into a long leg cast with the knee in a slightly flexed position. As the casting material sets, a supracondylar femoral mold is applied that will minimize pistoning of the cast on the limb and provide improved rotational stability at the fracture site. Patients are typically allowed touchdown weight bearing with the aid of crutches and are followed in the clinic weekly with radiographs and a clinical check.
Minor changes in angulation that occur within the first few weeks from the time of cast placement can be managed with cast wedging using open or closed wedging techniques. Closing wedge techniques have been associated with skin compromise, and therefore open wedge techniques on the concave side of the deformity are preferred. Cast wedging is performed by first creating a near-circumferential cast cut at the level of the deformity. The angulation is corrected manually and a cast spreader can be employed immediately opposite the apex of the deformity to maintain the opening wedge. A solid spacer, such as a piece of wood, cork, or stacked tongue depressors, is then placed in the defect left by wedging the cast open and the cast spreader removed. When repeat radiographs demonstrate the restoration of acceptable alignment, the cast and spacers are overwrapped with additional cast material.
The patient is typically transitioned into a functional brace 3 to 5 weeks after injury, provided that the radiographic reduction remains adequate and the acute symptoms have subsided. According to Sarmiento et al,11 the success of functional bracing is based on the philosophy that immobilization of the joints above and below the fracture is not necessary for fracture healing or maintaining alignment. Controlled motion allowed by the brace at the fracture site is conducive to osteogenesis. Constraint of the incompressible fluids of the surrounding soft tissue envelope by the rigid shell of the brace creates a hydraulic pressure-like effect that stabilizes the fracture. Patients are instructed to keep the Velcro straps of the brace secured and the brace fitting snugly throughout the day. Importantly, active joint range of motion and weight bearing are encouraged, as these activities result in dynamic compression of the fracture. Repeat radiographs are commonly obtained 1 week following transition into the functional brace to ensure that there is no loss of alignment. Thereafter, the patient is followed clinically and radiographically at monthly intervals. The patient is weaned out of the functional brace when pain with weight bearing and fracture-site palpation has minimized and there is radiographic progression toward union. Functional rehabilitation, gait training, strengthening, and return to preinjury activities are subsequently instituted.
Surgical Treatment
Indications
The classic indication for operative management of a tibial shaft fracture was the open fracture or the highly unstable closed fracture. However, changes in patient expectations have produced a decreased tolerance for malunion, non-union, and resultant functional deficits. It is clear that, when compared with nonoperative methods, unstable tibial shaft fractures treated with intramedullary fixation have a decreased rate of malunion, decreased time to union, minimized joint stiffness, and improved functional outcome.19 However, when considering a surgical option for the treatment of a tibial shaft fracture, the balancing of surgical risks and benefits should be thoroughly discussed with the patient, and the final decision should be made on a case-by-case basis. The vast majority of indications for operative treatment of tibial shaft fractures are relative and include the following:
Open fractures
Fractures associated with polytrauma or multiple injuries
Significantly displaced fractures, including shortening of > 1 cm, > 50% translation, > 5 to 10 degrees of malalignment in any plane
Fractures associated with compartment syndrome or requiring vascular repair
Fractures that have unacceptable alignment following manipulative reductions
Surviving the Night
Although the majority of tibial shaft fractures remain uncomplicated, several scenarios can occur that mandate immediate evaluation and management.
Dysvascular Limb
The presence of a tibial shaft fracture with altered vascularity most commonly occurs in the setting of an open tibia fracture, but may present with closed injuries. The initial presentation may demonstrate diminished or absent pedal pulses, a cool foot, and altered sensation. The ankle-brachial index (ABI) is a simple ratio of the blood pressure at the ankle compared with that in the arm, and has been demonstrated to be a useful bedside diagnostic test that can rapidly and consistently provide an objective measurement of blood flow. An ABI of < 0.9 has been demonstrated to be a highly sensitive threshold that suggests altered blood flow that warrants continued assessment and evaluation.25 The simplest therapeutic maneuver to perform in the setting of an abnormal ABI (< 0.9) is gross clinical realignment of the limb, followed by a repeated ABI. Subsequent to this, persistent measurements below 0.9 require an immediate vascular consultation, and treatment entails emergent vascular assessment and reestablishment of adequate blood flow. In the majority of situations, the treatment algorithm is as follows:
Exploration of the open wound (if present) or on-table angiography
Provisional restoration of distal flow with temporary shunting
Provisional skeletal realignment and external fixation to provide length, alignment, and rotation
Definitive revascularization with primary repair or bypass grafting
Prophylactic four-compartment fasciotomy of the leg and potentially fasciotomy of the foot
Delayed, definitive skeletal stabilization. This last step may occur immediately at the same operative setting, or days to weeks later depending on the viability of the limb, general physiology of the patient, and status of the soft tissue envelop.
Treatment of the dysvascular tibia commonly occurs in the setting of a mangled extremity, and limb salvage is a complex undertaking. A multidisciplinary team approach (vascular, orthopaedic, and plastic surgical services) is required with an objective appraisal of the potential for reconstruction, the anticipated functional outcome, and an overall physiological assessment of the patient (particularly other significant injuries or associated medical comorbidities, among other factors). Not infrequently, an immediate amputation is required.
Compartment Syndrome
One of the most challenging scenarios for the orthopaedic surgeon is the patient with a tibial shaft fracture and an evolving or fulminant compartment syndrome. The overwhelming majority of the orthopaedic community agrees on several aspects of compartment syndrome associated with tibial shaft fractures:
A missed compartment syndrome results in significant patient morbidity.
A missed compartment syndrome is a significant medicolegal issue.
The presence of compartment syndrome is best treated with an emergent and complete fasciotomy of the involved muscle compartments.
What remains ambiguous is the determination of a specific and predictive diagnostic threshold at which the diagnosis of compartment syndrome actually occurs. Current diagnostic parameters are imperfect26 and rely on clinical signs of neuromuscular ischemia (disproportionate pain at rest or with stretching of the musculotendinous units within the affected compartments, altered sensory and motor function of the nerves traversing the same compartments) and compartment pressure manometry. In the latter situation, compartment pressures within 30 mmHg of the diastolic pressure, or absolute compartment pressures of 30 to 40 mmHg have been recommended as the point where myoneural ischemia may begin to occur.27,28 However, the substantially high false-positive rate of these thresholds has also been demonstrated, and indicates that several unnecessary fasciotomies are obligatorily performed.26 Given the severe sequelae associated with a missed diagnosis, and until more specific diagnostic advances occur, the treating surgeon is invariably required to err on the side of caution.29 Once the diagnosis is established, treatment requires an emergent and thorough fasciotomy of all involved muscle compartments with skeletal stabilization.
Open Fracture
Definitive treatment of open tibial shaft fractures remains challenging, and while several principles should be adhered to, all fractures should be managed on a case-by-case basis. To minimize the risk of infection, delayed union, and nonunion, open tibial shaft fracture management should include the following:
Antibiotic prophylaxis with a first-generation cephalosporin and appropriate tetanus prophylaxis, administered as soon as possible
Bedside debridement of obvious contamination, wound coverage with a sterile bandage, and gentle limb realignment and splinting
Urgent operative debridement, irrigation, and fracture stabilization (need not be definitive at the time of the first surgery)
A complete evaluation of the zone of injury and a thorough excision of all nonviable tissue including devitalized bone fragments. The traumatic wounds are typically extending proximally and distally or supplemental counterincisions can be made to minimize further dissection within traumatized but viable skin. The proximal and distal bone ends should be delivered into the wound and cleaned of all contamination, including medullary debris.
Wound irrigation with several liters of sterile saline solution using low-pressure delivery mechanisms (gravity or low-pressure pulse lavage). In wounds with gross surface contamination, castile soap added to the saline solution may provide enhanced wound decontamination.
Skeletal stabilization with a locked medullary implant is currently the treatment of choice for the definitive fixation of open, diaphyseal fractures of the tibia.
Immediate primary closure of the open wound in fractures with an adequate amount of viable skin to enable tension-free closure. In clean wounds with significant soft tissue loss, a negative-pressure wound therapy device is applied; in wounds noted to have severe contamination, an antibiotic bead pouch is utilized to deliver high concentrations of local antibiotics. In both situations, microvascular or plastic surgery consultation should be sought and should result in soft tissue reconstruction within 7 days.
Significant bone defects that are likely to result in delayed or nonunion are managed with antibiotic polymethylmethacrylate (PMMA) spacers to preserve the potential space for bone graft or to induce a bioactive membrane at the site of bone loss. Staged bone grafting with autograft is performed once the soft tissue envelope has stabilized and all wounds have epithelialized, ideally within 4 to 6 weeks.
Currently, there is insufficient evidence to conclude that gram-negative or clostridial prophylaxis is beneficial in the routine management of open fractures.30 The duration of antibiotic prophylaxis is also controversial because there is no clear evidence that supports the use of such prophylaxis beyond 24 hours.30 Surgical debridement should occur as soon as the patient′s general physiological condition permits, but it remains controversial whether there is an absolute threshold for time-to-debridement that should be the goal. Although the time-dependent process of bacterial adhesion may be a critically important first step in the development of infection, numerous prospective and retrospective clinical trials have failed to convincingly demonstrate a relationship between infection and time from injury to debridement.31–35 Most recently, however, Hull and colleagues36 examined the relationship between delay to surgical debridement and deep infection in a series of 364 consecutive patients with 459 open fractures treat at an academic level one North American trauma hospital. The authors found a statistically significant increase in the rate of deep infection for each hour of delay. Specifically, delay had a larger absolute effect on the rate of deep infections on fractures of the tibia, fractures of a higher Gustilo-Anderson type, and those that were grossly contaminated. Interestingly, a large prospective study of severe open tibia fractures identified that the most important variable associated with decreased infection rates was timely transfer to a regional trauma center for definitive care.35 The consensus is that operative debridement should occur urgently (within 24 hours of injury) when the patient′s physiological status and operating resources allow.35,37–39 Although delayed treatment appeared safe for type 1 open fractures, Hull and colleagues recommend more urgent operative debridement when the negative prognostic factors of tibial site, higher GustiloAnderson fracture type, and/or contamination are present.18
Current evidence does not support the use of antibiotic solution for the irrigation of open fractures, and one randomized trial has shown a potential detrimental effect to wound healing with the addition of antibiotics to the irrigant.40 More recent data appears to favor the use of several liters of sterile saline solution with low-pressure pulsatile lavage in minimizing infection rates, nonunion, and woundhealing problems.41
Early skeletal stabilization of open tibial shaft fractures is an important component of protecting the soft tissue envelope from further damage. The method of stabilization has evolved over the years to favor intramedullary fixation. Plate and screw fixation has long fallen out of favor because of high complication rates, particularly wound breakdown and infection. Early studies reported infection rates of 20 to 40% in Gustilo type III open tibia fractures.42,43 A more recent systematic review examined 11 studies that evaluated 492 open tibial shaft fractures treated with plating.44 The overall union rate ranged from 62 to 95% and a pooled estimate of deep infection rate was calculated at 11%. This study noted that the results of plating are not nearly as dismal as previously thought, but that the exact circumstances need to be clarified with further studies. Newer percutaneous or minimally invasive techniques, particularly when combined with fixed-angle plate/screw devices, appear to limit iatrogenic soft tissue injury and may decrease infection rates as compared with previous studies. No study thus far has evaluated these methods in the treatment of open tibial shaft fractures.
External fixation offers three seemingly significant advantages over internal fixation in open tibia fracture management: (1) it enables rapid fracture stabilization; (2) the lack of metallic implants applied at the site of the open injury limits further soft tissue damage; and (3) minimization of foreign material (particularly metal) at the site of the open injury decreases the potential for bacterial adherence. A recent meta-analysis of patients treated with external fixation reported a union rate of 94%, but noted an overall 16.2% infection rate with 4.2% developing chronic osteomyelitis.45 The rate of pin-track infection approximated 30%, and 68.5% required at least one further operation to achieve union. This same study noted that intramedullary nailing (whether reamed or unreamed) demonstrated union rates of 95 to 97% and a 6.6 to 7% infection rate, with 30% of patients needing a reoperation to achieve union. More recently, a comparative analysis was performed examining the prevalence of complications of open tibial shaft fractures stratified according to the Gustilo-Anderson classification.46 This study noted a significant difference in deep infection rate and nonunion rate in type IIIB fractures as compared with types I, II, and IIIA, regardless of treatment method (external fixation, undreamed medullary nails, reamed medullary nails). In type IIIB injuries treated with a medullary nail, the deep infection rate was estimated to be 9.2% versus 19% in those treated with external fixation. The importance of this paper is to highlight the problem of the open type IIIB tibia fracture. A randomized trial of intramedullary fixation versus external fixation for open type IIIB tibia fractures has recently begun and may provide answers to this challenging injury.
The Multiply Injured Patient
A multiply injured patient with a tibial shaft fracture requires a careful assessment to avoid hampering ongoing resuscitative efforts and yet adequately manage the injured limb. Prolonged procedures in unor under-resuscitated multiply injured patients that do not significantly contribute to the resuscitation effort (cessation of hemorrhage or debridement of necrotic tissue) are to be avoided.47–49 The goals of “damage-control surgery” are to lessen complications arising from a result of the so-called second hit of increased acute surgical burden. Simple fracture patterns without significant soft tissue injury or swelling can be managed with noncircumferential splinting and repeated checks to monitor for compartment syndrome. In patients with high-energy injuries, significant soft tissue swelling, open wounds, gross displacement, or evolving compartment syndromes, the limb should undergo an expedient but appropriate debridement of open wounds, fasciotomy if required, and gross realignment with application of a simple uniplanar external fixator. Although this is preferably performed in the operating room, some patients who are too critically ill to be transported can be managed with bedside external fixation (and fasciotomy if required) in the intensive care unit. Once the patient has been resuscitated, conversion to definitive intramedullary nail can be performed with minimal risk of deep infection, particularly when performed within 14 days of external fixation application.50,51
Surgical and Radiographic Anatomy
The tibia has unique topographical and cross-sectional anatomy. The medullary canal of the tibial shaft is relatively straight in longitudinal dimension and relatively triangular in cross section. The straight longitudinal dimension is accessed through an anteriorly based proximal starting point for intramedullary fixation. The posteriorly angulated proximal bend seen on tibial intramedullary implants enables nail insertion via an off-axis starting point, akin to the proximal bend of a trochanteric femoral nail relative to the anatomic access of the femur.
The proximal and distal tibial metadiaphyseal areas are cancellous in nature and associated with cortical thinning and medullary cavity expansion. The thinner cortex in these areas causes external fixation pins to have less purchase than in the diaphyseal bone and risks loosening. The medullary cavity expansion seen both proximally and distally in the metaphyseal regions is clinically relevant for medullary implants because of the lack of cortical support for the nail; fractures occurring in these metaphyseal regions, therefore, are prone to malalignment when treated with medullary nails. Accurate tibial nail starting points are critical for minimizing angular deformity when nailing proximal tibial fractures, and for minimizing iatrogenic injury to intra-articular structures of the knee. Buehler et al52 noted that, in the frontal plan, a relatively lateral starting point on the superior tibia was collinear with the anatomic axis of the medullary canal through the isthmus. Other authors have also noted that a lateral-based starting point is an important component in minimizing frontal plane malalignment when nailing proximal third tibia fractures.53 Tornetta et al54 described the anatomic safe zone for tibial nailing, which minimized the risk to intra-articular knee structures, particularly the anterior horns of the menisci, and later provided the radiographic correlates (Fig. 33.4). This safe zone was noted to be immediately medial to the lateral tibial spine in the frontal plane, and immediately anterior to the articular surface as visualized on the lateral radiograph.55

Critical to the accurate radiographic identification of the tibial starting point is obtaining a true AP radiograph during this portion of the procedure. Walker and colleagues56 noted that significant alterations in the perceived starting point occur with tibial rotation. An accurate AP image demonstrates the fibula head longitudinally bisected by a line drawn tangential to the lateral margin of the lateral tibial plateau. Similarly, the location of the patella tendon does not reliably predict the location of an accurate starting point. Althausen et al57 noted that variability exists in the anatomic relationship between the patellar tendon and the lateral tibial spine, and that a routine peri- or transtendinous approach may not give direct access to the ideal nail entry site.
The blood supply to the tibia is derived from intramedullary and periosteal sources. The intramedullary source is derived from the posterior tibial artery via a nutrient branch. This nutrient artery enters the tibia in its proximal aspect via the nutrient foramina located at the posterolateral tibial cortex adjacent to the origin of the soleus.58 Upon entering the medullary cavity, it splits into three ascending branches, with one descending branch providing endosteal branches. After injury, and particularly during manipulation of the medullary canal, the endosteal blood supply is injured and the dominant supply to the bone is from the periosteum. In the uninjured tibia, the periosteal blood supply, derived from the anterior tibial artery, provides 25% of the bone′s vascularity. After injury, the bone may be 100% reliant on this source of blood supply. Thus, soft tissue or periosteal stripping produced by the injury or created surgically renders the tibia increasingly avascular. Considerable research has also noted that reestablishment of endosteal blood flow to preinjury proportions occurs 10 to 12 weeks after injury.59–65
Understanding the compartmental anatomy of the muscular and neurovascular structures, including their relationships relative to each other, is critical for managing tibial shaft fractures. Safe and effective fasciotomies, application of external fixation devices (particularly tensioned-wire fixators), and percutaneous screw insertions for submuscular proximal and distal plate applications are examples of situations when complete understanding of this anatomy is critical.66–70
Surgical Techniques
Intramedullary Fixation of Tibial Shaft Fractures
Video 33.1 Intramedullary Nailing
Today, the prevailing treatment method of unstable diaphy-seal tibial fractures is locked intramedullary fixation. With advancements in tibial nail design and fracture reduction techniques, tibial nailing has been expanded to also include fractures that have significant involvement of the proximal and distal metaphyseal portions of the bone, including those that may extend into the proximal or distal tibial articular surface (Fig. 33.5). Current sources of controversy include the relative risks and benefits of reamed versus unreamed nail insertion, the use of union adjuncts such as ultrasound stimulation or bone morphogenetic proteins, and the use of external fixation versus medullary fixation for the treatment of severely contaminated open tibial shaft fractures.

Provided that the cortical wall thickness is not excessively diminished, reaming of the medullary canal and use of a larger nail improves the biomechanical properties of the nailed fracture construct. This occurs by increasing the contact area between the nail and the smoothed endosteal surface of the bone, and more significantly, by inserting a larger-diameter nail. Depending on the nailing system that is utilized, larger diameter nails generally accept larger diameter interlocking bolts as compared with smaller diameter nails. The larger diameter interlocking bolts increase fracture construct stability because they are mechanically stiffer and are less prone to fatigue failure. The biological consequences of reaming the medullary canal have been studied in several animal models. Reaming has an immediate negative effect on cortical blood flow by destroying the endosteal blood supply. Although this may seem counterproductive to fracture healing, a compensatory and significant increase in the vascular perfusion of the surrounding soft tissue envelope, particularly the adjacent musculature
The decision to perform reamed or unreamed tibial nailing, therefore, is a theoretical trade-off between balancing the biological consequences of reaming with the mechanical benefits of a larger nail.59–65 Numerous high-quality studies comparing reamed and unreamed tibial nailing have been unable to clearly identify a significant benefit or detriment from either technique. A recent Cochrane review identified nine randomized and two quasi-randomized trials involving 2,093 patients.71 Using primarily outcomes of health-related quality of life, patient-reported function, and reoperation for treatment failure or complications, there were no statistically significant differences between reamed and unreamed nailing in “major” reoperations or in secondary outcomes of nonunion, pain, deep infection, malunion, and compartment syndrome. There appears to be some evidence that reaming is more likely to reduce the incidence of major reoperations related to nonunion in closed fractures, and implant failure (usually autodynamization from broken screws) occurs less often in the reamed group.
Currently, I use a minimally reamed technique for both open and closed fractures, because the placement of a medullary nail that accepts interlocking bolts ~ 5 mm in diameter may be less likely to result in breakage, avoids the potential for nail incarceration by using the reamer as a motorized sound to accurately identify the diameter of the narrowest portion of the canal, and avoids the creation of a distraction fracture gap that may result from using an unreamed nail that is passable, but too tight within the distal segment.
In 2008, the large multicenter trial of reamed versus unreamed tibial nailing (Study to Prospectively evaluate Reamed Intramedullary Nails in patients with Tibial fractures [SPRINT]) was published.72 Important findings from this study include the following: (1) a surprisingly low (4.6%) overall reoperation rate for nonunion (bone grafting or exchange nailing); (2) delaying reoperation for non-union for at least 6 months may substantially decrease the need for reoperation; and (3) there was no difference between reamed and unreamed nails in terms of reoperation to obtain union. However, there was a higher incidence of autodynamization due to interlocking screw breakage in the unreamed group in open fractures. If patients and clinicians see autodynamization as a negative event, then results support the use of reamed nailing for closed fractures. Subsequent to this, the authors published several prognostic factors for predicting outcomes after intramedullary nailing of the tibia.73 The authors found that the severity of injury clearly plays the most important role in outcome following intramedullary nailing of the tibia. High-energy fractures, fracture gap > 1 cm, and fractures needing soft tissue reconstruction (rather than primary closure) were all significantly associated with the occurrence of an adverse event (a surgical procedure to obtain union, removal of a failed implant, fasciotomy, or debridement of infection or hematoma). In this same study, autodynamization was also considered a negative event. Although the clinical importance of this is debatable, autodynamization may be reduced, in some instances, by not using stainless steel nails and by instructing the patient to avoid full weight bearing after surgery.
Successful tibial nailing begins with a careful assessment of the patient, the injured limb, and radiographic investigations. Full-length radiographs are obtained, including focused views on the knee and ankle to identify any associated fractures of the proximal or distal aspects of the tibia. The location, direction, and amount of displacement, and the magnitude of comminution are noted for the tibia fracture, and, if present, the fibula fracture. In distal tibial shaft fractures, associated medial or posterior malleolar fractures are not infrequent occurrences and their morphology can be further elucidated with computed tomography (CT) scanning. Abnormal radiographic relationships of the distal tibiofibular syndesmosis may signify associated rotational injury of the ankle joint, and wide displacements between the tibia and fibula may suggest substantial soft tissue disruption. Proximally, wide displacements between the tibia and fibula are of concern because of the possible involvement of the arterial trifurcation. Impediments to medullary nailing such as aberrations of the medullary canal, cortical medullary debris, and previous implants are evaluated.
Whether assessing an open or a closed fracture, the focus of the preoperative plan is on the method of achieving and maintaining reduction during reaming, nail insertion, and completion of interlocking. Numerous techniques are available including manual manipulations, indirect reductions using an external fixator or Large Distractor (Depuy Synthes, West Chester, PA), fibular open reduction and internal fixation (ORIF), percutaneous or open tibial clamp applications, and open reduction with short-length plating and unicortical screw fixation. All of these techniques are useful in isolation or in combination, but they require the surgeon to balance the potential biologically detrimental effects of the surgical footprint with the benefits of an increasingly accurate fracture reduction. The presence of an associated articular injury either at the proximal or distal aspects of the tibia requires a careful assessment and management plan (Fig. 33.6). When present, the epiphyseal fracture is invariably reduced and provisionally stabilized prior to medullary preparation and nailing. This creates an epiphyseal “block” that can accept and be interlocked into the proximal or distal aspect of the tibial nail. In these situations, the preoperative plan should (1) determine the adjunctive implants required for articular stabilization, (2) consider the potential for implant collisions and how this will be avoided, (3) include the requirement of adequate reaming or preparation of the epiphyseal portions of the tibia to avoid nail insertion with excessive force, and (4) identify a tibial nail with multiple interlocking options to ensure adequate fixation of the proximal or distal epiphysis.

Intramedullary Fixation of Diaphyseal Tibial Shaft Fractures
After successful induction of anesthesia, the patient is placed supine on a radiolucent table and prophylactic antibiotics are administered. Ideally, the distal end of the table should be free of any underlying supportive devices to provide the surgeon unimpeded access to the injured extremity. Full muscle relaxation should be administered as it substantially facilitates the surgical reduction and fixation. A soft lumbosacral support is used under the ipsi-lateral torso to enable placing the injured extremity in a neutral position. The limb is then prepared and draped with sterile technique and placed on an elevated foam ramp or pillow. A tourniquet is not applied. The image intensifier is located at the contralateral side of the table and the monitor placed so that the surgeon and assistant(s) can have an adequate view.
In many instances, particularly when utilizing an open technique or when applying an external fixator or Universal distractor as an indirect technique, reduction of the tibial shaft or fibula is performed with the leg in relative extension. Once reduction is obtained, the knee is subsequently flexed and the limb supported on a radiolucent triangular support. A lateral image of the tibia should be taken with the knee flexed prior to making the incision for the starting point. If the fracture site flexes or translates during knee flexion, special techniques for fracture reduction will need to be used during the procedure. These techniques are discussed later (see Proximal Metaphyseal Tibial Fractures, below). If the fracture flexes with knee flexion, but reduces in extension, a suprapatellar portal should be considered so that the tibia can be nailed with the leg in an extended position.
The surgeon may find it advantageous to use the image intensifier to assist in the placement of the skin and tendon incision. The medial and lateral borders of the patella, patella tendon, and the inferior pole of the patella are palpated, and the overlying skin is marked with a surgical marker. Using a true AP view, a pin can be centered over the desired starting point, and the relationship of this point to the tendon ascertained. Then the surgeon will have to decide whether to perform a medial, lateral, or transtendinous approach. For most proximal fractures, a lateral starting point is preferable. Subsequently, a 2-cm incision centered directly over the inferior pole of the patella is marked, and the skin, subcutaneous tissue, and prepatellar bursa are incised until the paratenon of the patella tendon is identified. Deep dissection then occurs according to the desired approach. If a transtendinous approach is used, the medial and lateral aspects of the tendon are identified and the paratenon and substance of the patella tendon are incised longitudinally along its length. The medial and lateral halves of the patella tendon are retracted and a guide pin introduced into the intervening space.
Once the bone is encountered, the desired tibial nail starting point is identified using proper AP and lateral fluorography. The tip of the guide pin is placed in the desired position, and then gently tapped into the cortex using a mallet, and an accurate guide-pin trajectory into the proximal tibia is then achieved. Invariably, this is collinear with the anterior cortex of the proximal tibial metadiaphysis on the AP and lateral fluoroscopic views. Avoiding a guide-pin trajectory that is too posteriorly directed is critical for avoiding several iatrogenic complications such as posterior cortical fracture, eccentric osseous reaming, and partial or complete reaming wire transection. These complications can be avoided with sufficient knee flexion and lateral fluoroscopic imaging during guide-pin insertion.
Once the guide pin has been inserted several centimeters, the starting point and entrance trajectory are enlarged using a cannulated small-diameter entry reamer designed to fit over the guide pin. Both the guide pin and entry reamer are subsequently removed. The surgeon then places a small bend approximately 0.5 to 1.0 cm proximal to the end of a straight ball-tipped reaming rod, secures the reaming rod to a T-handled chuck, and introduces the reaming rod into the proximal tibial entrance hole. The T-handled chuck enables the surgeon to easily turn, advance, and withdraw the curved ball-tipped guide rod. The curve at the distal end of the guide rod enables the wire to be steered more accurately through the tibia, particularly as the distal metaphyseal cancellous bone is encountered. The ball at the distal end of the ball-tipped reaming rod prevents the reamer heads from passing beyond the guidewire, and more importantly, enables the extraction of a broken or disengaged reamer head from the medullary canal. The ball-tip reaming rod is manually advanced through the proximal segment and delivered distally to the fracture site.
Using closed, open, or percutaneous techniques, the tibial fracture is then realigned to achieve anatomic length, alignment, and rotation, and the reaming rod is then advanced accurately into the distal segment, typically terminating at the physeal scar. The length of the tibial canal is then estimated using a radiographic ruler, or by using two reaming rods of the same length and subtracting the difference of the exposed portions. Reaming is initiated using a reamer size that is anticipated to be 2 to 3 mm less than the anticipated definitive nail size. Typically, the reaming begins with an 8-mm reamer and progress up in 0.5- to 1.0-mm increments until cortical “chatter” is obtained. There are several important surgical issues to consider during reaming. The reamer head can generate a significant amount of heat, and therefore reamers should be sharp and not inserted with significant force. A slow advance and an approach-withdraw technique helps minimize thermal injury and aids in keeping the reamer flutes clean. To avoid eccentric reaming and subsequent imperfect reduction and nail placement, the reamer should be advanced under power only through a reduced fracture. To avoid progressive inadvertent reaming of the tibial tubercle portion of the entrance hole, the reamers should be placed into, and withdrawn from, the starting hole manually with a slight posterior pressure to avoid anterior translation or elongation of the starting hole. It should also be remembered that the reamers will only ream to the ball of a straight ball-tipped guide rod or to the bend of the curved ball-tipped guide rod. Although this is not typically a problem, an area of unreamed dense epiphyseal cancellous bone may be encountered with terminal nail insertion that may result in distraction of the fracture site. If more distal reaming is required, the curved ball-tipped guide rod must be inserted slightly more distally or exchanged for a straight ball-tipped guide rod to enable more distal reaming.
To prevent the ball tip or the curved portion of the reaming rod from becoming incarcerated within the nail and proximal locking jig mechanism, the curved ball-tipped guide rod is typically exchanged for a straight non–ball-tipped nail insertion rod after the completion of medullary reaming. An exchange tube is utilized to prevent inadvertent, errant placement of the insertion rod. The definitive tibial nail is checked for accurate sizing and is assembled to the proximal locking jig. Prior to insertion, the accuracy of proximal locking is ensured by observing that the proximal locking sleeves and drills accurately target the proximal interlocking holes. The knee is once again appropriately flexed to enable the nail to be inserted as collinear with the anatomic tibial axis as possible. The nail is then placed over the insertion rod and delivered manually into the proximal tibia. Because the proximal tibial starting point and the anatomic axis of the tibia are not exactly collinear in the sagittal plane (unlike the piriformis fossa start point and femoral anatomic axis), the nail will course posterior toward the posterior cortex of the tibia. Adequate flexion of the knee enables the nail to smoothly pass along the posterior cortex until the nail becomes more aligned with the anatomic axis of the tibia as the isthmus is encountered. Gentle blows from a mallet should advance the nail along the insertion rod and the tibia with each strike. The fracture is maintained in a reduced position as the nail is passed into the distal segment. The insertion rod is typically withdrawn from the nail within 1 to 2 cm of the definitive nail position, and final seating is performed with AP and lateral views of the distal tibia and proximal tibia to ensure that the nail is not left proud.
Proximal interlocking is performed with the patient′s knee in flexion using the proximal locking jig, in accordance with the manufacturer′s instructions. The proximal locking jig and the radiolucent triangular support are then removed and the knee is then placed in relative extension on a supportive bump or ramp pillow. Distal interlocking is performed under fluoroscopic guidance using a freehand technique. For the vast majority of diaphyseal tibial fractures, I prefer two bicortical medial-to-lateral proximal and two bicortical medial-to-lateral distal interlocking bolts. At the conclusion of the procedure, extraneous clamps or provisional reduction aids are removed, final radiographic images are obtained, and a clinical assessment is performed to ensure satisfactory tibial length, alignment, and rotation and that the compartments of the leg are soft and compressible (Fig. 33.7). The surgical wounds are closed in layers, sterile dressings are applied, and the limb is subsequently placed into a below-knee splint with the foot and ankle in a plantigrade position. Postoperative care includes completion of 24 hours of prophylactic antibiotic administration, and frequent clinical examinations to monitor pain and appropriate use of analgesia. The limb is splinted for 1 to 2 weeks until swelling has subsided, the surgical wounds have sealed, and the patient is experiencing decreasing pain. During this time, active knee and toe range of motion is encouraged. After 1 to 2 weeks, the injured limb is placed into a removable prefabricated boot, and the patient is allowed active range of motion of the knee, ankle, subtalar, metatarsophalangeal, and interphalangeal joints. Partial weight bearing is instituted and gradually progressed to full weight bearing over the ensuing 4 to 6 weeks. Patients are typically encouraged to be full weight bearing by 6 weeks postinjury. Periodic radiographic and clinical examinations are performed to ensure satisfactory joint and muscle function, normalization of gait, return to employment/function, pain assessments and management, and radiographic progression toward union.

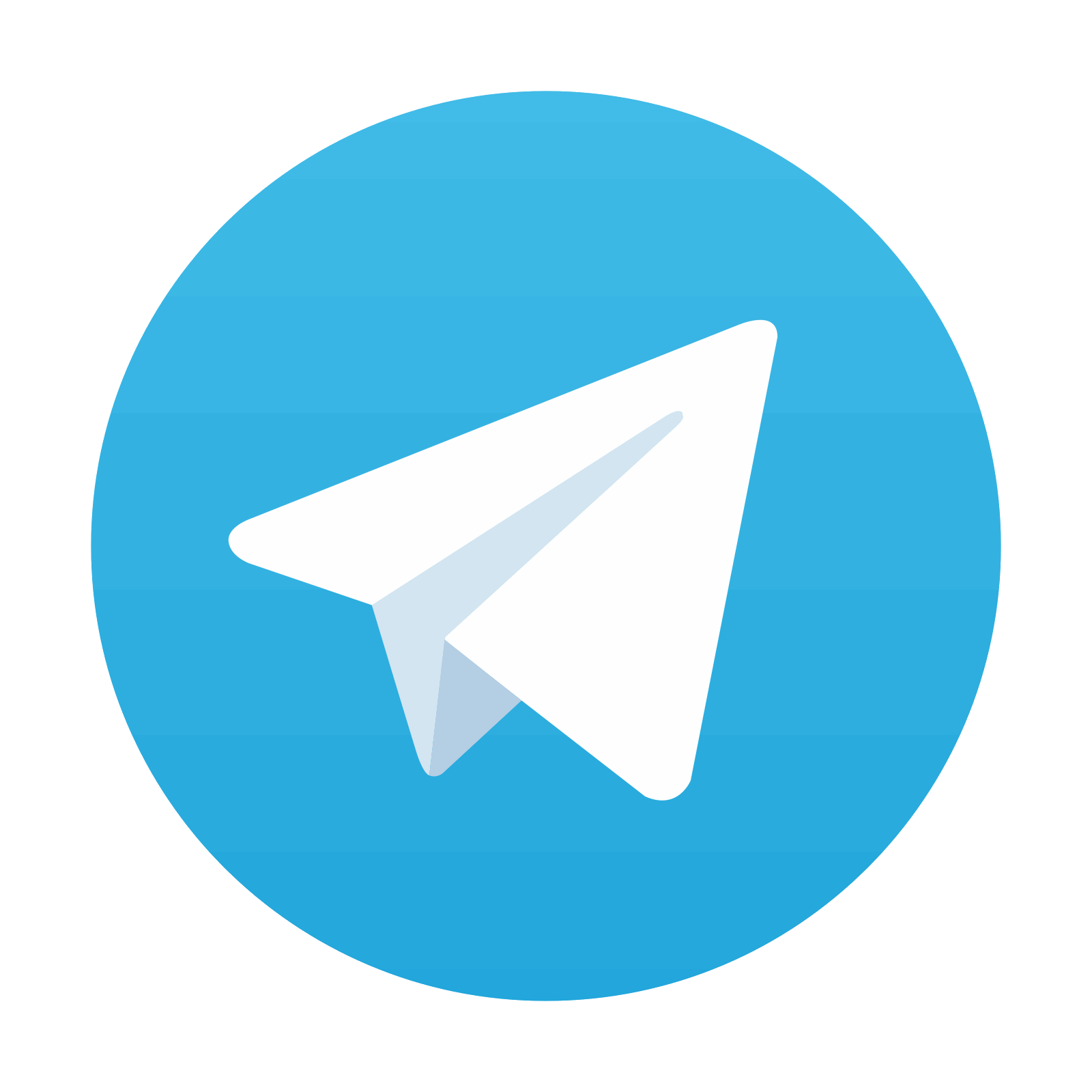
Stay updated, free articles. Join our Telegram channel

Full access? Get Clinical Tree
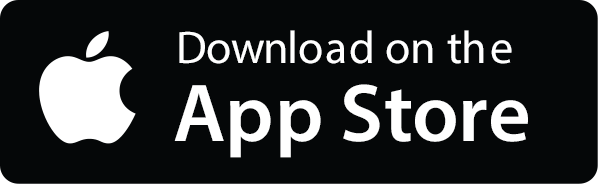
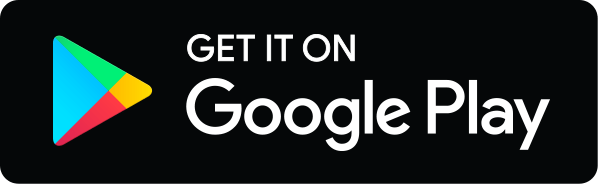
