Thyroid Gland
Rubina A. Heptulla
NORMAL THYROID DEVELOPMENT AND THYROID HORMONE SYNTHESIS
The thyroid gland first appears in the fourth week of gestation as an epithelial proliferation and invagination of the endoderm of the foregut, and the synthesis of thyroglobulin begins at this time. The thyroid then penetrates the mesoderm and migrates downward but remains connected to the floor of the foregut by means of the thyroglossal duct. This duct eventually becomes solid and, in normal individuals, involutes completely. By the seventh week of development, the now bilobed gland reaches its normal position anterior to the trachea. By the tenth to eleventh week, the fetal thyroid begins to trap and oxidize iodide, and by the twelfth week, colloid formation is detectable. By the eleventh week of gestation, the thyroid gland secretes thyroxine (T4) and triiodothyronine (T3). By the thirteenth week, thyroxine-binding globulin (TBG) and thyroid-stimulating hormone (TSH) are detectable in serum. Maturation of the fetal hypothalamic-pituitary-thyroid axis usually occurs by the twentieth week of gestation.
The synthesis of thyroid hormone is regulated through a central biologic feedback system. Under the stimulatory influence of the hypothalamic tripeptide thyrotropin-releasing hormone (TRH), also known as thyrotropin-releasing factor, the pituitary thyrotrophs secrete TSH. TSH enters the circulation and binds to specific receptors on the thyroid gland to stimulate production of T4. This stimulation occurs within the cell through a second messenger system, guanine nucleotide-coupled cyclic adenosine monophosphate production, a mechanism common to many peptide hormones. The circulating levels of T4 and T3 feed back at the level of the pituitary and the hypothalamus to regulate the release of TSH.
Thyroid hormone synthesis is a multistep procedure that begins when circulating iodide is trapped by the iodine pump and undergoes organification by thyroperoxidase. Organic iodide binds to the tyrosine residues of thyroglobulin, resulting in the formation of monoiodotyrosine and diiodotyrosine. Monoiodotyrosine and diiodotyrosine condense to form T3 and T4, the major thyroid hormones produced. T3 and T4 are stored on the thyroglobulin molecule in the form of colloid or are released from the thyroglobulin molecule into the circulation. Thyroglobulin itself normally is not released into the plasma and, if detected, may indicate a thyroid cell abnormality such as thyroiditis or carcinoma. Although the major thyroid hormone produced in and secreted by the thyroid gland is T4, its effects are exerted in peripheral tissues through its deiodination to the much more potent T3. The amount of T3 secreted by the thyroid gland itself adds a minimal fraction to the total circulating T3 under physiologic conditions. Inborn errors in thyroid hormone metabolism have been described for all of these steps.
The thyroid gland produces a third thyroid hormone, reverse T3 (rT3), which is synthesized from T4 by a deiodinase different from that which produces T3. Its physiologic role is unknown. The rT3 levels are high during fetal life, when T3 levels normally are lower than those in postnatal life. After birth, rT3 levels decrease markedly, and T3 levels increase into the normal range. In certain conditions, such as the euthyroid sick syndrome, the levels of circulating rT3 may be elevated.
T4 and T3 are transported in the plasma bound to the thyroid hormone-binding proteins TBG, the major binding protein, and thyroxine-binding prealbumin. Albumin also binds thyroid hormone with low affinity. The protein-bound fractions of circulating T4 and T3 account for more than 99% of the total hormone, and more than 70% of the total is bound to TBG. Even minor changes in the levels of the thyroid hormone-binding proteins can result in a significant change in the level of total circulating hormone, although the free (i.e., metabolically available, not protein-bound) hormone remains the same. For the same reason, TBG acts as a buffer to protect the tissues from fluctuations in T4 levels. As with inborn errors of thyroid hormone synthesis, many disorders of thyroid hormone-binding proteins, the most common of which is the X-linked recessive condition of TBG deficiency, have been described.
The physiologic effects of thyroid hormone are protean, accounting for the myriad symptoms that occur in thyroid disorders. These effects include protein synthesis, cell growth and differentiation, critical effects on the maturation of the central nervous system (CNS) and skeleton, maintenance of oxidative metabolism and heat production, and maintenance of cardiovascular function. The circulating levels of thyroid hormone also affect muscle tone, deep tendon reflexes, and maturation of the epidermis.
The thyroid gland also is the source of calcitonin, produced by the so-called C cells. These cells are embryologically derived from the neural crest and, thus, are not truly thyroid in origin. The physiologic effects of calcitonin oppose those of parathyroid hormone.
THYROID FUNCTION TESTS
Static Tests
The static tests of thyroid function include measurement of plasma levels of the various components of the thyroid system. Normal values for many of these components are given in Table 380.1.
Total T4 and free T4 concentration is determined by an immunometric assay that is the most widely used test of thyroid function and reflects the total of the protein-bound and free hormone. Total T4 is not a direct measurement of T4. Changes in serum-binding protein levels produce corresponding changes in total T4, although physiologically active free T4 is unchanged. This change results in abnormal serum total T4 levels in a physiologically normal patient. To overcome this obstacle, free T4 by equilibrium dialysis is used and is a direct measurement of free T4. Because the direct measurement of the active hormone accurately measures the free hormone level, it is
unaffected by serum-binding protein abnormalities or thyroid-binding protein excess or deficiency. However, this assay is expensive, time-consuming, and technically demanding, limiting its widespread commercial use. Total and free T3 concentrations are determined using the methods described for T4. In normal individuals, two-thirds of the T3 measured in plasma arises from peripheral deiodination of T4, and only one-third arises from direct secretion from the thyroid gland.
unaffected by serum-binding protein abnormalities or thyroid-binding protein excess or deficiency. However, this assay is expensive, time-consuming, and technically demanding, limiting its widespread commercial use. Total and free T3 concentrations are determined using the methods described for T4. In normal individuals, two-thirds of the T3 measured in plasma arises from peripheral deiodination of T4, and only one-third arises from direct secretion from the thyroid gland.
TABLE 380.1. THYROID FUNCTION TESTS IN INFANCY, CHILDHOOD, AND ADULTHOODA | ||||||||||||||||||||||||||||||||||||||||||||||||||||||||||||
---|---|---|---|---|---|---|---|---|---|---|---|---|---|---|---|---|---|---|---|---|---|---|---|---|---|---|---|---|---|---|---|---|---|---|---|---|---|---|---|---|---|---|---|---|---|---|---|---|---|---|---|---|---|---|---|---|---|---|---|---|
|
The T3 resin uptake (T3RU) once was used routinely to estimate the number of thyroid hormone-binding protein sites, reflecting the circulating level of free T4. T3RU is not a measure of plasma T3 concentration. Radiolabeled T3 is added to an aliquot of the patient’s serum and binds to all available (i.e., unoccupied) TBG binding sites. Because TBG has a greater affinity for T4 than for T3, the radioactive T3 does not displace the patient’s T4 from the TBG molecule. A resin that binds T3 is added, and the unbound radioactive T3 is taken up by the resin. This percentage increases as the number of available TBG binding sites decreases. The T3RU test is useful only if the total T4 concentration is known. The free thyroxine index, an estimation of the free T4 concentration, is calculated from the total T4 concentration and the T3RU using the following formula:

The T3RU and free thyroxine index have been replaced largely by direct measurement of free T4 concentration.
TSH levels are determined by an ultrasensitive immunometric assay and are helpful in the interpretation of thyroid hormone levels. The pituitary gland is exquisitely sensitive to changes in thyroid hormone levels, and elevated levels may indicate early thyroid gland failure or inadequate thyroid hormone replacement therapy before plasma thyroxine concentrations decrease below the normal range.
Measurement of TBG levels now is widely available, and this information is useful for interpreting abnormal thyroid hormone levels. TBG levels may be altered by a variety of conditions and medications (Box 380.1).
Many drugs may affect thyroid function test results because of interactions at various levels of thyroid hormone synthesis, release, and metabolism (Table 380.2). Obtaining a thorough history of medications is essential in the evaluation of a patient with an abnormality of thyroid function.
Immunoglobulin measurements helpful in the diagnosis of thyroid disorders include antithyroglobulin and antimicrosomal antibodies, which may be present in autoimmune disorders of the gland, and thyroid-stimulating immunoglobulin (TSI), which is important in the pathogenesis of hyperthyroidism. TSH-blocking antibodies can be measured at some centers and have been implicated in the pathogenesis of hypothyroidism.
BOX 380.1 Factors that Influence Thyroid-Binding Globulin Levels
Increased thyroid-binding globulin
Congenital (X-linked)
Hepatitis
Porphyria
Heroin, methadone
Estrogens, pregnancy
Oral contraceptives
Tamoxifen
5-Fluorouracil
Perphenazine (Trilafon)
Decreased thyroid-binding globulin
Congenital (X-linked)
Hepatic cirrhosis
Nephrotic syndrome
Androgens
Antiestrogens
Glucocorticoids
Nicotinic acid
Acromegaly
Protein-losing enteropathy
Protein-calorie malnutrition
Hyperthyroidism
TABLE 380.2. DRUGS THAT INFLUENCE THYROID HORMONE LEVELS | ||||||||||||||||||||||||||||||||||||||||||||||
---|---|---|---|---|---|---|---|---|---|---|---|---|---|---|---|---|---|---|---|---|---|---|---|---|---|---|---|---|---|---|---|---|---|---|---|---|---|---|---|---|---|---|---|---|---|---|
|
Dynamic Tests
The TRH stimulation test of TSH release is a helpful tool in the evaluation of patients whose thyroid hormone and TSH levels do not establish a diagnosis. In response to an intravenous infusion of TRH, the normal person has a brisk increase in serum TSH, followed by a slower return to the normal range. This pattern is accompanied by a similar increase and decrease in the serum prolactin concentration. The magnitude and timing of the TSH response to TRH help to differentiate normal from abnormal thyroid function. This test is useful in infants as well as older patients. An exaggerated TSH response occurs in primary hypothyroidism, whereas a blunted response occurs in hyperthyroidism. In addition, the TSH response to TRH may allow differentiation of central hypothyroidism caused by hypothalamic or pituitary dysfunction.
The T3 suppression test is helpful in differentiating hyperthyroidism caused by autonomous gland hyperfunction (i.e., TSI stimulation) from other causes. After a baseline of early radioactive iodine uptake has been determined, the patient is treated with exogenous T3 for 1 week, at which time the radioactive iodine uptake test is repeated. An autonomously hyperfunctioning gland does not show the normal decrease in radioiodine uptake.
Radiologic Studies
Radiologic studies of the thyroid gland involve the administration of radioactive isotopes, which are taken up by the thyroid gland and, to a lesser extent, by certain other tissues. Because the percentage of administered radioactive isotopes taken up by the thyroid gland is higher in infants and children than in adults and because the radiation risk to the thyroid gland is cumulative, the use of these studies for infants and children should be minimized. The commonly accepted indications for performing these studies in children include the evaluation of thyroid nodules, as an adjunctive test for elucidation of the cause of hyperthyroidism; localization of ectopic thyroid tissue; evaluation of dyshormonogenesis; and assistance in establishing the diagnosis when the combination of other static and dynamic tests of thyroid function is inconclusive.
Three radioactive isotopes are used commonly for thyroid studies. Iodine-131 results in the highest absorbed dose of radioactivity and has the longest half-life; its use generally is reserved for thyroid ablative therapy and for imaging studies in patients with thyroid cancer. Iodine-123 emits much less beta-radiation and is the isotope usually used for iodine uptake studies. Technetium (Tc)-99m pertechnetate results in the lowest dose of radioactivity, but once taken up by the thyroid gland, it is not further metabolized and, therefore, is not useful in studies of thyroid hormone metabolism and release. However, it can be used in the evaluation of thyroid nodules (i.e., hot versus cold) and goiters (e.g., homogeneous versus nonhomogeneous uptake; increased versus decreased uptake).
The 24-hour uptake of radioactive iodine (123I or 131I, depending on the clinical situation) is a common method for assessing the presence of thyroid tissue and for determining the daily iodine turnover of the thyroid gland. The daily turnover reflects the cumulative effect of thyroid clearance, renal clearance, thyroid retention, and dietary intake, and it is reported as the percentage of the total dose given.
In the early or 20-minute uptake test, the percentage of uptake for 20 minutes after administration of the intravenous dose of isotope is measured cumulatively. This uptake reflects thyroid uptake and organification of the circulating iodine load and is less affected by factors that modify the 24-hour uptake. Some patients, particularly patients with cancer preparing for a total body uptake study, are advised to consume a diet low in iodine before the test so that uptake of the iodine isotope is maximal.
The perchlorate or thiocyanate discharge test, which is performed after the 20-minute uptake, measures the amount of radioactive iodine that can be released from the gland after uptake. This test is useful for evaluating suspected dyshormonogenesis.
The T3 suppression test described earlier is helpful in establishing the diagnosis of hyperthyroidism. It also can be used as a method to detect relapses in Graves disease without interrupting the patient’s medical therapy.
Ultrasound of the thyroid gland can be used to differentiate solid from the more rare cystic nodules and may be as useful as is a low-risk screening procedure for this purpose. Ultrasound also is useful in differentiating thyroidal from nonthyroidal masses.
ABNORMAL THYROID CONDITIONS
Abnormalities of the thyroid gland may result from altered function (e.g., hypothyroidism, hyperthyroidism), altered structure (e.g., enlargement, nodule), or nonthyroidal causes (e.g., drugs, other illnesses).
Hypothyroidism
Hypothyroidism is defined as a state in which the thyroid gland fails to secrete sufficient quantities of thyroid hormone. Primary hypothyroidism results from a problem inherent to the gland itself, and secondary or central hypothyroidism results from the failure of pituitary stimulation of the thyroid gland. Primary and central hypothyroidism can be either congenital or acquired.
Congenital Hypothyroidism
Congenital hypothyroidism is a disease with an overall prevalence of approximately 1 in 4,000 live births, including 1 of 2,000 persons of Far Eastern or Hispanic descent, 1 of 5,500 persons of European descent, and 1 of 32,000 persons of African descent. Ninety-five percent of all cases are sporadic, and 5% are genetic, most often reflecting a dyshormonogenesis. It has a 2:1 female-to-male predominance, and associations with specific HLA types have been reported in certain populations. Newborn screening for congenital hypothyroidism is performed in all 50 states in the United States, but the methods of screening vary. Healthy, premature infants have lower T4 concentrations than do term infants of the same chronologic age, which must be considered when evaluating the results of the newborn screen. If the newborn screen blood sample is obtained within the first day of life, the TSH level may be falsely elevated because of the peripartum TSH surge.
Etiology
The causes of congenital hypothyroidism are considered here according to the types of hypothyroidism they produce.
Permanent primary hypothyroidism denotes irreversible failure of the thyroid gland to produce sufficient thyroid hormone. The most common cause is an ectopic thyroid gland, which results from improper migration during fetal development. It accounts for more than two-thirds of all cases detected by newborn screening worldwide. The ectopic thyroid tissue often can be demonstrated by Tc-99m scanning, and it is found most commonly at the base of the tongue (i.e., lingual thyroid). These aberrantly located glands do not function properly and do not produce an adequate amount of thyroid hormone.
The next most common cause of congenital primary hypothyroidism is hypoplasia, or aplasia of the gland, and this thyroid dysgenesis may result from the same pathophysiologic process, as does ectopy of the gland. In some cases, immunoglobulins have been implicated in the pathogenesis. A TSH-binding inhibitor immunoglobulin (TBII) has been described in sibling cases of nongoitrous neonatal hypothyroidism and in association with maternal chronic lymphocytic thyroiditis. TBII may be associated with a transient form of hypothyroidism in the newborn. TBII is detectable in both maternal and infant sera. Another antibody, known as thyroid growth-blocking immunoglobulin, has been associated with thyroid dysgenesis. It can block the growth of thyroid cells in vitro. Antithyroglobulin and antimicrosomal antibodies are not known to play direct roles in the pathogenesis of congenital hypothyroidism.
The third most common cause of congenital primary hypothyroidism is dyshormonogenesis, an inborn error of thyroid hormone synthesis, secretion, or metabolism. Dyshormonogenesis is responsible for most of the familial cases of congenital hypothyroidism. The most common form is a defect in the organification of iodide. Other causes of familial congenital hypothyroidism include deiodination defects, abnormal thyroglobulin, iodide trapping defects, abnormalities of the TSH receptor (e.g., familial unresponsiveness to TSH), and failure of thyroid hormone secretion. A rare peripheral resistance to thyroid hormone in which affected patients have clinical
hypothyroidism but elevated T4 levels and normal TSH levels that prevent neonatal recognition of the disease has been described.
hypothyroidism but elevated T4 levels and normal TSH levels that prevent neonatal recognition of the disease has been described.
Congenital hypothyroidism may result from maternal radioactive iodine treatment if it has been given after the eighth week of gestation, when fetal iodide trapping has begun.
Transient primary hypothyroidism is a self-limited process, and the cause is determined by a careful history. The causes include maternal iodine deficiency (e.g., endemic goiter), fetal or neonatal exposure to iodine (e.g., maternal medications, amniofetography, painting of the cervix, painting of the umbilical stump), maternal antithyroid drugs, maternal antibodies (e.g., TBII), and, rarely, association with the nephrotic syndrome (i.e., urinary loss of iodine). Transient hypothyroidism is more likely to occur in premature than in full-term infants. Although transient, some of these conditions may require temporary treatment with thyroid hormone. Hypothyroxinemia of prematurity (low T4 with normal TSH) is not understood clearly, but treatment with thyroxine generally is not recommended.
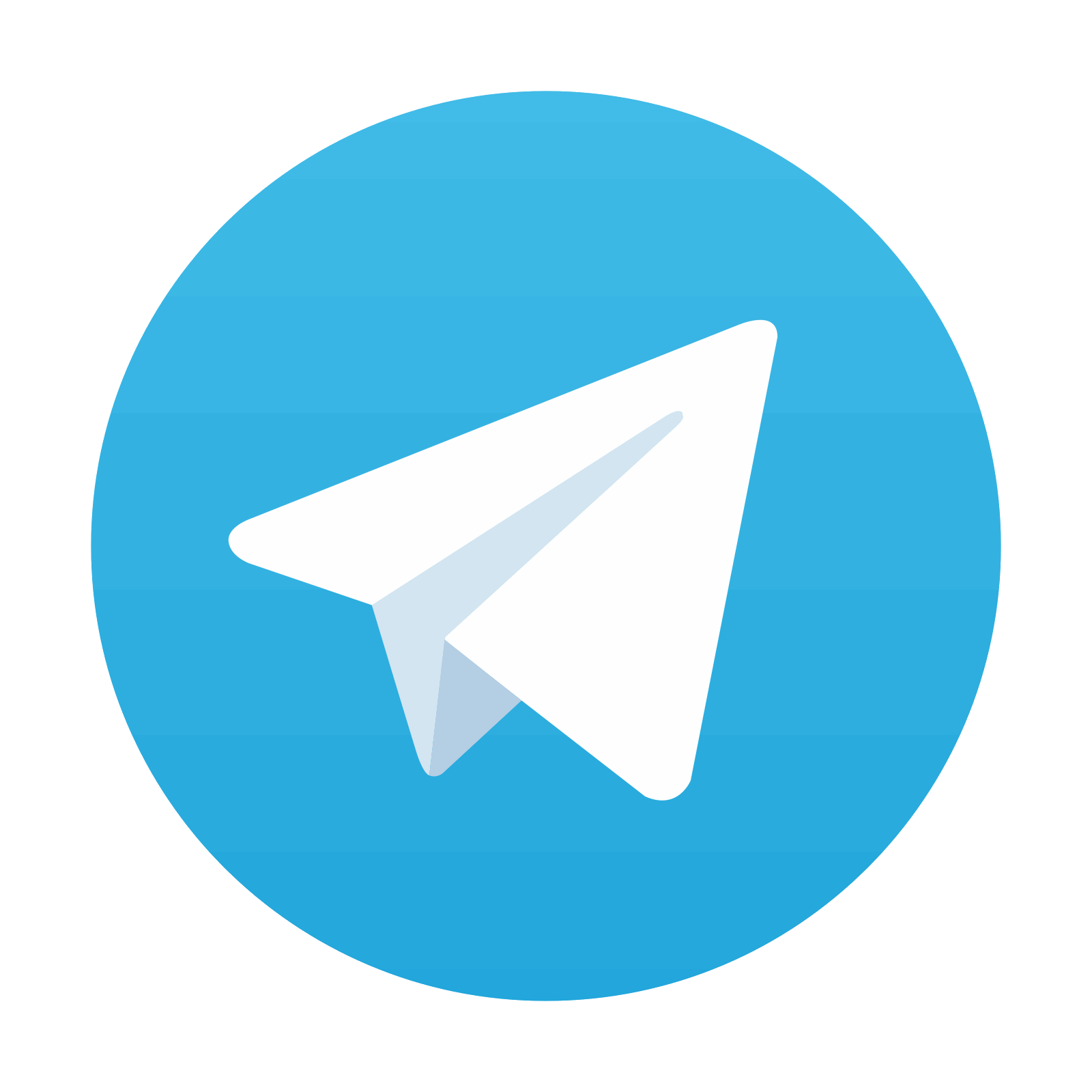
Stay updated, free articles. Join our Telegram channel

Full access? Get Clinical Tree
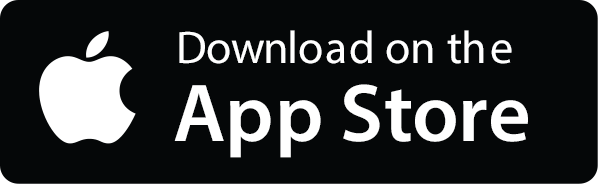
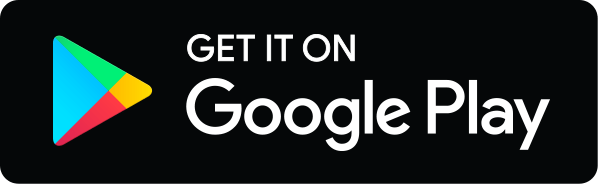
