Thoracolumbar and sacral fractures
Osteoporotic vertebral fractures
Traumatic thoracolumbar injuries
THORACOLUMBAR FRACTURES
Introduction
People’s life expectancy across the world has increased and the number of elderly in the population is expected to increase considerably in the years to come. In the United States, the fastest growing age group is those above 85 years and this segment of the population is expected to double by 2025.1 Osteoporosis is very common in the elderly population and the spine is the most common site for osteoporotic fractures.2 Injuries of the vertebral column in the elderly population are increasingly being recognized as an important healthcare issue. These fractures can be multiple and spontaneous and can result in significant morbidity and mortality. Adverse outcomes after spinal injury in elderly patients, including poorer chest function and increased in-hospital and post-discharge mortality, have been described. Patients who sustain an osteoporotic vertebral fracture are at a high risk of future fractures of the spine and the proximal femora. To prevent complications of inactivity it is essential that these patients are returned to functional activities as early as possible.
Management of vertebral fractures in the elderly requires a thorough understanding of geriatric physiology, the natural history of osteoporotic fractures and their treatment principles. Management can be complex because of their altered physiology, poor functional reserve, presence of comorbidities, cognitive dysfunction, polypharmacy and other factors. Although the majority of injuries can be managed conservatively, operative treatment is indicated in unstable fractures, polytraumatized patients, chronic painful post-traumatic deformities and pseudarthroses. Conservative care using bracing is cumbersome particularly because of poor bone quality, pre-existing spinal deformity, fragile skin, restrictive lung disorders, cognition abnormalities and restrictions in the usage of analgesics. On the other hand, surgical management is difficult for all the above reasons and the co-existing occurrence of osteoporosis and degenerative spinal diseases including diffuse idiopathic skeletal hyperostosis, ankylosing spondylitis and degenerative spondylosis which make instrumentation difficult. This chapter will focus on the injuries affecting the thoracolumbar and sacral regions in the elderly, with special emphasis on osteoporotic vertebral fractures.
OSTEOPOROTIC VERTEBRAL FRACTURES
Epidemiology
With improvements in preventive and therapeutic medical care life expectancy has increased throughout the world. The number of elderly people (aged 65 and above) has increased by approximately 1.7 million in the last 25 years.3 It is expected that 23% of the population will be aged 65 years and above by 2034.4 With increasing age, gradual bone loss due to osteoporosis, cognitive dysfunction, decreased muscle tone and medications that affect balance increase the risk of the elderly sustaining fractures, especially of the spine, hip and the wrist. The estimated lifetime risk of developing a spine, hip or wrist fracture after the age of 50 years is 40% in women and 13% in men.
Most of our knowledge regarding the demographics of osteoporotic fractures has been derived from studies of the Caucasian population. In the United States, it is estimated that approximately 10 million people suffer from osteoporosis and an additional 18 million have osteopenia.5 Approximately 1.5 million osteoporosis-related fractures occur in the United States each year. The most commonly affected regions are the vertebrae with approximately 750,000 fractures each year, wrist (250,000 fractures) and hip (250,000 fractures).6 Few studies have assessed the prevalence and incidence of vertebral fractures in non-white ethnic groups. It is notable that the estimated prevalence of vertebral fractures in Hispanic American or Japanese American women is approximately one-half of that of white women, and it is even lower in African Americans.7 Apart from the morbidity caused by these fractures, management of these fractures places significant socioeconomic stress on the family and society. In a study of the management of osteoporotic fractures, it was observed that approximately US$14 billion was spent on the care of patients in whom osteoporosis-related complications developed.6
Clinical features
Osteoporotic vertebral fractures typically occur at the thoracolumbar junction (T12–L1) and in the mid-thoracic region. Only about 30% of vertebral fractures are recognized at the time of injury because the diagnosis depends on the patient reporting back pain of sufficient severity to warrant a radiograph. Most of the remaining ‘clinically silent’ fractures heal without untoward sequelae but may also result in progressive vertebral deformities which may have a significant impact on health and quality of life. Gehlbach et al. observed that one or more asymptomatic vertebral fractures were noted in 132 (14%) of 934 chest X-ray films obtained in women age 60 years and older.8 The prevalence of radiographically identified vertebral deformities increases from 5% between the ages of 50 and 54 to 50% between the ages of 80 and 84.9
Most osteoporotic vertebral fractures occur following trivial day-to-day activities. In cases of severe osteoporosis, the cause of trauma can be as simple as coughing, stepping out of a bathtub, vigorous sneezing, lifting a trivial object or even sudden muscle contraction. In some patients with less severe osteoporosis, more force may be required such as falling off a chair or slipping on the floor. In the acute stage, there is pain in the affected region which is aggravated by activity. The pain may radiate along the thoracic or abdominal wall and is felt as a constricting band by the patient. The acute pain usually improves in 2–3 weeks and most patients are able to perform their daily activities once the acute pain subsides.
As the collapsed anterior part of the vertebral body heals, the spine gradually bends forward causing a kyphotic deformity. Multiple fractures may result in significant loss of height (Figure 31.1). Progressive kyphosis and sagittal imbalance results in shortening of the paraspinal musculature and imposes significant stress on the muscles resulting in muscle fatigue and pain. This chronic pain is typically worse with ambulation and relieved or improved with bed rest. The pain may continue long after the acute fracture has healed.
The kyphotic deformity also affects the general functioning of the patient. The increasing thoracic kyphosis is compensated for by exaggerated lumbar lordosis, retroversion of the pelvis and knee flexion (Figure 31.2). This abnormal posture places significant stress on the hip musculature resulting in early fatigue. With increasing kyphosis, the rib cage presses down on the pelvis, reducing the thoracic and abdominal space. In severe cases, this can lead to impaired pulmonary function, a protuberant abdomen and early satiety leading to weight loss. In a prospective study of osteoporotic fractures it was observed that the overall function declined in patients with vertebral fractures similarly to those with hip fractures. Apart from chronic pain, sleep disturbance, anxiety due to fear of falling, depression due to decreased mobility and lack of self-esteem, and poor quality of life are the other sequelae that follow vertebral fractures. Recent studies have observed a relationship between osteoporotic kyphotic deformity and gastroesophageal reflux disease (GERD) in the elderly.10 Although the exact mechanism is unclear decreased lumbar lordosis, poor sagittal balance, a high intake of oral medications and decreased back muscle strength were observed to be important risk factors for GERD.10
Figure 31.1 (a) Anteroposterior and (b) lateral radiographs in a 74-year-old patient who presented with chronic low back pain. The radiographs show a degenerative scoliosis and multi-level osteoporotic compression fractures that had resulted in global kyphosis.
Figure 31.2 (a) Anteroposterior (AP) and (b) lateral standing radiographs of an 80-year-old patient with multiple osteoporotic fractures of the thoracolumbar spine. Note the exaggerated kyphosis at the thoracolumbar region, straight lumbar spine, retroverted pelvis and a high positive sagittal balance. The soft tissue shadow of the abdomen shows the compressed abdominal contents and reduced thoracic volume.
Vertebral deformities are associated with a 2.8-fold increase in the risk of hip fracture and a fivefold increase in the risk of another vertebral fracture within 3 years.11,12 Early detection of vertebral fractures has tremendous importance because it has been shown that early treatment with anti-osteoporotic medications reduces the risk of future fractures of the spine and hip. Vertebral fractures are also associated with increased mortality. The reasons for increased mortality are not clear but are presumed to be due to pulmonary disease, decreased mobility and cardiovascular disease. In one study, vertebral fractures were associated with a 16% reduction in expected 5-year survival.13 A prospective study found that women with clinical vertebral fractures had an 8.6-fold increased 4-year mortality, compared with a 6.7-fold increase among those with hip fractures.14
Usually neurological symptoms are very rare, both in the acute stage and the chronic deformity phase. Because the majority of the damage is limited to the anterior column, the fracture is usually stable and rarely associated with spinal canal compromise. However a chronic kyphosis, with an unfused retropulsed bone fragment following an osteoporotic fracture can indent the spinal cord resulting in myelopathy in some patients. This post-traumatic vertebral collapse is described as Kummell’s disease and can result in delayed neurological deficit (Figure 31.3). The classic clinical presentation of Kummell’s disease includes a ‘trivial’ trauma to the spine where patients are essentially asymptomatic for weeks to months, but then develop instability type back pain, angular kyphosis and sometimes a neurological deficit. Radiographs show an area of bone destruction and radiolucency within the collapsed vertebral body. Kummell’s disease is considered to represent delayed, post-traumatic vertebral body collapse secondary to osteonecrosis.15
Investigations
In all patients with vertebral fractures, the clinician should consider secondary causes of osteoporosis such as osteomalacia, multiple myeloma, hyperthyroidism, hyperparathyroidism and renal failure. Initial tests should include a complete blood count, serum calcium, phosphorus, alkaline phosphatize and renal parameters. If clinically indicated, further testing should include serum 25-hydroxyvitamin D and parathyroid hormone levels, protein electrophoresis, thyroid function tests and levels of sex steroids.
Figure 31.3 Kummell’s disease. Anteroposterior (AP) and lateral radiographs (a,b) show a linear gas shadow within the fractured L1 vertebral body. Sagittal and axial T2 MRI images (c,d) show a corresponding fluid signal within the body indicative of osteonecrosis.
Plain anteroposterior (AP) and lateral radiographs are the initial images obtained for a suspected compression fracture. The AP view will show a decrease in the height of the vertebral body, mild widening of interpedicular distance and osteoporosis. The lateral view is diagnostic and shows compression of the anterior aspect of the vertebra resulting in the classic wedge-shaped vertebral body. Typical features include generalized osteoporosis or osteopenia and a fracture involving the anterior vertebral body with intact pedicles and posterior elements. Healed vertebral collapse may be seen in other vertebrae. Compression fractures usually occur at the thoracolumbar junction, usually at T8–L1 and rarely at T6–T8 and L2–L4. It is important to image the entire spine because 20–30% of vertebral compression fractures are multiple. In some patients, the acute fracture is not well visualized and serial plain films taken 2–3 weeks later can show the vertebral injury because the deformity can take days to weeks to develop.
Three broad types of vertebral collapse are described: anterior wedge, biconcave and crush deformities. Sugita et al. proposed a classification system for osteoporotic vertebral fractures based on their study of 135 fractures in 73 patients.16 The fractures were classified into five types based on the initial lateral radiographs. These are: (a) the swelled-front type, in which 50% of the anterior wall of the vertebral body is swollen; (b) the bow type, in which the anterior wall is pinched in and the endplate is falling in, resembling the bow of a ship; (c) the projecting type, in which 50% of the anterior wall of the vertebral body is projecting and which appears as a small bulge without a fracture line; (d) the concave type, in which the endplate is falling in and the anterior wall is intact; and (e) the dented type, in which the centre of the anterior wall of the vertebral body is dented and a fracture line is seen in the vertebral body. They observed that of the five types, the swelled-front type, bow-shaped type and projecting type fractures had a poor prognosis with late collapse and they often showed a vacuum cleft. On the other hand, concave type and dented type fractures had a better prognosis and almost always achieved fusion.
Computed tomography (CT) and magnetic resonance imaging (MRI) are not regularly performed. CT scans have been helpful in identifying fractures that are not well visualized on plain films, distinguishing a compression fracture from a burst fracture, demonstrating posterior vertebral wall integrity and in evaluation of the integrity of the posterior elements. CT also can reveal spinal canal narrowing and the presence of retropulsion of bone fragments (Figure 31.4). MRI is recommended when patients are suspected to have spinal cord compression or other neurologic symptoms. Malignancy should be considered as the first diagnosis in patients younger than 55 years with a compression fracture without trauma, or only minimal trauma. A complete past medical history including any treatment for malignancy or swelling in the neck or breast, should be taken and MRI should be part of the initial investigation in these patients (Figures 31.5 and 31.6). In osteoporotic fractures, the affected vertebra will appear bright in T1 and T2 images because of fatty replacement of the marrow. In acute fractures, a dark marrow oedema line can be seen in T1 images. Malignant vertebral collapse will appear dark in T1 and bright in T2 images. Contrast enhancement of the affected vertebra will be present. Another important differentiating feature is the status of the posterior cortex. In malignant collapse, the posterior cortex will be bulging into the spinal canal whereas in benign fractures, the posterior wall will appear intact or can have a sharp angulated fracture. Follow-up MRI in patients with persistent pain will show typical fluid signal within the vertebral body diagnostic of pseudarthrosis. Table 31.1 details the MRI features of both benign and malignant fractures.
Bone density studies are useful for evaluating the severity of osteoporosis and in advising patients of the likelihood of subsequent fractures. A T score of –2.5 indicates severe osteoporosis and warrants appropriate treatment as explained below. It is important to recognize that bone mineral density measured at the lumbar spine in those over 70 years of age may be falsely elevated due to end plate sclerosis, aortic calcification or spondyloarthropathy. In general, a low bone mineral density (T score <–1) is an independent predictor of fractures and hence it is recommended that all women aged 65 years or older, regardless of additional risk factors, have a bone mineral density measurement. A nuclear medicine bone scan is useful when surveying the entire skeleton for osteoporotic fractures, especially when symptoms are atypical. It is particularly helpful in diagnosing sacral insufficiency fractures, which are common in osteoporosis but difficult to visualize on radiographs. Bone scans also can differentiate between an acute or healed compression fracture because new fractures will appear as ‘hot’ spots.
Figure 31.4 (a) Anteroposterior (AP) and (b) lateral radiographs depict an osteoporotic compression fracture of T12. (c) Sagittal and (d) axial CT shows posterosuperior corner retropulsion of the fractured vertebra and an intravertebral vacuum phenomenon.
Figure 31.5 (a) Sagittal T2 images show iso- to hypointense signals, (b) T1 images show hypointense signals with a bulging posterior vertebral cortex. (c) Axial contrast enhanced images show enhancement and involvement of the pedicles by the tumour infiltration.
Figure 31.6 Sagittal (a) T1 MR image shows hypointense signals, whereas the (b) T2 image shows an isointense body with an intact posterior vertebral wall suggestive of a benign fracture.
Management
The care of patients with vertebral fractures includes pain management, rehabilitation and prevention of further fractures. Acute pain due to vertebral fractures may last 2–3 weeks but rarely beyond 10 weeks. Most compression fractures are stable and can be treated by conservative methods. Patients are treated with a short period, of no more than a few days, of bed rest. Prolonged inactivity should be avoided, especially in elderly patients.
Oral analgesics are administered for pain control. Standard analgesics include acetaminophen 50–60 mg/kg body weight per day in divided doses, tramadol 50 mg/dose, codeine 30 mg/dose and non-steroidal anti-inflammatory drugs (NSAIDs). Acetaminophen is avoided in patients with hepatic derangement. Opiate analgesics can cause constipation, gastric distension, nausea, vomiting and drowsiness, which are discomforting and potentially harmful in the elderly patient. NSAIDs (diclofenac, aceclofenac, ibuprofen, ketorolac) provide good analgesia but can be potentially nephrotoxic and induce gastritis. These are used judicially in consultation with a geriatrician and based on the patient’s analgesic needs. Generally, analgesic needs can be met with therapeutic doses of acetaminophen and breakthrough pain can be managed with codeine 30–60 mg every 6 hours.
Calcitonin, by subcutaneous or intranasal administration, can be beneficial in reducing pain from acute vertebral fractures. It is recommended that a calcitonin dose of 50–100 IU be given subcutaneously, or 200 IU intranasally, for pain due to vertebral fractures. Several short-term randomized trials have demonstrated that calcitonin has a rapid analgesic effect.17,18 Physical modalities for pain relief such as heat, cold and ultrasound and massage therapy are used for osteoporotic fracture pain but have not been studied for efficacy.
With progressive kyphosis, the patient’s centre of gravity shifts forward which can affect the normal gait pattern. Walking aids such as four-post walkers, elbow crutches or walking sticks can provide stability and help in preventing falls. Controversy exists regarding the role of bracing. A hyperextension orthosis or a thoracolumbar sacral orthosis (TLSO) is usually prescribed for these fractures, as it is believed to offer pain relief, correct posture and help immobilization of the spine. However various authors have shown that external support has no mechanical stabilizing effect on the spine.19,20 In a systematic review, Giele et al. concluded that there is no evidence for the effectiveness of bracing in patients with traumatic thoracolumbar fractures.20 If used chronically, bracing may lead to weakness of the paravertebral muscles and cause disuse osteoporosis and worsening of back pain.
Table 31.1 MRI differentiation of benign and malignant fractures in the osteoporotic spine
Once the acute pain improves, patients are advised to undertake core strengthening exercises. Exercise programs for elderly patients after a vertebral fracture have demonstrated decreased use of analgesics, improved quality of life, and increased bone mineral density. A randomized controlled trial in elderly women with vertebral fractures found that an exercise program consisting of balance and muscle strengthening exercises, resulted in reduced use of analgesics and pain level.21 Most patients perceive significant improvements in their pain and functional capacity after 6–12 weeks and can return to a normal exercise program once the fracture has fully healed. Regular activity and muscle strengthening exercises have been shown to decrease future vertebral fractures and chronic back pain.22
In 20–30% of patients, the fracture may not heal completely, resulting in painful pseudarthrosis. Such patients who continue to have severe pain and who do not respond to conservative treatment may be candidates for percutaneous vertebral augmentation procedures such as vertebroplasty and kyphoplasty. Percutaneous vertebroplasty involves injecting acrylic cement into the collapsed vertebra to stabilize and strengthen the fractured vertebral body. This procedure does not restore the shape or height of the compressed vertebra. Kyphoplasty involves an initial insertion of an inflatable balloon through the pedicle into the vertebral body to re-expand the collapsed vertebra. With these procedures, pain relief has been reported in 60–100% of cases.23,24 The procedures can be performed under local or regional anaesthesia as a day case procedure.
To assess the safety and efficacy of vertebroplasty for osteoporotic fractures, Buchbinder et al. performed a multicentre, randomized, double-blind, placebo-controlled trial in which participants with one or two painful osteoporotic vertebral fractures that were of less than 1-year duration were randomly assigned to undergo vertebroplasty or a sham procedure. Out of 78 participants, they observed that vertebroplasty did not result in a significant advantage in any measured outcome at 1 week or at 1, 3 or 6 months. There were significant reductions in overall pain and also improvement in rest pain, physical functioning and quality of life in both study groups.25 In another study by Kallmes et al., 131 patients, who had one to three painful osteoporotic vertebral compression fractures, were assigned to undergo either vertebroplasty or a simulated procedure without cement (control group). The authors observed that at 1 month, there was no significant difference between the vertebroplasty group and the control group in either the disability score or the pain rating. Both groups had immediate improvement in disability and pain scores after the intervention. The authors also noted that there was a trend towards a higher rate of improvement in pain in the vertebroplasty group (64% vs 48%, P = 0.06) and at 3 months, there was a higher crossover rate in the control group than in the vertebroplasty group (51% vs 13%, P<0.001).26 While these two randomized trials questioned the efficacy of vertebroplasty for osteoporotic fractures, the methodology of the trials was criticized by Boszczyk.27 He observed that the results of these trials contradict previously published clinical series on vertebroplasty which have shown good clinical efficacy. He analysed the procedural details of the two randomized controlled trials (RCTs) specifically with regard to injected polymethylmethacrylate (PMMA) volumes which was an average fill volume of 2.8±1.2 mL per level. He noted that the existing data indicate that a minimum fill volume of 13–16% of the vertebral body volume is necessary for a relevant biomechanical effect on restoration of vertebral strength. This would be a minimum of 4 mL PMMA for any thoracic or lumbar vertebra (average 30 mL volume). Hence he concluded that the treatment arm of the two studies included patients who were not treated in a reasonably effective manner and hence the information provided is insufficient to conclusively disprove the clinical efficacy of vertebroplasty.27
Vertebroplasty
Although percutaneous vertebroplasty was performed first by Galibert and Deramond in 1987, the technique only gained popularity in the treatment of osteoporotic vertebral fractures in the last decade. The procedure can be performed under local or general anaesthesia. The patient is positioned prone on a series of soft pillows placed to support the head, chest, pelvis and the knee joints or on two longitudinal bolsters under the chest and pelvis. A radiolucent table is mandatory and after positioning the patient, AP and lateral images are taken to check the adequacy of the imaging.
The procedure starts with an AP image of the target vertebra. The arm of the fluoroscope is tilted cranially or caudally until the vertebral endplates of the involved vertebra are seen parallel to each other. The arm is then rotated in the medio-lateral plane until the spinous process of the affected vertebra is seen exactly midway between the two pedicles. A small skin incision is made horizontally starting from the lateral wall of the pedicle and extending laterally along the transverse process. A Jamshidi needle is then passed through the fascia and the paraspinal muscles, with medial angulation towards the spine. The needle tip is aimed towards the lateral pedicle wall in the upper lateral quadrant of the pedicle eye and confirmed with an AP image. Under fluoroscopy guidance, the needle is gently tapped into the pedicle with slight medial angulation until the medial pedicle wall is reached. A lateral fluoroscopy image is now taken to locate the position of the needle tip. When the tip is at the lateral margin of the pedicle in the AP view, the tip of the needle should be at the posterior point of the pedicle in the lateral view. As the needle progresses to the centre of the pedicle in the lateral view, the tip of the needle must be seen in the centre of the pedicle in the AP view. When the tip has crossed the pedicle and enters the vertebral body in the lateral view, the tip of the needle can cross the medial margin of the pedicle in the AP view (Figure 31.7). Once the needle is confirmed to be in the ideal position (anterior one-third of the vertebra in the lateral view and the middle of the vertebra in the AP view), cementing can be started to perform a unipedicular vertebroplasty. If the needle is lateralized on the AP view, then a bipedicular vertebroplasty (Jamshidi needle into both pedicles) is performed to ensure an adequate fill. A biopsy should be performed in case of suspected pathological fractures.
When the cement has the consistency of toothpaste, it is injected through the needle under continuous lateral fluoroscopic control in order to observe and prevent any cement leakage (Figure 31.8). By adjusting the position of the needle, one can avoid cement leakage into the disc space. If there is any leakage into the canal cementing should be stopped. The cement is allowed to set for some more time and the needle is re-directed to start cementing. If there is any further leakage, then cementing should be abandoned.
The actual volume of cement to be injected is not clear. While small amounts of cement (<2 mL) will have no appreciable effect, excessive cement can harden the vertebral body beyond its original state and may have an adverse effect on adjacent osteoporotic vertebrae. Biomechanical studies have shown that the minimum volume required for achieving restoration of compressive strength or stress distribution ranges between 13 and 16% of the original vertebral body volume.27,28 The average vertebral body volume is estimated to range from 12 mL, at T6, to 45 mL at L5 depending on the size of the vertebral body.27,28 This would indicate that for the 16% cement fill required to restore vertebral strength, a minimum of 2–3 mL is required in lower thoracic vertebrae and 3.5–7 mL is required in the thoracolumbar and lumbar vertebrae.
If there is a need for vertebroplasty at more than one level, caution should be exercised to avoid injecting more than 20–30 mL cement in a single stage. Augmentation of more than one vertebral body can be associated with fat embolism from the vertebral marrow. The reasons for embolism are probably related to the increase in intraosseous pressure during cement injection forcing the bone marrow contents into the circulation. In a retrospective study of 78 patients, Kaufmann et al. reported a significant drop in oxygen saturation for 10 minutes after vertebroplasty.29 Based on this, it has been recommended that the maximum volume of injected cement should not exceed 30 mL or three levels per session.
Figure 31.7 Technique of vertebroplasty. (a–c) The ideal needle entry point is in the upper and lateral corner of the pedicle. Medial angulation is maintained as it traverses the pedicle. When it reaches the medial pedicle wall, it should have crossed the posterior vertebral border. (d) Possible error 1: The needle has been inserted with less medial angulation. In the anteroposterior (AP) view, it is still well away from the medial pedicle wall but in the lateral view, the tip is already beyond the posterior vertebral wall. (e) Possible error 2: The needle has been inserted with too much medial angulation. In the AP view, the tip is touching the medial pedicle cortex but in the lateral view, the tip has not traversed the pedicle fully. This is a dangerous error as it is associated with a risk of canal breach and neurological complications.
Figure 31.8 Management of a painful osteoporotic vertebral fracture with bipedicular vertebroplasty. The anteroposterior (AP) and lateral radiographs depict a compression fracture of the L1 vertebra (a,b). Sagittal T2 and T1 images (c,d) show oedema and collapse of the L1 vertebra. The patient was treated by vertebroplasty. Note the good fill of the cement within the body in the AP and lateral views (e,f).
Vertebroplasty in the acutely fractured vertebral body is not advised for two reasons: first because of the favourable outcomes with conservative care in most patients and second the high risk of cement leakage in the presence of acute fractures. Use of continuous fluoroscopy while injecting the cement and using the cement only when the consistency has become like toothpaste can reduce the chances of cement leakage. In patients with a suspected defect in the posterior vertebral wall, a small amount of contrast dye can be injected into the vertebral body to look for extravasation into the spinal canal. The cement diffuses into osseous space and after it solidifies it stabilizes the vertebral body. Pain relief is enabled by two probable mechanisms. First, the cement combines the individual bone fragments in a single block avoiding the painful micro-movements between individual fragments. The second mechanism may be related to the exothermic process that accompanies the polymerization of PMMA which results in ‘thermal neurolysis’ of the painful nerve endings within the vertebral body. In addition, the PMMA results in significant strengthening of osteoporotic bone, reducing the risk of subsequent fractures.
After vertebroplasty, it has been reported that a marked improvement in pain symptoms can occur in 90% of cases. But the procedure can be associated with complications including bleeding at the site of needle insertion, transient fever, cement leakage into the disk or into the paravertebral soft tissues, epidural space or paravertebral veins, leading to pulmonary embolism, new fractures in adjacent vertebrae, infection and rarely cerebral embolism and even death (Figure 31.9). The incidence of complications ranges from 1% to 3%.30 Cement leakage into the spinal canal is the most feared complication. Although leakage of cement into the spinal canal is asymptomatic in most cases, it can potentially lead to serious neurological complications (Figure 31.10). Although intraforaminal leakage is less common, it can be more bothersome for the patient. In a study by Cotten et al., it was found that while leakage from the spinal canal was well tolerated, two of the eight patients with foraminal leakage had significant radiculopathy. In most cases, the symptoms are transient and respond well to nerve-root blocks or oral medication.31
A number of reports have indicated an increased risk of secondary vertebral fractures adjacent to the augmented vertebra.32,33,34,35 and 36 Several factors have been considered to be risk elements for developing adjacent vertebral level fractures. These include the degree of augmentation of the treated vertebra (i.e. more cement fill), location of the adjacent vertebra at the thoracolumbar junction, leakage of cement into the disc space and a decreased vertebral stiffness secondary to severe osteoporosis. Lin et al. studied 38 patients who underwent vertebroplasty over a 12-month period and observed that vertebral bodies adjacent to a disc with leakage of cement had a 58% chance of developing a new fracture compared with 12% of vertebral bodies adjacent to a disc without leakage.37 Leakage into the disc can restrict the flexibility of the adjacent vertebral body and thus may increase the risk of a secondary fracture. This factor appears more important in vertebrae where the initial strength of the vertebral body is very low. Heini et al. and Tomita et al. observed that the lower the initial bone mineral density, the more pronounced the augmentation effect.38,39 and 40 In strong non-osteoporotic bone, cement augmentation does not produce any significant changes.
Other authors have suggested that adjacent fracture risk is part of the disease process as patients with vertebral fractures develop fractures at adjacent levels even while on conservative treatment. The rates of new fracture after cement augmentation procedures vary between vertebroplasty (0–52%), kyphoplasty (5.8–36.8%) and conservatively treated patients (19.2–58%) but these are not comparable due to the varied scientific designs of the studies.41 Several authors have suggested that kyphoplasty could reduce the risk of adjacent fractures. With the development of a kyphotic deformity, the body’s centre of gravity shifts forward resulting in an increased forward bending moment and may predispose the adjacent vertebrae to have secondary fractures. Hence partial reduction of kyphotic deformity with kyphoplasty can decrease the risk of new fractures.42 In a comparative study, Kasperk et al. found that at a mean follow-up of 6 months, seven (35%) out of 20 conservatively treated patients developed secondary fractures, while 12.5% of 40 patients who had undergone kyphoplasty developed adjacent level fractures.42
Figure 31.9 Complication of vertebroplasty. The patient presented with a T12 osteoporotic collapse as seen in the anteroposterior (AP), lateral and sagittal MR images (a–c). Despite vertebroplasty (d,e), the patient had severe pain postoperatively and a repeat MRI (f) revealed a frank pseudarthrosis at T12 and incorrect cementing at the L1 level.
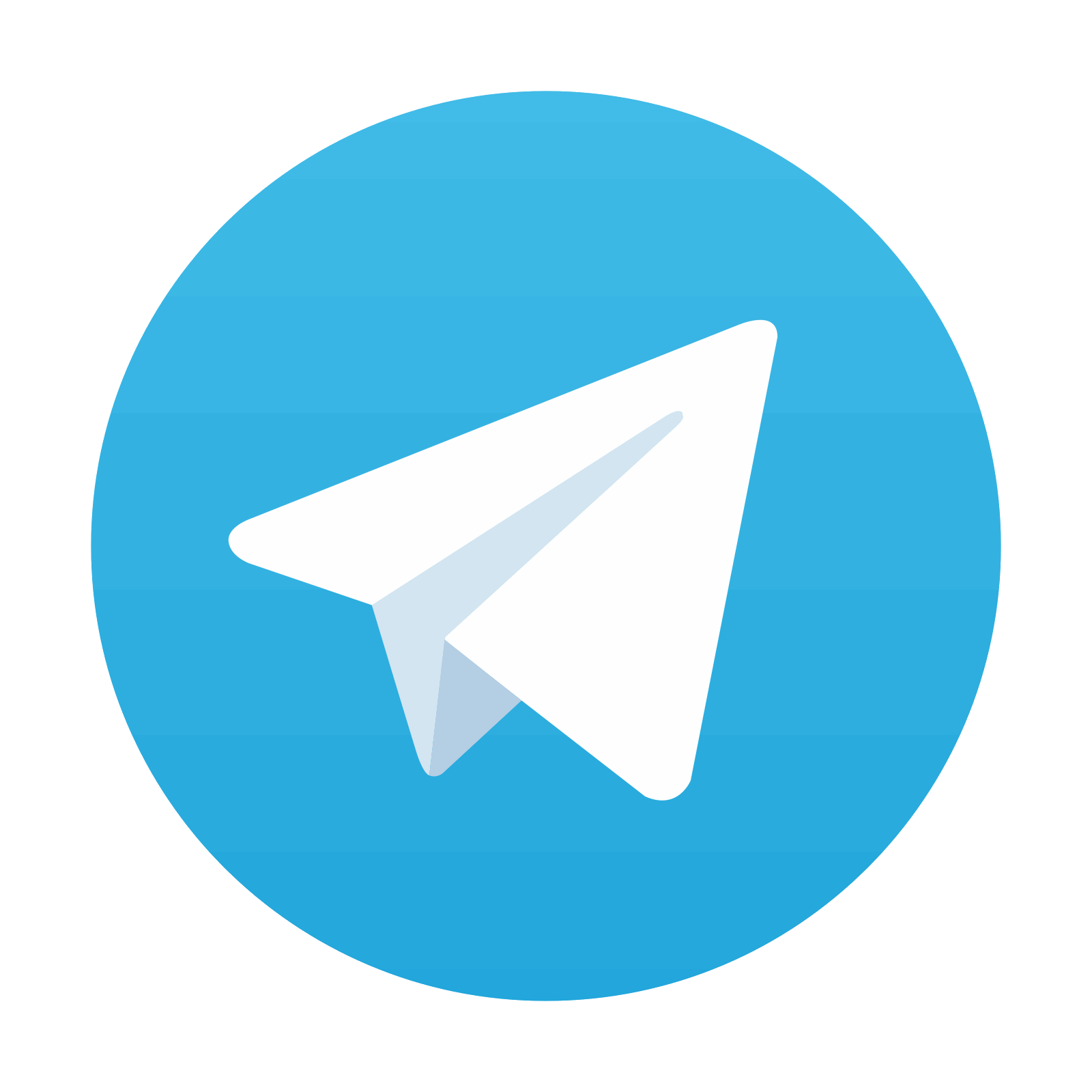
Stay updated, free articles. Join our Telegram channel

Full access? Get Clinical Tree
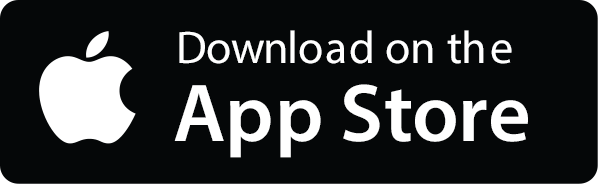
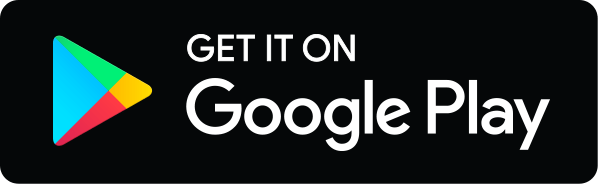